This article examines the effects of radiation on prosthetic breast reconstruction when human dermal allograft is used in the reconstruction. A brief review of radiation terminology and techniques as applied to the breast is given, followed by a review of the effects of radiation on wound healing in human tissue. The effects of radiation on prosthetic breast reconstruction before the advent of dermal allografting are reviewed. The addition of dermal allograft in reconstruction has led to a reduced number of complications. An algorithm for surgical treatment of irradiated prosthetic breast reconstructions is presented, with a discussion of the authors technique.
Roentgen discovered the x-ray in 1895. Within 1 year, Gocht in Germany treated the first 2 patients with x-ray radiation for painful locally advanced disease and obtained relief, demonstrating the potential benefits of radiation. Shortly thereafter, in 1901, Becquerel noted the deleterious effects of radiation when he reported that he developed a wound on his chest skin after leaving a vial of radium in his breast pocket for 6 hours. He noted the acute erythema and subsequent ulceration that took a prolonged time to heal. Thus, the double-edged sword that is radiation became known to physicians, and this therapeutic/wounding effect has remained a challenge to this day.
- 1.
External beam radiation has acute and chronic deleterious effects on wound healing in breast reconstruction, and thus the quality of the reconstruction itself.
- 2.
Complication rates of 30% to 60% were reported following radiation to an implant breast reconstruction before the use of acellular dermal matrices (ADM) materials.
- 3.
Complication rates for irradiated implant reconstructions have been lowered with the use of ADM materials, but the quality of the irradiated reconstruction is likely inferior to nonirradiated reconstruction.
- 4.
A staged approach to the irradiated implant reconstruction is advised, which involves placement of a tissue expander followed later by implant exchange.
- 5.
It is helpful, at the stage of initial tissue expander reconstruction, to:
- •
Secure the ADM material to the overlying breast skin flap with internal quilting sutures
- •
Secure the intramammary fold with permanent sutures
- •
- 6.
The irradiated breast implant reconstruction can be improved with additional pieces of ADM material, capsulectomy, and fat grafting at the time of expander removal/implant exchange. Fat grafting can be done at a later stage.
Ionizing radiation
Energy from radiation can be absorbed by biological material and can result in excitation of electrons in the tissue or ionization of the atoms within this tissue. Release of enough ionizing energy within the tissue can cause localized damage to that tissue. This damage, known to medicine for longer than a century, can be therapeutic or harmful depending on several factors.
Radiation can be classified as electromagnetic or particulate. Electromagnetic radiation such as the short-wavelength x-ray can cause damage to human tissue, as can the particulate radiation produced by discrete particles such as electrons and protons (among others). This particulate radiation can be delivered by a variety of techniques, by intracavitary means or via external beam. This article discusses the effects of external beam radiation therapy in common use today, but recognizes that newer techniques of intracavitary and partial breast irradiation are currently being evaluated.
External beam radiation
As would be expected, the higher the beam energy, the deeper the penetration into human tissue. Earlier beam energy devices were relatively lower-voltage x-ray machines that delivered low energy and were only able to penetrate superficially into tissue. These devices are termed orthovoltage, and are still useful for skin irradiation. Most breast tumors are now treated with high-energy megavoltage machines, commonly a linear accelerator, which can generate beams of sufficient energy to penetrate deeper into tissue.
The unit of measure of the biological effect in tissue caused by radiation is termed the radiation absorbed dose or rad. At present the gray (Gy) is used to represent 100 rad. Thus the more commonly used 50 Gy is equivalent to 5000 rad.
The degree of tissue penetration for a given radiation dose varies depending on the energy delivered. Fortunately, depth of penetration can be calculated very precisely for the different energy sources, and this depth of maximum dose (D max ) follows a predictable curve. Initially there is a zone of superficial penetration with a rapid buildup of energy, then a loss of energy as the beam penetrates deeper in the case of low-energy orthovoltage radiation. This process would yield the greatest effect in the skin, for example. By contrast, the high-energy megavoltage beam would have a slow buildup of energy superficially and reach its maximal energy deeper in the tissue, thus sparing the skin from the maximal radiation effect and damage while delivering the maximal effect and damage to tumors deeper in the tissue, such as the breast.
External beam radiation
As would be expected, the higher the beam energy, the deeper the penetration into human tissue. Earlier beam energy devices were relatively lower-voltage x-ray machines that delivered low energy and were only able to penetrate superficially into tissue. These devices are termed orthovoltage, and are still useful for skin irradiation. Most breast tumors are now treated with high-energy megavoltage machines, commonly a linear accelerator, which can generate beams of sufficient energy to penetrate deeper into tissue.
