An Approach to Scar Mitigation
Fiona Wood
KEY POINTS
The ultimate goal is skin regeneration, not repair that results in scar formation.
Scar minimization begins at the time of the injury.
Multiple interventions can impact the ultimate scar outcome.
Fibrosis is the repair mechanism in all human tissues when the capacity to heal by regeneration is overwhelmed.1 The control of the process of fibrosis—the interplay between the cells and the extracellular matrix (ECM)—is key to retaining normal function of the organ.2 In scar minimization the aim is to control the process to facilitate wound healing while avoiding excessive or abnormal ECM with the associated functional impact.3
The skin is a dynamic organ, responsive to the environment and replenishing its surface continuously. However, when injured it has a limited capacity for regeneration, and the resulting scarring leads to functional and aesthetic sequelae.4 Cutaneous scarring may be classified related to its severity as normotrophic,5,6 hypertrophic,7,8 or keloid scarring.9 The severity of the scar worn for life is influenced by a range of factors related to the following:
Specific individual predispositions10
The severity and type of injury11
The interventions12
Complications during the healing process13
The focus of this chapter is on the minimization of scarring postburn injury. The complexity of the process provides multiple therapeutic opportunities to limit scarring along the clinical journey. The scar continues to turnover throughout life as clearly seen in children as they grow, also providing an opportunity for intervention.14
It is well described that burn injury is associated with an aggressive scarring response related to the stimulation of a severe systemic inflammatory response (see Chapter 6).15 However, there are many factors related to the pathophysiology and natural history of burn injury and recovery, which are common to all cutaneous injury repair responses (see Chapter 9).16 The injury and individual’s predisposition to scarring may not be amenable to direct influence at this time, but the knowledge of predisposition to poor outcome can guide timing and the type of intervention. The therapeutic opportunities to influence the scar outcome currently rely upon the understanding of the underlying mechanisms along the continuum of the total healing response.17 Further, the goal can be extended by the knowledge of regenerative repair to achieve the ultimate aim of tissue restoration with scarless healing (see Chapter 27).1,18
The fundamental premise for this chapter is: Every intervention from the point of injury will influence the scar worn for life. It is with this in mind that the potential for incremental gains at every stage of the healing process will be considered. Key to minimizing scarring is a knowledge of the science of the healing response from hemostasis, inflammation, vascularization, and deposition of the ECM, through to the final remodeling of the scarred area.19
Understanding the mechanism of injury is the initial step in the scar risk assessment; a clean surgical procedure will clearly carry less risk of pathologic scarring than a contaminated blast wound or a burn injury. Factors affecting the speed of healing will have a profound impact on scar outcome; these may be classified as systemic or local. For example, a diabetic patient with a secondary infection will heal relatively more slowly with an increased risk of scarring.13
Attention to detail is essential at every stage of care in all circumstances, whether the intervention involves a clean excision and suture of a cutaneous lesion or excision and repair of a burn wound. The focus of clinical care is aimed at bringing together “the triangle of care,” which is (1) the assessment of the patient’s condition, (2) the experience and knowledge of the clinician, and (3) the environment of operation.20
The chapter will explore the opportunities related to scar minimization by reduction in the time to healing, with specific reference to burn wound injury and scarring. The basic understanding of essential topics will be introduced to link the concept of meticulous attention to detail in clinical care along the entire journey with the current scientific knowledge.
Skin Structure and Function
Scar minimization involves treatment of skin injury with the aim of maintaining function. Although a comprehensive review is beyond the scope of this chapter,21 it is appropriate to spend a few moments considering the functionality
of skin and the impact of the injury and repair process on the range of functions.
of skin and the impact of the injury and repair process on the range of functions.
