The Cellular and Molecular Basis of Scarring: The Paradigm of Hypertrophic Scarring After Thermal Injury
Edward E. Tredget
Jie Ding
KEY POINTS
Hypertrophic scar(s) (HTS) are a common dermal form of fibroproliferative disorder.
HTS commonly develop after thermal and other injuries to the deep layers of the dermis where prolonged inflammation occurs.
Although HTS have a low mortality, they can result in functional limitations and cosmetic and psychological difficulties for burn survivors.
Very few satisfactory treatment exists currently, which emphasizes the necessity of improving understanding of the mechanisms upon which novel therapy can be developed.
Clinical Significance of Fibroproliferative Disorders of the Skin
Fibroproliferative disorders (FPD) can involve many tissues throughout the body and constitute a leading cause of mortality in the United States, where they are involved as reported in 45% of annual deaths (see Chapter 3). Hypertrophic scars (HTS) and keloids are the dermal equivalent of FPD (Fig. 6-1).
HTS are a dermal form of FPD resulting from fibrotic wound healing after injuries to the deep dermis, because of burn injury, laceration, abrasions, surgery, and other trauma. HTS are red, raised, rigid and can cause pruritus, pain, and joint contractures. HTS formed in the facial area can cause cosmetic disfigurement, which predisposes to difficulties in psychological and social recovery after injury. HTS differ from keloids, which are characterized by frequent extension beyond the boundary of the original skin injury, occurrence even after very minor insults, and a higher incidence in darkly pigmented races. HTS occur commonly in all racial groups and are confined to the boundaries of the original wound.
HTS impose relatively low mortality but great morbidity, varying in incidence from 44% following surgical wounds to up to 91% following burn wounds, depending on the depth of injury.1,2,3 In the United States, 1.25 million people are treated for burns annually, 50,000 of them requiring hospitalization.4,5,6 In Canada, thermal injury necessitating hospitalization affects 26.4 patients per 100,000 people, amounting to greater than 7,000 significant thermal injuries annually; approximately 80% involve patients less than 45 years of age.7,8 Although the mortality rate for thermal injury has improved, burn patients experience a prolonged period of hospitalization (mean 26.2 days) and prolonged rehabilitation,9,10 requiring an average of 12.7 weeks off work for patients with thermal injuries greater than 30% of the total burn surface area (TBSA). Much of the rehabilitative phase is related to functional and cosmetic limitations imposed by HTS,10 including a reduction in range of motion of the extremities and the intense pruritus and heat intolerance making early return to work prohibitive6,7,10 (see Chapter 11).
Risk Factors for Hypertrophic Scars of the Skin
Risk factors such as young age, race/ethnicity, location of injury, and severity of injury (TBSA burn >20%) are well-recognized clinical features in the development of FPD of the skin.3,8,11 Prospective randomized studies have revealed that high body mass index, non-Caucasian ethnic background, and scar discomfort including pain and itch are positively associated with HTS, especially in individuals <50 years of age. The presence of hypertension is positively associated with the development of HTS,
and antihypertensive drugs and erythropoiesis-influencing agents appear to have antifibrotic affects.11 HTS develop with very high frequency after prolonged inflammation of slowly healing deep dermal wounds independent of other factors,12 with an incidence ranging up to 30% to 72% of burn patients as documented by Bombaro in injured military personnel.13,14 Unfortunately, HTS and keloids are known to respond poorly to current forms of therapy including pressure garments, topically applied silicone, and intralesional steroids (see Chapter 10). Improvements usually accrue slowly over months or years, and often incompletely.3,11,12,15,16,17,18 Genetic abnormalities associated with FPD, including single nucleotide polymorphisms (SNP), have been identified in some racial subpopulations such as the p27kip1 SNP in American Indian/Alaskan Natives.19