The unit of measure of the biological effect in tissue caused by radiation is termed the radiation absorbed dose or rad. At present the gray (Gy) is used to represent 100 rad. Thus the more commonly used 50 Gy is equivalent to 5000 rad.
The degree of tissue penetration for a given radiation dose varies depending on the energy delivered. Fortunately, depth of penetration can be calculated very precisely for the different energy sources, and this depth of maximum dose (D max ) follows a predictable curve. Initially there is a zone of superficial penetration with a rapid buildup of energy, then a loss of energy as the beam penetrates deeper in the case of low-energy orthovoltage radiation. This process would yield the greatest effect in the skin, for example. By contrast, the high-energy megavoltage beam would have a slow buildup of energy superficially and reach its maximal energy deeper in the tissue, thus sparing the skin from the maximal radiation effect and damage while delivering the maximal effect and damage to tumors deeper in the tissue, such as the breast.
Dosing schedule for radiation
The fact that certain cells are more sensitive to the effects of radiation than others was first noted in 1906. Since then it has become clear that cells are most sensitive to the effects of radiation during the G2-M phase of the cell cycle and are most resistant during the S phase. Thus, a single dose of radiation will not affect all the cells that are irradiated, because of differences in cell-cycle status. This situation has led to the concept of fractionation or delivery of radiation in installments over time, which is the current practice.
Radiation effects on skin and wound healing
As noted earlier, the effect of radiation on skin has been known for more than a century. Radiation has both acute and chronic effects that, of course, vary with the type, dose, and delivery schedule used.
The acute effects include erythema, dry desquamation at lower doses, and moist desquamation at higher doses. The erythema is the direct result of an inflammatory process caused by increased capillary permeability. Dry desquamation is the result of a dose of radiation strong enough to kill some epidermal cells, but allowing enough of the remaining epidermal cells to survive and proliferate. Moist desquamation occurs when an inadequate number of epidermal cells survive and the exposed dermis oozes serous fluid.
The chronic effects include either an increase or a decrease in pigmentation, thickening and fibrosis of the skin, telangiectasia, and alteration of hair, sweat, and sebaceous gland function. Pigmentation is altered by death of melanocytes or by deposition of pigment into the dermis. Dermal thickening can occur, as the collagen within the involved dermis will swell. If the dose is strong enough, fibroblasts are impaired and unable to synthesize collagen, and the dermis will atrophy and could ulcerate. Telangiectasia is the result of thrombosis of deeper vessels. Active hair follicles and sebaceous glands are killed by the radiation. This aspect is particularly important, because these structures provide migrating epidermal cells that are necessary for reepithelialization and wound repair.
As a direct result of these effects, a skin wound that is radiated is prone to prolonged healing, with a resultant scar that is thin in the dermis as well as the epidermis, has poor vascularity and strength, is susceptible to opening, and is unable to defend adequately against infection.
Effects of radiation on expanded skin in the animal model
Understanding the effects of radiation on skin, soft tissue, and wound healing, the question occurs as to what effects radiation may have on expander/implant (silicone or saline) breast reconstruction. A few studies have examined the effects of radiation on previously expanded skin in an animal model. Goodman and colleagues used New Zealand white rabbits and evaluated the effects of radiation on expanded skin 6 weeks after a single radiation dose of 25 to 35 Gy. A thickening of the epidermis but no change to the dermis was found, although the histology was performed only 6 weeks postradiation. Dvali and colleagues studied effects of radiation on Yorkshire pigs. Skin was irradiated in fractions up to a total dose of 48.6 Gy and expanders placed under this skin 3 months later, and the skin flaps were then expanded. The skin flaps were evaluated almost 6 months after completion of the radiation. At this time the irradiated skin flaps were reduced in overall area by 23% compared with nonirradiated controls. It was also found that radiation reduced expanded skin flap viability by almost one-third compared with controls. Performing a capsulectomy did not significantly worsen this decreased viability.
Effects of radiation on prosthetic breast reconstruction before the use of acellular dermal matrices
Much has been written on the effects of radiation on silicone and saline implant breast reconstruction. It is helpful to review the literature published before the introduction of acellular dermal matrices (ADM) in these reconstructions, to better assess the effects of the radiation with the subsequent use of these materials and to determine if there are any differences.
Given the proven negative effects of radiation on wound healing, it is not surprising that external beam radiation has been shown to exert a negative effect on the outcome of breast reconstruction using tissue expanders and saline or silicone implants.
