The Biomechanics of Scar Formation
Dominik Duscher
Michael T. Longaker
Geoffrey C. Gurtner
KEY POINTS
All phases of wound healing are influenced by mechanical forces, and mechanotransduction, the mechanisms by which mechanical force is converted to biochemical stimuli, plays a pivotal role in cutaneous fibrosis.
Extracellular biomechanical cues are transduced by the extracellular matrix (ECM), a dynamic structure with multiple functions. Mechanical stimuli can expose hidden domains and alter spatial concentration of growth factors within the ECM, resulting in changes of cellular behavior and phenotype. Additionally, stored factors within the ECM can be released based on the effects of mechanical force.
Intracellular mechanotransduction, the mechanisms by which cells “feel” and interact with their environment, is mediated by mechanoresponsive ion channels (e.g., Ca2+), growth factor, and cytokine receptors (e.g., for transforming growth factor (TGF)-β or stromal cell-derived factor (SDF)-1), integrin-matrix interactions (e.g., involving focal adhesion kinase (FAK)) and G protein-coupled receptors (GPCRs).
Mechanomodulation can be utilized therapeutically. Randomized controlled clinical trials have demonstrated that mechanomodulation of the wound environment using an elastomeric silicone dressing significantly reduces scar development.
Scar formation is among the most complex biologic processes and represents a substantial source of morbidity worldwide. In humans, scarring is the typical response to tissue injuries. The process of fibrotic repair, prioritizing early restoration of tissue integrity rather than functional regeneration, offers a survival advantage and is therefore highly conserved in evolution.1,2 Despite extensive research efforts dedicated to the expansion of our understanding of the mechanisms underlying scar formation, effective clinical therapies for scar mitigation are only beginning to be developed. A detailed understanding of the numerous signaling pathways involved is essential to develop remedies for fibrosis and scarring. During initial research efforts concentrated on elaborating the biochemical mechanisms involved in scar formation, however, evidence has emerged that mechanical forces play a previously underestimated role in the modulation of these pathways. The impact of mechanical forces on cutaneous scarring was first observed as early as the 19th century,3 but only recently have the underlying signaling mechanisms begun to be elucidated. Mechanotransduction, which refers to the mechanisms by which mechanical forces are converted to biochemical stimuli, has been closely linked to inflammation and is believed to play a pivotal role in cutaneous fibrosis.4 There is increasing evidence that all phases of wound healing are influenced by mechanical forces,5 but the field of wound mechanobiology is still in its infancy. However, utilizing the recent insights into how mechanotransduction of environmental cues effects the behavior of cells and tissues will help us to formulate effective therapeutics and may lead to the achievement of the ultimate goal, to transform fibrotic healing into tissue regeneration.
Molecular Biomechanics of Scar Formation
The field of mechanobiology continues to advance rapidly. The application of innovative in vitro and in vivo models leads to a more thorough understanding of the effects of mechanical forces on biologic processes.6 It has been demonstrated that cells are able to convert mechanical stimuli into biochemical or transcriptional changes via the process of mechanotransduction.7 Signal transduction from the microenvironment involves numerous proteins and molecules of the extracellular matrix (ECM), the cytoplasmic membrane, the cytoskeleton, and the nuclear membrane, which transport mechanical cues down to the nuclear chromatin to alter cellular programs at the genetic and epigenetic level.8
Several attempts to define the role of mechanical influences in molecular biology have been made. The most widely accepted system linking the different levels of mechanotransduction is known as tensional integrity or “tensegrity.”9 First described as an architectural concept,10
this principle, based on isolated components in compression inside a net of continuous tension, was adapted and developed by Ingber et al.9 to explain how cellular structures and processes are influenced by mechanical force. However, a complete understanding of the complex mechanotransduction pathways in living organisms remains elusive. Nevertheless, the observations made in small and large animal studies implicate a significant involvement of mechanical influences in the development of cutaneous scarring. Translating these findings into clinical therapies must be our principal goal.
this principle, based on isolated components in compression inside a net of continuous tension, was adapted and developed by Ingber et al.9 to explain how cellular structures and processes are influenced by mechanical force. However, a complete understanding of the complex mechanotransduction pathways in living organisms remains elusive. Nevertheless, the observations made in small and large animal studies implicate a significant involvement of mechanical influences in the development of cutaneous scarring. Translating these findings into clinical therapies must be our principal goal.