and antihypertensive drugs and erythropoiesis-influencing agents appear to have antifibrotic affects.11 HTS develop with very high frequency after prolonged inflammation of slowly healing deep dermal wounds independent of other factors,12 with an incidence ranging up to 30% to 72% of burn patients as documented by Bombaro in injured military personnel.13,14 Unfortunately, HTS and keloids are known to respond poorly to current forms of therapy including pressure garments, topically applied silicone, and intralesional steroids (see Chapter 10). Improvements usually accrue slowly over months or years, and often incompletely.3,11,12,15,16,17,18 Genetic abnormalities associated with FPD, including single nucleotide polymorphisms (SNP), have been identified in some racial subpopulations such as the p27kip1 SNP in American Indian/Alaskan Natives.19
![]() FIGURE 6-1 HTS in a 15-year-old boy 20 months after burn injury. (From Ladak A, Tredget EE. Pathophysiology and management of the burn scar. Clin Plast Surg. 2009;36(4):661-742.) |
As burn mortality rates improve, the resulting severe sequelae lead to prolonged periods of hospitalization and extensive rehabilitation, much of which is related to functional and psychological limitations imposed by HTS. A degree of remodeling of their HTS is a prerequisite for returning to their preinjury duties.20,21 Although most burn survivors recover, physical and psychiatric rehabilitation are significantly affected by the stigmatization, which occurs from their resulting scars22,23 (see Chapters 19 and 24).
Morphology and Composition of the Matrix in Hypertrophic Scars
Highlights:
HTS have thinner collagen fibrils with extensive regions of hyaline-appearing proteoglycans and glycoproteins in the interfibrillar spaces.
Ultrastructural nodules or whorls are present in HTS.
HTS contain higher proportions of types III and V collagens and low amounts of the small leucine-rich proteoglycan decorin, as compared with normal skin.
Collagen is the predominant extracellular matrix (ECM) protein in normal skin and HTS, where it functions to provide tensile strength of the tissue. However, collagen represents only about 30% of the dry weight of HTS because of other components including proteoglycans and glycoproteins, such as fibronectin and tenascin.24 The major genetic form of collagen in skin is type I, assembled into thick fibrils, fibers, and fiber bundles. In HTS, thinner collagen fibrils averaging around 60 nm are present compared with 100 nm in normal dermis. This is in part because of higher proportions of thinner types III and V collagens in HTS, as much as 33% and 10%, respectively.25 In light microscopy,
much of the collagen in HTS is in whorls or nodules rather than thick fibers or fiber bundles that are normally oriented parallel to the surface in unaffected skin26 (see Chapter 5). Extensive regions of hyaline-appearing material exist in HTS with little organization of the fine collagen fibrils, leading to an ovoid or irregular cross-section on electron microscopy. The interfibrillar space is occupied by proteoglycans and glycoproteins, which normally function to provide turgor, resilience, and resistance to compression as well as influencing cell adhesion and other functions, partly by modifying growth factor activity. Proteoglycans, which account for the water holding capacity of tissues, consist of one or more glycosaminoglycan chains as linear anionic polymers of disaccharides covalently attached to a protein core.27 In HTS there is a 2.4-fold increase in glycosaminoglycan content and they are hyperhydrated relative to normal dermis and mature scars, accounting for the increased turgor in HTS.24,27
much of the collagen in HTS is in whorls or nodules rather than thick fibers or fiber bundles that are normally oriented parallel to the surface in unaffected skin26 (see Chapter 5). Extensive regions of hyaline-appearing material exist in HTS with little organization of the fine collagen fibrils, leading to an ovoid or irregular cross-section on electron microscopy. The interfibrillar space is occupied by proteoglycans and glycoproteins, which normally function to provide turgor, resilience, and resistance to compression as well as influencing cell adhesion and other functions, partly by modifying growth factor activity. Proteoglycans, which account for the water holding capacity of tissues, consist of one or more glycosaminoglycan chains as linear anionic polymers of disaccharides covalently attached to a protein core.27 In HTS there is a 2.4-fold increase in glycosaminoglycan content and they are hyperhydrated relative to normal dermis and mature scars, accounting for the increased turgor in HTS.24,27