Krueger and colleagues showed a 68% complication rate for tissue expander/implant reconstructions that were irradiated postoperatively compared with a 31% complication rate without radiation, resulting in a reconstructive failure rate of 37% compared with an 8% failure rate without radiation. Other reports show very high complication rates, high Baker III-IV capsule rates, and higher rates of reconstructive failure resulting in expander or implant loss when radiation follows tissue expander/implant reconstructions.
Similarly, Spear and Onyewu reported on a variety of tissue expander/implant reconstructions treated with and without radiation, and included reconstructions that had been irradiated before reconstruction, during expansion, and after reconstruction. A capsular contracture rate of 32.5% was found, regardless of when the patient received the radiation, compared with a rate of zero in the nonirradiated group. There was also a 37.5% incidence of adding a transverse rectus abdominis muscle (TRAM) or latissimus flap in the radiated group versus a 10% incidence in the nonirradiated patients to either salvage or improve the cosmesis of the reconstruction. Indeed, to combat these very high rates of complications, advanced capsule formation, and/or implant loss, Spear and Onyewu advise their patients that addition of a TRAM or latissimus flap at the time of expander removal/implant exchange will be needed in 50% of cases involving radiation.
Parsa and colleagues advise delaying reconstruction by at least 6 months after radiation to assess skin damage, and then to proceed with expander/implant reconstruction if there is no induration and moderate or better skin changes.
Kronowitz and colleagues propose a delayed-immediate reconstruction whereby an expander is placed immediately at the time of the mastectomy and, if radiation is needed postoperatively, the expander is left in place until completion of the radiation, before being replaced by an autologous flap.
Fine and Hirsch at Northwestern suggest a similar plan, but do not routinely place an autologous flap after radiation, and use an implant depending on desires of the patient.
Cordeiro and colleagues reported a totally different timetable whereby the tissue expander is placed immediately and inflated during subsequent chemotherapy, then replaced with an implant before initiation of radiation therapy. These investigators report very similar results between the irradiated and nonirradiated groups overall in terms of complications, with only a higher Baker III capsule rate among the irradiated reconstructions.
Despite these various protocols, tissue expansion followed by implant/exchange breast reconstruction continues to challenge us all as plastic surgeons, especially when the reconstruction has been or will be irradiated. The question is what effect, if any, has ADM had on these problems? To answer this question one needs to briefly examine the relatively short history of ADM and implant breast reconstruction, which is examined in greater detail in the article by Baxter elsewhere in this issue.
Brief review of ADM and implant reconstruction
Although the use of ADM in immediate breast reconstruction is relatively new, first reported in 2001, its use has dramatically increased in popularity since then. Many publications support its use, safety, and efficacy in immediate single-stage implant (silicone, saline, adjustable) reconstruction as well as in a 2-stage tissue expander/implant exchange technique. The use and history of these materials is well covered elsewhere in this issue and is not the focus of this article. Previous work has shown histologically that human dermal allograft promotes less fibrosis and inflammation in capsules formed around implants. The history and the expanding use of these materials is, however, important in evaluating these materials in the context of breast irradiation. As experience with these materials has increased, so have the applications that are generating more reports and evaluations of ADM materials subjected to radiation during the course of breast reconstruction.
Experimental evidence for protective effect of ADM in irradiated prosthetic reconstruction
In an important animal study, Komorowska-Timek and colleagues reported on the protective effect of AlloDerm ® (LifeCell Corp, Branchburg, NJ, USA), a popular human acellular dermal matrix, on irradiated implant capsules formed in Sprague-Dawley rats using small saline implants. The implants were inflated and irradiated 2 days later with a single dose of 21.5 Gy. The resultant capsules were examined at 3 weeks postradiation and a second group at 12 weeks postradiation, and compared with irradiated non-AlloDerm ® controls and nonirradiated controls. The AlloDerm ® group was seen to have a protective effect on the radiation changes that were seen. There was less radiation-related inflammation and, importantly, only 1 of the 20 rats that were radiated developed a pseudoepithelium on the AlloDerm ® , whereas all of the non-AlloDerm ® radiated control capsules developed this pseudoepithelium. These findings, which have been confirmed elsewhere, are very important because it is believed that formation of this pseudoepithelium is a precursor to the formation of a fibrotic capsule in humans.
Armed with the knowledge of the protective effects of ADM on irradiated implant capsules in the animal model and knowing that ADM has been shown to diminish inflammation and fibrosis in capsules formed around implants in humans, the question is whether ADM offers any protective benefit to an implant breast reconstruction that has been or will be radiated. An increasing body of literature has evaluated the results of implant breast reconstruction using ADM that have been radiated. Less has been written on the effects of radiation if the radiation has preceded the reconstruction.