Extracellular Mechanotransduction
The ECM is much more than just an inert three-dimensional network passively offering structural support. It is a dynamic and living tissue responsible for numerous functions. The ECM governs cell adhesion, migration, differentiation, proliferation, and apoptosis and is highly involved in the complex processes of mechanotransduction11,12 (Fig 7-1). Mechanical cues transported through the ECM to cells can directly affect gene expression because of a direct link between structural proteins of the ECM to nuclear chromatin.13
Additional evidence supporting the theories how ECM can alter cell functionality and phenotype is provided by the fact that tissue stiffness and rigidity can be linked to tumor growth and malignancy.14 The extracellular environment can function in both ways, being pro-oncogenic in some circumstances,15 but also may reverse malignant behavior if corrected.16 Similarly, microenvironmental signals can influence scarring and fibrosis.17,18,19 It has been demonstrated that scar progression results from a positive feedback loop connecting the accumulation of ECM and increased matrix stiffness to the enhancement of fibroblast proliferation and collagen production via mechanoresponsive mechanisms.20,21
In addition to direct effects on cell behavior via ECM-cell membrane/cytoskeletal interfaces, mechanical cues can also execute indirect effects. Specifically, alterations in ECM structure can expose normally hidden domains and binding sites that have regulatory capabilities.22 The ECM can also alter the spatiotemporal composition of the microenvironment by changing the concentrations of soluble and matrix-bound effector molecules and growth factors, such as transforming growth factor beta (TGF-β), resulting in considerable impact on biologic functions.23
Intracellular Mechanotransduction
The mechanisms by which cells “feel” and interact with their environment are incompletely understood. However, recent efforts have led to the identification of key signaling pathways involved in intracellular mechanotransduction. The central mediators of mechanotransduction include mechanoresponsive ion channels (e.g., Ca2+), growth factor and cytokine receptors (e.g., TGF-β and stromal cell-derived factor 1 [SDF-1]), integrin-matrix interactions, and G protein-coupled receptors (GPCRs)24,25 (Fig. 7-2).
The fact that conformational alterations of ion channels govern numerous cellular functions is an established biologic concept. However, an understanding of how mechanoresponsive calcium channels influence fibrotic pathways has only recently begun to emerge.26 Specifically, calcium-dependent ion channels have been demonstrated to be heavily involved in the arrangement of elements of the cytoskeleton, which are associated with mechanotransduction.27 Additionally, Ca2+ influx caused by mechanical stimulation of the cell membrane activates mitogen-activated kinases (MAPKs), which enhance profibrotic gene expression.28
Growth factors and cytokines together with their receptors are implicated in all stages of wound healing and the development of cutaneous scarring. Recent work has shown
promising results regarding scar formation and appearance when the cytokine system is manipulated.29 Specifically, modulating the ratios of subsets of TGF-β in the wound microenvironment has received significant attention (see below). Mechanical stimuli result in a release of TGF-β from its reservoir in the extracellular latent complexes.30,31,32 This growth factor is associated with numerous fibrotic diseases, and acts mainly via the TGF-β receptor 2 and its downstream effector proteins SMAD 2 and 3. The TGF-β signaling cascade controls numerous profibrotic mechanisms, such as collagen production and fibroblast to myofibroblast differentiation.33,34 Another mechanoresponsive signaling molecule responsible for the regulation of cutaneous healing and fibrosis is SDF-1 or CXCL12. It has been demonstrated that mechanical stretching can upregulate SDF-1a in skin, which directly leads to the recruitment of circulating proregenerative mesenchymal stem cells through the SDF-1a/CXCR4 axis.35 However, somewhat contradictory results regarding the influence of SDF-1 on wound healing and scar formation have been reported. Whereas hypertrophic burn scars could be associated with increased SDF-1a/CXCR4 signaling,36 it could also be demonstrated that the therapeutic application of SDF-1 to cutaneous wounds of mice and pigs leads to enhanced healing with decreased fibrosis.37,38 Additionally, a local increase of SDF-1 has been identified as the potential underlying mechanism of noncontact, low-frequency ultrasound therapy, which has been shown to have beneficial effects in the treatment of chronic wounds.39 This further corroborates the pivotal role of cytokines at the intersection of mechanotransduction and tissue healing, and strongly merits further investigation.