HTS contain only 2% of the normal amount of a small dermatan sulfate proteoglycan, decorin, which is the major proteoglycan found in normal dermis. Instead, there is a sixfold higher concentration of a large proteoglycan versican, which carries 12 to 30 chrondroitin sulfate chains. It is found normally in hyaline cartilage, and in small amounts in proliferating regions of the epidermis and associated with elastin in the dermis. Decorin regulates collagen fibril formation; decorin knockout animals display collagen fibrils that are variable in diameter and irregular in outline,28 similar to that seen in HTS.27
As scars mature spontaneously, collagen fibers become coarser and better organized and there is a return of decorin (detected by immunohistochemistry) associated with a large increase in the number of decorin expressing cells.27,29 Mature scars ultimately show contents of collagen, proteoglycans, and water that are indistinguishable from those in normal dermis.24
Table 6-1 Features of Normal, HTS, and Deep Dermal Fibroblasts | ||||||||||||||||||||||||||||||||||||||||||||||||||||||||||||||||||||
---|---|---|---|---|---|---|---|---|---|---|---|---|---|---|---|---|---|---|---|---|---|---|---|---|---|---|---|---|---|---|---|---|---|---|---|---|---|---|---|---|---|---|---|---|---|---|---|---|---|---|---|---|---|---|---|---|---|---|---|---|---|---|---|---|---|---|---|---|
|
The Cellular Basis of Hypertrophic Scarring
Fibroblasts and Myofibroblasts
Highlights:
Dermal fibroblasts are the predominant cell type in the dermis.
HTS fibroblasts have many unique properties different from normal skin fibroblasts.
Myofibroblasts are a contractile phenotype of fibroblasts.
Normal fibroblasts and HTS fibroblasts have been found to have significantly different features in vitro (Table 6-1). Many HTS strains synthesize fibronectin, pro-α2[I] collagen mRNA and protein, and transforming growth factor beta (TGF-β)30 at higher levels than normal dermal fibroblasts. All strains of HTS fibroblasts consistently demonstrate reduced collagenase (matrix metalloproteinase—MMP-1),31 nitric oxide,32 and decorin production.33
Increased numbers of myofibroblasts constitute a prominent component of the hypercellular matrix in HTS; they contain microfilament bundles and alpha smooth muscle actin (α-SMA) important for wound contraction, a significant comorbid complication of HTS and other FPD.34,35 The development of myofibroblasts appears to be induced by TGF-β33,36 and strongly correlates with the severity of burn injury (TBSA). Myofibroblasts appear to differentiate not only from regional fibroblasts in the wounds under the influence of TGF-β,36 but also from bone marrow-derived blood-borne sources.37,38,39 Different from fibroblasts, myofibroblasts show highly modulated responses to TGF-β1 and interferon (IFN)-γ during wound healing, and in collagen synthesis and contractile capacity.36 Myofibroblast formation can be induced by the application of tension in the
absence of wounding38 (see Chapter 7). As granulation tissue is converted to HTS and to mature scar tissue, a reduction in cellularity occurs through the induction of apoptosis, a process that is abrogated in myofibroblasts present in HTS by the expression of α-SMA in stress fibers.39,40
absence of wounding38 (see Chapter 7). As granulation tissue is converted to HTS and to mature scar tissue, a reduction in cellularity occurs through the induction of apoptosis, a process that is abrogated in myofibroblasts present in HTS by the expression of α-SMA in stress fibers.39,40
Fibroblast Heterogeneity and the Profibrotic Microenvironment
Highlights:
Sorrell and Caplan have found that normal adult human skin contains at least three separate subpopulations of fibroblasts; these occupy unique niches depending on the depth in the dermis and exhibit distinctive differences when isolated by limited dilution cloning.42,43 Fibroblasts associated with hair follicles show distinctive characteristics from cells in the papillary and reticular dermis.44,45 Papillary dermal fibroblasts, which reside in the superficial dermis, are heterogeneous in terms of morphology and proliferation kinetics; reticular fibroblasts in the deep dermis possess myofibroblast-like characteristics associated with greater collagen lattice contraction and α-SMA expression.46,47,48,49