Skin consists of two main regions: (1) the outer epithelial tissue, the epidermis, which is a stratified squamous epithelium; (2) the dermis, which is the deeper thicker connective tissue layer. The tissue construct has cells derived from all three embryologic layers with specialized cell types and morphologic differences related to anatomical location.22 The scar response to injury varies in relation to the body site of injury, with modulated responses linked to the cell phenotype.23
The skin’s primary function is to act as a highly innervated and responsive protective barrier to the environment, restricting fluid losses and invasion of microorganisms. The secondary functions include vitamin D synthesis, thermoregulation, innate immune responses, as well as providing a range of homeostatic and sensory functions.21
As a complex multifunctional organ that is in a continuous state of regeneration, the epidermal layer is replaced every 6 to 8 weeks. The process of regeneration is stimulated in response to insult or injury, retaining a capacity of self-repair from the cells of the dermal-epidermal junction (DEJ) and skin adnexal structures.24 The epidermis is responsive to both the external environment and to the systemic pathophysiology; optimal function is associated with retention of the epidermal integrity.
Epidermal damage is a frequent occurrence, and in the vast majority of instances the cells of the DEJ and those surrounding the skin adnexal structures are able to respond: proliferating, migrating, and differentiating to achieve a regenerative repair.25 When the tissue damage is such that the capacity to regenerate is overwhelmed, the process of healing becomes associated with scarring. The time to achieve epidermal cover is directly related to the extent of injury.26 The keratinocyte phenotype modified by the healing process has an influence on the underlying fibroblasts involved in scar formation.27
![]() FIGURE 8-1 Integra dermal template introduced into a full thickness skin defect to facilitate dermal repair with a second-stage procedure to repair the epidermis. (Courtesy of Integra Life Sciences.) |
Although epidermal repair restores the essential barrier, the dermis provides the structural integrity and supports the skin adnexal structures and their functions, including the stem cell niche vital in regeneration and repair.
The balance of the turnover in the ECM is pivotal in the process of fibrosis and, hence, scar formation. An understanding of the processes of deposition and degradation of the matrix affords potential therapeutic opportunities.19 However, the capacity of the dermal element of the skin to regenerate is severely limited and scar does not retain the architectural framework required for the maintenance of the adnexal structure environments, with the resulting loss of the specific functions (see Chapter 5).28 Work to replace the dermis with a scaffold for cell migration and skin restoration has had some clinical success. Integra (Integra LifeSciences, Plainsboro, NJ) dermal template has been in clinical use for some decades and is a construct of bovine collagen and glycosaminoglycans with an average pore size of 80 µm.29 When introduced into a full thickness skin defect, the cells migrate into the scaffold to establish a vascular network; the fibroblasts express a reticular dermal phenotype through a process of tissue-guided regeneration limited to the dermal ECM (Fig. 8-1). There is an impairment of neural ingrowth
into the construct and, at this stage of the technology, no restoration of hair follicles, sweat glands, or sebaceous units.30
into the construct and, at this stage of the technology, no restoration of hair follicles, sweat glands, or sebaceous units.30
Despite the restoration of the primary barrier functions, the scar will not function as normal skin, with significant differences seen in the architectural framework, chemistry of the ECM, and the cell phenotype. Clear differences are seen with the loss of skin and adnexal structures, and when investigating the impact on the peripheral nerve fields within the scar repair.30 In some cases the extent of the skin loss is so great that it is life threatening (as in acute burn injury) with fluid loss and vulnerability to infection.31
In all cases the restoration of a full thickness skin construct specific for the functionality of the given body site is the ideal goal.24 An understanding of the functions lost in the repair process directs us down a path of tissue salvage and guides the tissue engineering efforts to manufacture more than a two-layered structure of dermis and epidermis. It is clear that exploration of regenerative therapies for the restoration of the skin is the ultimate aim for full functional recovery.25,32
Basic Wound Healing
A breach in skin integrity results in the initiation of a complex series of overlapping processes driven locally and systemically.33 The systemic optimization is a fundamental aspect of facilitating wound healing and is considered in the holistic approach to the assessment and treatment of the patient. The factors of influence are wide ranging, inclusive of pain, stress, nutrition, comorbidities, and cultural practices. Taking the time to consider all the factors and engagement in correction where possible will help in improving the clinical outcome.