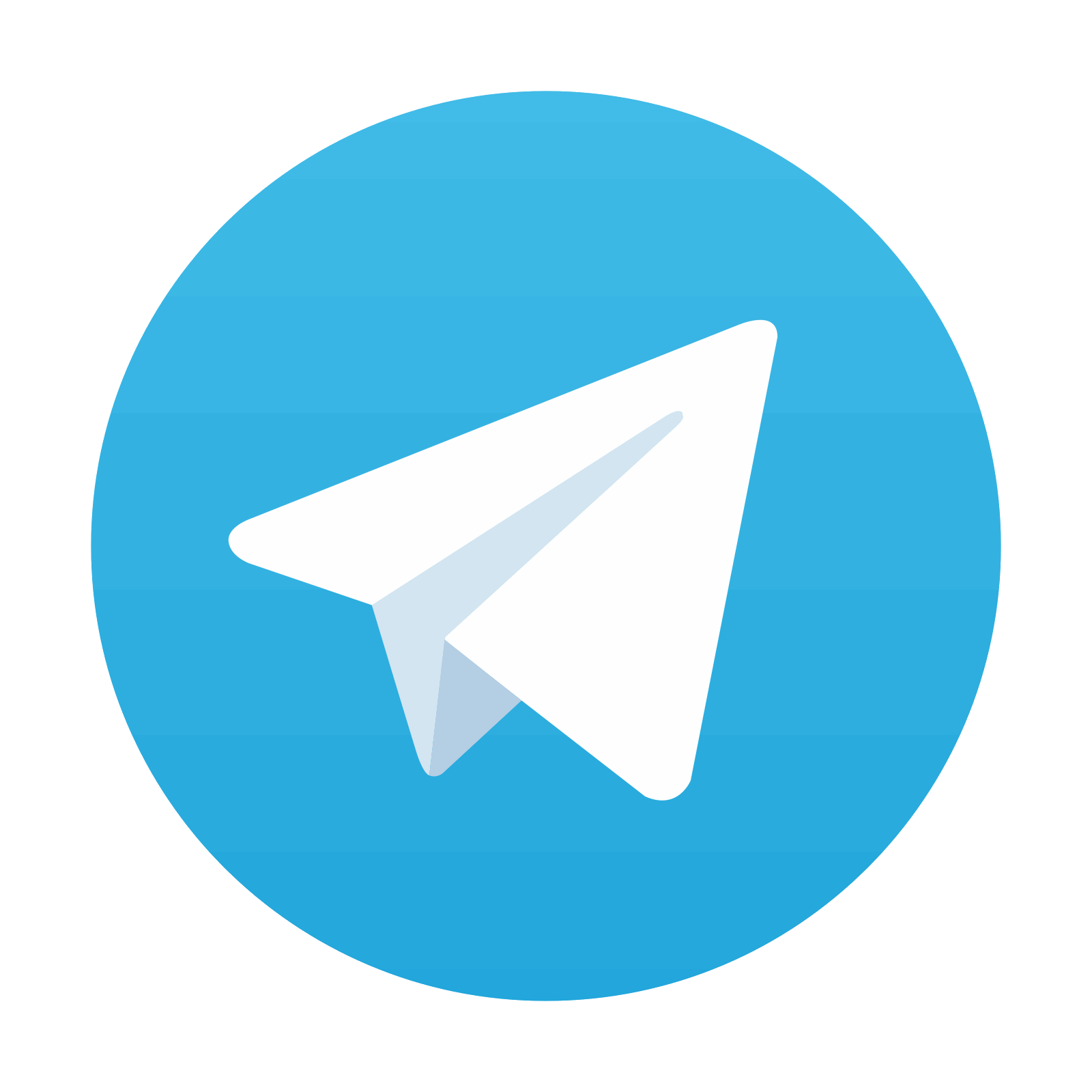
Stay updated, free articles. Join our Telegram channel

Full access? Get Clinical Tree
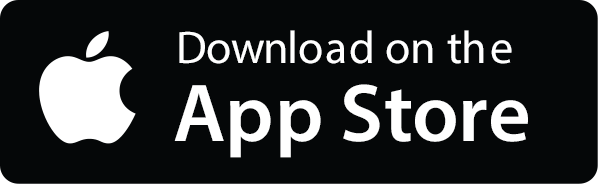
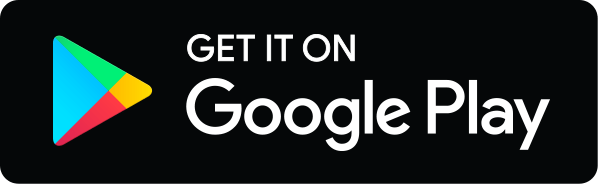