Fibroblasts that arise from the deeper layers proliferate at a slower rate,48 are significantly larger morphologically,49 and MMP-1 mRNA is significantly lower. Fibroblasts from the deeper layers produce more TGF-β, connective tissue growth factor (CTGF), and heat shock protein 47 (HSP47), a human chaperone protein for type I collagen. Fibroblasts from the deeper layer also produce more α-SMA protein and contract collagen gels more efficiently.49 They also produce more collagen, more of the fibrocartilaginous proteoglycan versican, and less decorin.48,49 As discussed earlier, decorin and other members of the small leucine-rich repeated protein (SLRP) family, fibromodulin and lumican, function to bind type I collagen in the ECM and regulate the kinetics of collagen fibrillogenesis and the diameter and distance between fibrils.49 Decorin and fibromodulin can also bind to and inhibit TGF-β1 activity in vitro50 and in vivo.51,52 Low levels of growth factor production by fetal cells, especially TGF-β1, is a major factor in the absence of excess collagen deposition and scar formation51,52 (see Chapter 27). In contrast, overexpression of TGF-β1 results in marked lung fibrosis, which is significantly reduced by concomitant increased expression of decorin.52 Fibroblasts isolated from the deep dermis produce less decorin and more large cartilage-like proteoglycans, including versican and aggrecan, which can account for the ultrastructural abnormalities in HTS. Recently fibrocytes, a bone marrow-derived circulating monocyte, have also been described to produce less SLRPs and more versican, hyaluronan, perlecan, and biglycan in the ECM.46,53 The profibrotic characteristics of deep dermal fibroblasts can be upregulated by bone marrow-derived mesenchymal stem cells.54
The Role of Toll-Like Receptor Signaling in Fibrosis
Highlights:
Toll-like receptors (TLRs) are present on normal and HTS fibroblasts.
They appear to mediate inflammation and the activation of dermal fibroblasts in HTS, and antagonism of this pathway may lead to novel therapeutic options.
TLRs are a group of highly conserved molecules that allow the innate immune system to sense molecules commonly present in bacteria and viruses, termed pathogen-associated molecular patterns (PAMPs), or endogenous molecules that are released from necrotic tissue, termed damage-associated molecular patterns (DAMPs).55 They function as activators of the innate immune system, but have increasingly been implicated in the switch from normal wound healing responses to fibrosis in many different organs and tissues.55,56 Ten different members that bind specific ligands exist; TLR2 recognizes gram-positive bacteria and TLR4 senses gram-negative bacteria by binding lipopolysaccharide (LPS).57 The mechanism of fibrosis has not been established in the skin and many other tissues. However, in the liver TLR4-dependent fibrosis is stimulated by LPS directly, targeting fibroblast precursors which release chemokines to activate macrophage-like Kupffer cells. This results in unrestricted TGF-β-mediated activation of hepatic stellate cells, increased deposition of ECM, and the promotion of liver fibrosis.58,59,60,61,62 TLRs are not just expressed in immune cells and monocytes, but are found in a range of tissues such as cardiac myocytes, vascular cells, cortical tubule cells, mesangial cells, podocytes, and dermal fibroblasts, which may contribute to the inflammation and resulting fibrosis of heart, kidney, lung, and skin.63,64,65,66,67,68,69
Recently, aberrant TLR activation by endogenous molecules released by necrotic or activated cells and ECM molecules upregulated upon injury or degraded following tissue damage (DAMPs) have been implicated in a number of diseases where inappropriate, pathogenic inflammation is at the basis of the fibrosis.70,71 One such DAMP is the matrix proteoglycan biglycan, which has been found to be highly expressed in human HTS tissue and HTS fibroblasts in vitro; deep dermal fibroblasts have been shown to produce more biglycan (Table 6-1).33,53 Although fibroblasts play an important structural role in wound healing, emerging evidence suggests that fibroblasts modify the healing microenvironment by inducing inflammation through activation of the TLRs and signaling through nuclear factor
kappa B (NF-κB); this can lead to both the recruitment of monocytes and immune cells and subsequent production of inflammatory cytokines.72,73
kappa B (NF-κB); this can lead to both the recruitment of monocytes and immune cells and subsequent production of inflammatory cytokines.72,73
Thus, fibroblasts appear capable of stimulating inflammation via TLR activation, likely via NF-κB and mitogen-activated protein kinase (MAPK), which upregulates the infiltration of inflammatory cells. In addition, activated deep dermal fibroblasts appear very important in the development of severe FDP of the skin. Future investigation of these profibrotic cells may improve the understanding of the role of TLRs in fibrosis and lead to novel therapeutic options to antagonize abnormal activation of fibroblasts by inflammation.