promising results regarding scar formation and appearance when the cytokine system is manipulated.29 Specifically, modulating the ratios of subsets of TGF-β in the wound microenvironment has received significant attention (see below). Mechanical stimuli result in a release of TGF-β from its reservoir in the extracellular latent complexes.30,31,32 This growth factor is associated with numerous fibrotic diseases, and acts mainly via the TGF-β receptor 2 and its downstream effector proteins SMAD 2 and 3. The TGF-β signaling cascade controls numerous profibrotic mechanisms, such as collagen production and fibroblast to myofibroblast differentiation.33,34 Another mechanoresponsive signaling molecule responsible for the regulation of cutaneous healing and fibrosis is SDF-1 or CXCL12. It has been demonstrated that mechanical stretching can upregulate SDF-1a in skin, which directly leads to the recruitment of circulating proregenerative mesenchymal stem cells through the SDF-1a/CXCR4 axis.35 However, somewhat contradictory results regarding the influence of SDF-1 on wound healing and scar formation have been reported. Whereas hypertrophic burn scars could be associated with increased SDF-1a/CXCR4 signaling,36 it could also be demonstrated that the therapeutic application of SDF-1 to cutaneous wounds of mice and pigs leads to enhanced healing with decreased fibrosis.37,38 Additionally, a local increase of SDF-1 has been identified as the potential underlying mechanism of noncontact, low-frequency ultrasound therapy, which has been shown to have beneficial effects in the treatment of chronic wounds.39 This further corroborates the pivotal role of cytokines at the intersection of mechanotransduction and tissue healing, and strongly merits further investigation.
Although ion channels and cytokines certainly play an important role in the mechanobiology of scar formation, the most extensively studied cellular elements in this context are integrins. The members of this family of heterodimeric transmembrane receptor proteins possess a cytoplasmic domain, which communicates with the actin cytoskeleton, and extracellular domain binding molecules of the ECM.40 Integrins carry out both “outside-in” communication of environmental mechanical cues to the cell and “inside-out” interactions via forces produced by the cytoskeleton.40 This two-way signaling process is influenced by intracellular proteins, which bind to integrins to form large macromolecular structures, the so-called focal adhesions.41,42 The most prominent of these intracellular binding proteins is the focal adhesion kinase (FAK). Conformational changes in the complex macrostructure of the focal adhesions caused by mechanical stimuli lead to an activation of the nonreceptor protein tyrosine kinase FAK via autophosphorylation. Although integrins have no intrinsic enzymatic activity, they can affect downstream signaling pathways via FAK. Specifically, FAK signaling has been heavily linked to wound healing aberrations.4,43 Although mechanical stimuli influence all cell types involved in wound healing,44 the effects of FAK activation demonstrate how mechanical cues can change biologic mechanisms in different cell types in diametrically opposed directions. Our
laboratory has previously demonstrated that FAK is a key regulator of mechanosensing in cutaneous fibroblasts and that a fibroblast-specific deletion of FAK leads to reduced fibrosis after injury in a mouse model of scar formation.4 In stark contrast, the loss of FAK in cutaneous keratinocytes leads to a significant delay in wound healing and dermal proteolysis in mice,43 suggesting a skin layer-specific effect of FAK signaling with a complex influence on ECM repair.
laboratory has previously demonstrated that FAK is a key regulator of mechanosensing in cutaneous fibroblasts and that a fibroblast-specific deletion of FAK leads to reduced fibrosis after injury in a mouse model of scar formation.4 In stark contrast, the loss of FAK in cutaneous keratinocytes leads to a significant delay in wound healing and dermal proteolysis in mice,43 suggesting a skin layer-specific effect of FAK signaling with a complex influence on ECM repair.