The wound healing process is described as consisting of three phases—inflammation, tissue formation, and tissue remodeling (Fig. 8-2). An understanding of the underlying mechanisms provides therapeutic opportunities as highlighted in a review of the current literature.34
There are opportunities to improve clinical outcome by understanding the basic science behind our clinical observations. Initial hemostasis observed as clotting postinjury is associated with platelet degranulation and cytokine release that drives the inflammatory response. Endothelial cell changes allow cells and fluid to migrate from the circulation into the extracellular space.35 The resulting edema is an essential aspect of the healing response, but can cause ongoing cellular and tissue damage if not controlled. The fluid shifts that increase proportionally with the percent of total body surface area (%TBSA) involved in burn injury may lead to significant pathology, possibly leading to compartment syndrome requiring targeted therapy.36
![]() FIGURE 8-2 The phases of wound healing from injury to repair. (Courtesy of www.worldwidewounds.com.) |
As the process of tissue formation progresses, manipulation of the cellular pathway can influence the balance of necrosis and apoptosis, with the potential for tissue salvage and limiting the ongoing inflammatory drive.37 Tissue formation relies on the interaction of the cells and ECM, with the migration of fibroblasts to restore the dermal elements and keratinocytes to restore the epidermis. The processes are dynamic and a thorough knowledge of the applicable mechanisms provides opportunities for intervention over time, targeting the specific processes with an understanding of the triggers to phenotypic expression.38 Investigation of the epigenetic processes in wound healing and scarring is important in understanding the potential for them to be returned to a regenerative state.39 The methylation patterns seen in skin pathologies may also give insight into novel therapies.
Transforming growth factor (TGF)-β, a protein extensively investigated as a controller of cellular proliferation and differentiation in wound healing, is an example of how mechanistic understanding can drive therapeutic development.40 It is a significant regulator of collagen deposition in the scarring process. Key to the feedback pathways for collagen buildup is the extracellular cross-linking forming the ECM framework, a process that maintains the aberrant collagen structure. The enzyme required for the cross-linking of collagen within the matrix is lysyl oxidase; its inhibition may provide an opportunity to manipulate the scar at the remodeling stage.41
The understanding of the basic science is an area of intense activity. From these brief examples it is clear that there is real potential for integrating the knowledge into clinical care and scar minimization.
Burn Wound Pathophysiology
A basic understanding of burn injury responses is essential in linking the extent of the injury, the wound healing process, and the potential to improve clinical outcome by scar reduction.
In burn injury the tissue damage is well described by Jackson’s burn wound model42 (Fig. 8-3). The energy of the injury in contact with the skin results in an area of tissue destruction with associated cell death. The zone of necrosis is nonsalvageable and will be a significant driver of the overall scar outcome. In the future, mechanisms of repair might be found that could be applied to chemically repair denatured proteins.43 Damage to ECM architecture and chemistry and cellular disruption are all triggers to inflammation.37 The necrotic tissue interface is the driver of both the local and systemic inflammatory responses, which are essential for wound healing and also a driver of the pathologic scar when uncontrolled.44
The surrounding viable tissue rapidly becomes edematous as the loss of capillary integrity leads to the loss of fluid and small molecular weight proteins into the extracellular space along with cell migration. This zone of stasis is tissue that is potentially salvageable with appropriate intervention. Focusing therapy on salvage of this tissue will reduce the overall scarring.45
The surrounding zone of hyperemia is the primary vascular response to injury that facilitates the healing process. Much of the work to date has focused on the maintenance of the circulation to maintain vital organ function, specifically as the surface area of burn injury reaches 10 to 20 %TBSA and the associated fluid shifts compromise the circulation requiring fluid resuscitation.46 The fluid resuscitation needs to be titrated to the patient’s response in order to maintain the circulation, but also to limit the extent of the secondary edema and minimize the extension of the zone of stasis. As the zone of stasis extends, the relative hypoxia triggers apoptosis and necrosis, thereby extending the zone of necrosis. The resulting scar is directly related to the extent of the zone of necrosis. Therefore, limiting the zone of necrosis will have a direct effect on the scar outcome.47
The extent of the systemic responses postburn injury are such that as the %TBSA increases, the injury has a life-threatening impact systemically driven by the breach in barrier function of the skin. It is well known that the responses to burn injury are more severe than excisional injury, with a greater risk of severe pathologic scarring.37 Reducing the time to healing is the key to scar minimization. The resulting fluid shifts and cellular responses, locally and systemically, are all opportunities to improve therapies by understanding the underlying mechanisms and targeted therapy.