The Role of Blood-Borne Cells in Wound Healing and Fibrosis
Fibrocytes
Highlights:
Circulating bone marrow-derived fibrocytes are recruited to the wound site after injury.
They contribute to wound healing and fibrosis by producing ECM, secreting cytokines including TGF-β, and presenting antigen to T cells.
They regulate fibroblasts and promote myofibroblast differentiation (Fig. 6-3).
Previously, Bucala has identified an adherent and proliferating population of cells with a fibroblast-like morphology that expresses a variety of biomarkers including some hematopoietic cell markers, mesenchymal cell markers, chemokine receptors, antigen-presenting cell markers, and others.74,75,76,77 They make up 0.5% of peripheral blood leukocytes, but can constitute 10% of cells infiltrating acute wounds.76 The chemokines CCL12 and CXCL12 and secondary lymphoid chemokines and their receptors CCR2, CXCR4, and CCR7 are involved in fibrocyte migration in vivo.78 These migrating fibrocytes are capable of synthesizing ECM proteins, proteases including collagenase, and growth factors such as TGF-β1, tumor necrosis factor alpha (TNF-α), interleukin (IL)-6 and IL-10, but can also present antigens and thereby prime naïve T lymphocytes.79,80 It has been demonstrated that fibrocytes differentiate into fibroblasts and myofibroblasts via in vivo and in vitro experiments.81,82
Fibrocytes have been identified in burn patients from the peripheral blood mononuclear cells (PBMCs), where the percentage of type I collagen-positive fibrocytes was significantly higher (up to 10% of PBMCs) than for control individuals (normal level <0.5%) and correlated with serum levels of TGF-β.83,84 In vitro, fibrocytes can be cultured from CD14+ PBMCs, but required TGF-β in the conditioned media from CD14– PBMCs for differentiation.85 Leukocyte-specific protein 1 (LSP-1) is a unique marker for fibrocytes and is upregulated in burn patients, remaining stable through differentiation.84,85 Double staining with antibodies to LSP-1 and the C-propeptide of type 1 collagen (COL-I) has identified a 300% increase in fibrocytes in HTS tissue, located primarily in deeper layers of the papillary dermis (Fig. 6-4).84 Characteristic morphologic alterations in fibrocytes occur in vitro after exposure to endotoxin, which are corrected by treatment with IFN-α2b.85,86,87
From serial analysis of burn patients with HTS, increased numbers of fibrocytes are present in HTS tissues compared to mature scars and normal skin.86,87,88 Quantitatively, fibrocytes produce less collagen than HTS fibroblasts; however, fibrocytes from burn patients differ from that of normal individuals because of their paracrine effects that include stimulating dermal fibroblasts to proliferate, production and contraction of the ECM, and producing TGF-β and its downstream effector, CTGF.87,88 These findings resemble others,89,90,91,92 where the principal source of collagen in other fibrosis models appears to be local fibroblasts. However, bone marrow-derived immune cells resembling fibrocytes