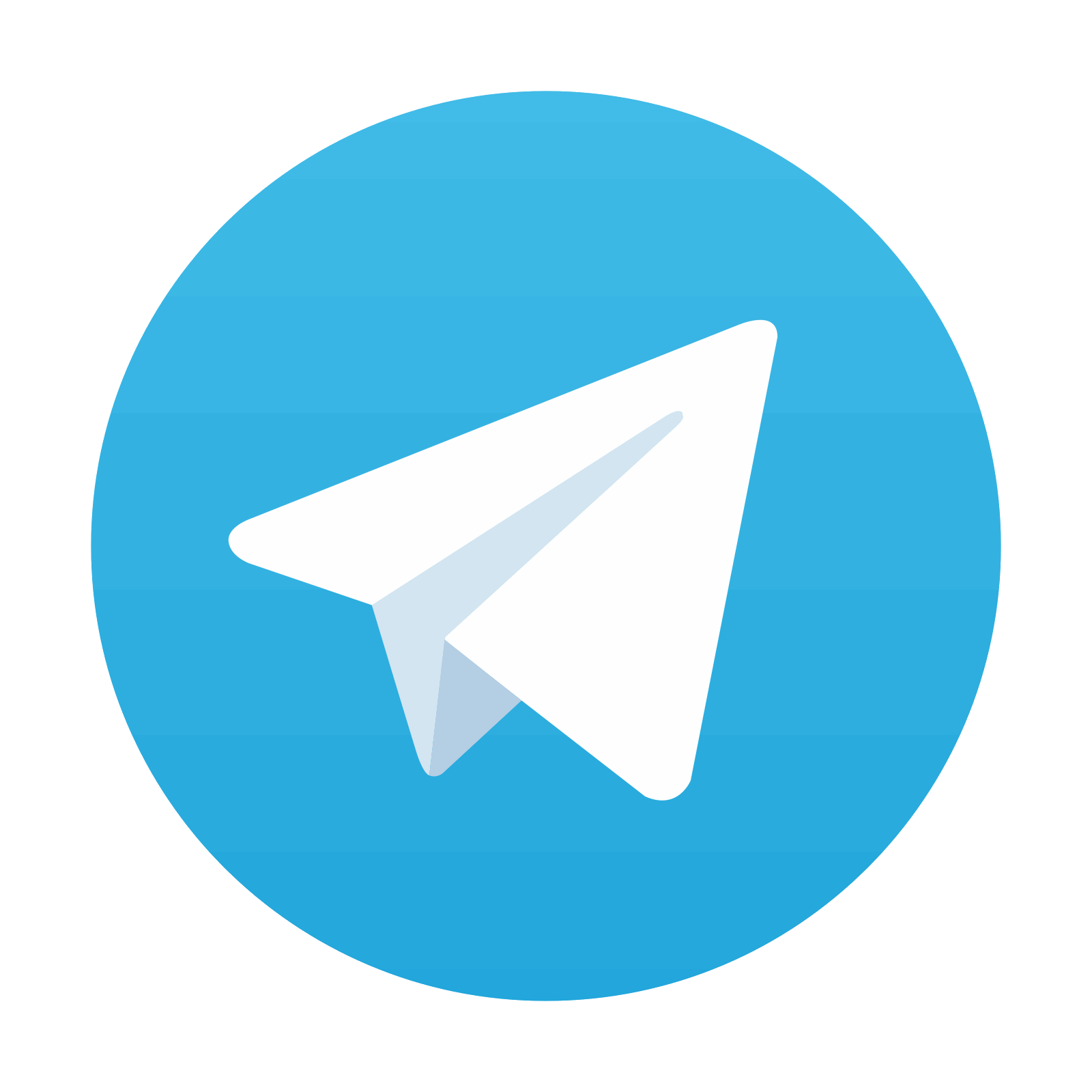
Stay updated, free articles. Join our Telegram channel

Full access? Get Clinical Tree
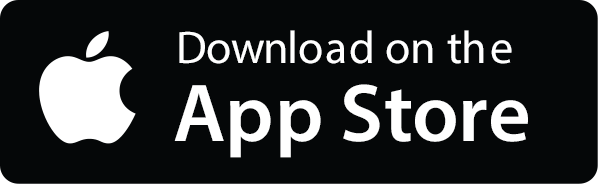
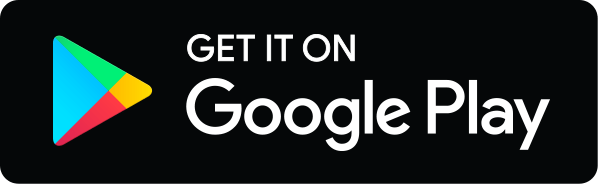