persist in the matrix, suggesting an important paracrine role of fibrocytes in HTS and other FPD. It is possible to antagonize many of these fibrogenic effects of fibrocytes in vitro with IFN-α; significantly decreased numbers of fibrocytes were also found in the tissues of burn patients in response to systemic IFN treatment in vivo and were associated with a concomitant resolution of fibrosis and scar remodeling.88 In addition, increased angiogenesis associated with increased vascular endothelial growth factor (VEGF) in HTS is reduced by IFN-α, in part because of suppression of endothelial cell proliferation and tubule formation through reduction in VEGF receptor expression in endothelial cells.87 Coexpression of VEGF mRNA with the stromal cell-derived factor 1 (SDF-1) mRNA further implicates fibrocytes in the pathophysiology of idiopathic pulmonary fibrosis and other fibroses.92
persist in the matrix, suggesting an important paracrine role of fibrocytes in HTS and other FPD. It is possible to antagonize many of these fibrogenic effects of fibrocytes in vitro with IFN-α; significantly decreased numbers of fibrocytes were also found in the tissues of burn patients in response to systemic IFN treatment in vivo and were associated with a concomitant resolution of fibrosis and scar remodeling.88 In addition, increased angiogenesis associated with increased vascular endothelial growth factor (VEGF) in HTS is reduced by IFN-α, in part because of suppression of endothelial cell proliferation and tubule formation through reduction in VEGF receptor expression in endothelial cells.87 Coexpression of VEGF mRNA with the stromal cell-derived factor 1 (SDF-1) mRNA further implicates fibrocytes in the pathophysiology of idiopathic pulmonary fibrosis and other fibroses.92
Heterotopic ossification (HO) is a clinical condition where mature lamellar bone is formed in nondamaged tissues such as muscle, tendon, and fascia, particularly after burns and traumatic injuries.93,94 HO can lead to skin breakdown, significant soft tissue deformity, joint ankylosis, and chronic pain that can prolong rehabilitation. In burn patients, the incidence of HO varies between 0.2% and 4%,93 and is more frequent in patients with extensive burns (>20% TBSA). Although HO may occur in joints unrelated to burn injuries, lesions may develop under areas of deep burns complicated by HTS, especially in the elbow,95,96 and it is associated with prolonged loss of consciousness, mechanical ventilation, long-term immobilization, burn wound infection and/or delayed closure, loss of skin grafts, and recurring local trauma including passive range of motion.95,96,97,98 Therapeutic strategies for the prevention of HO are of limited success and include local radiation, but concerns of long-term side effects of radiation, including the development of secondary malignancies, stress the need for better animal models to develop and adequately test novel therapies before application to patients.99
Recently, a large population of fibrocytes (LSP-1+ COL-I+) have been identified within HO specimens as distinctive blood-borne cells that traffic to injured and apparently noninjured tissues and interact with resident cells.100 Fibrocytes have the potential to differentiate into osteoblasts and chondrocytes101 and can be reprogrammed into antifibrotic cells stimulating MMP-1 production in dermal fibroblasts, collagen breakdown, and scar remodeling.102 Thus, HO and FPD such as HTS have common features and appear to be causally related. In this concept, after significant initial local tissue injury generates a systemic inflammatory response, unique PBMCs including fibrocytes contribute to the development of fibrosis and osteogenic matrix in injured and noninjured tissues in as yet unidentified mechanisms.
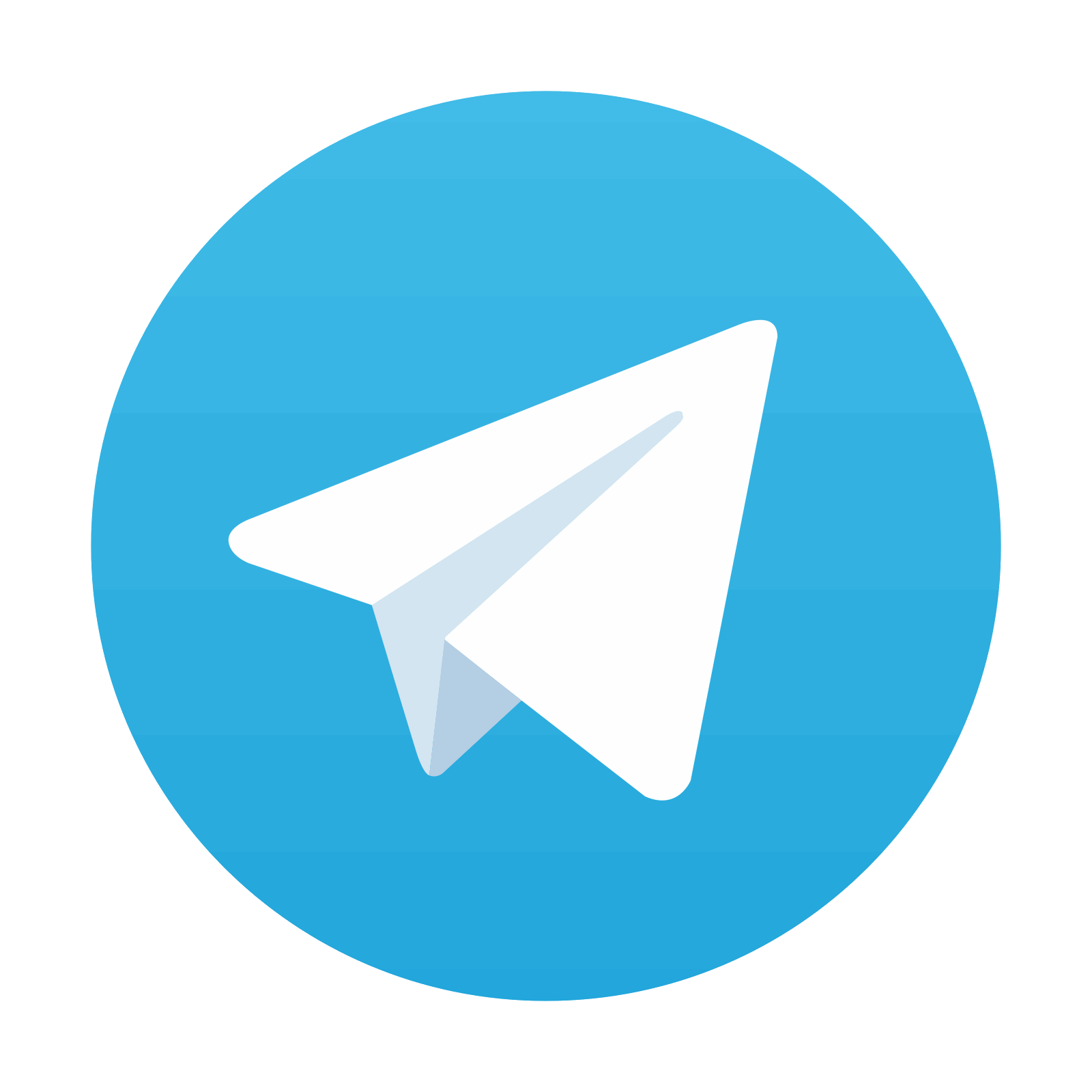
Stay updated, free articles. Join our Telegram channel

Full access? Get Clinical Tree
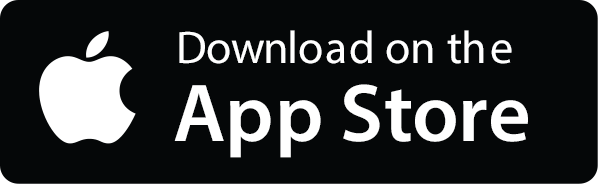
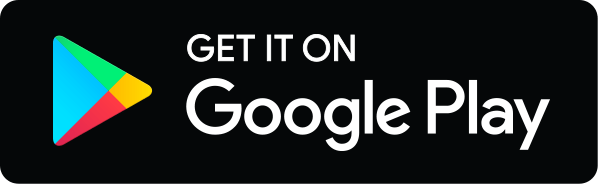