Because the burned tissue drives the systemic responses, one solution would be burn wound excision. However, the excision of the wound must be undertaken in an appropriate environment based on a risk analysis of the patient, the assessment of the wound, and the environment of operation.
Assessment of the Individual’s Risk of Scarring
There are well-documented factors that need consideration when assessing the individual’s risk of scarring.48 The age at the time of injury is known to influence not only survival, but the quality of survival and ultimate scar outcome. Therefore, this information needs acknowledgment in clinical decision making.49 The elderly generally scar less but heal more slowly, and may have less tolerance of interventions such as surgery.50 The young will scar more aggressively, and attention to reducing the time to healing requires special consideration.51 The general condition of the patient in terms of nutrition, fitness, stress, in addition to smoking drugs and alcohol, has the potential to influence the healing trajectory and hence scarring (see Chapter 9). Further, there are a range of comorbidities such as diabetes, vascular disease, autoimmune disease, and medications such as steroids, all with the potential to influence healing and scarring. A clear understanding of the patient’s condition is essential information in both clinical decision making and therapeutic interventions to improve the capacity to heal.52
Racial differences in scarring are well documented in the literature, with the genetic predisposition demonstrated to affect the level and quality of scar tissue formation in the wound.53 With investigation of the genetic data, there appears to be a range of genes involved (e.g., African American differing from Han Chinese).54 When investigating keloid disease, at the individual level the inheritance pattern is thought to be autosomal dominant with variable penetrance.55
Genetic screening studies have investigated some positive associations between polymorphisms in the major histocompatibility complex and scarring. The immunogenetic component to keloids and hypertrophic scars could potentially be explained as differing stages of the same disease, with different degrees of inflammation affected by genetic predisposition.56 For example, investigation of known pathways has shown associations between a polymorphism in TGF-β associated with poor scar outcome after melanoma removal,57 and between a polymorphism in the cell cycle regulator p27 and poor scar outcome after burn injury.58
Keloid formation is also described in some syndromes such as Rubinstein-Taybi syndrome,59 Goeminne syndrome,60 and Ehlers-Danlos syndrome,61 with described genetic links. The investigation of the epigenetic pattern, which drives the cell phenotype, has shown differential
regulation in a number of genes when comparing cells from scar and normal skin of the same individual. Further understanding of the underlying genetic links will provide the opportunity to better understand underlying mechanisms and investigate new therapeutic targets.
regulation in a number of genes when comparing cells from scar and normal skin of the same individual. Further understanding of the underlying genetic links will provide the opportunity to better understand underlying mechanisms and investigate new therapeutic targets.
When planning intervention, a holistic assessment of the patient is essential to understanding scar risk and tailoring the interventions where possible. It is clear that further investigation of the differential scar risk at the genetic and epigenetic levels will provide opportunities for targeted care in the future.
Burn Wound Assessment
The capacity to heal by regeneration without scar is limited as the cells of the DEJ and the skin adnexal structures are damaged.49 It is well known that the time to healing is a key driver of scar outcome.26 The most significant factors driving the severity of the long-term scar postburn injury are the %TBSA of skin involved and the depth of the injury.62 As the %TBSA increases, the systemic response and applicable interventions may also be associated with burn wound extension, increased tissue loss, and decreased capacity for healing.63
The use of clinical assessment and advancing technology along the clinical pathway is essential in guiding current interventions and measuring the impact of advancing therapies to reduce scarring. The %TBSA is based on a clinical assessment using the Lund and Browder chart and the rule of nines64 (Fig. 8-4). The clinical assessment of the depth of injury based on appearance, blood supply, and sensation is complex and needs to be done with the understanding that the wound will change over time and repeated assessment is needed to guide treatment.65 Laser Doppler imaging has been introduced to improve the accuracy of assessment by measuring the blood flow as an indicator of tissue viability; specifically, to guide the treatment in partial thickness wounds.66 The application of other techniques based on color (e.g., spectroscopy) and in situ tissue imaging (e.g., optical coherence tomography)67 provides opportunities to improve the understanding of the capacity of the injured skin to heal.
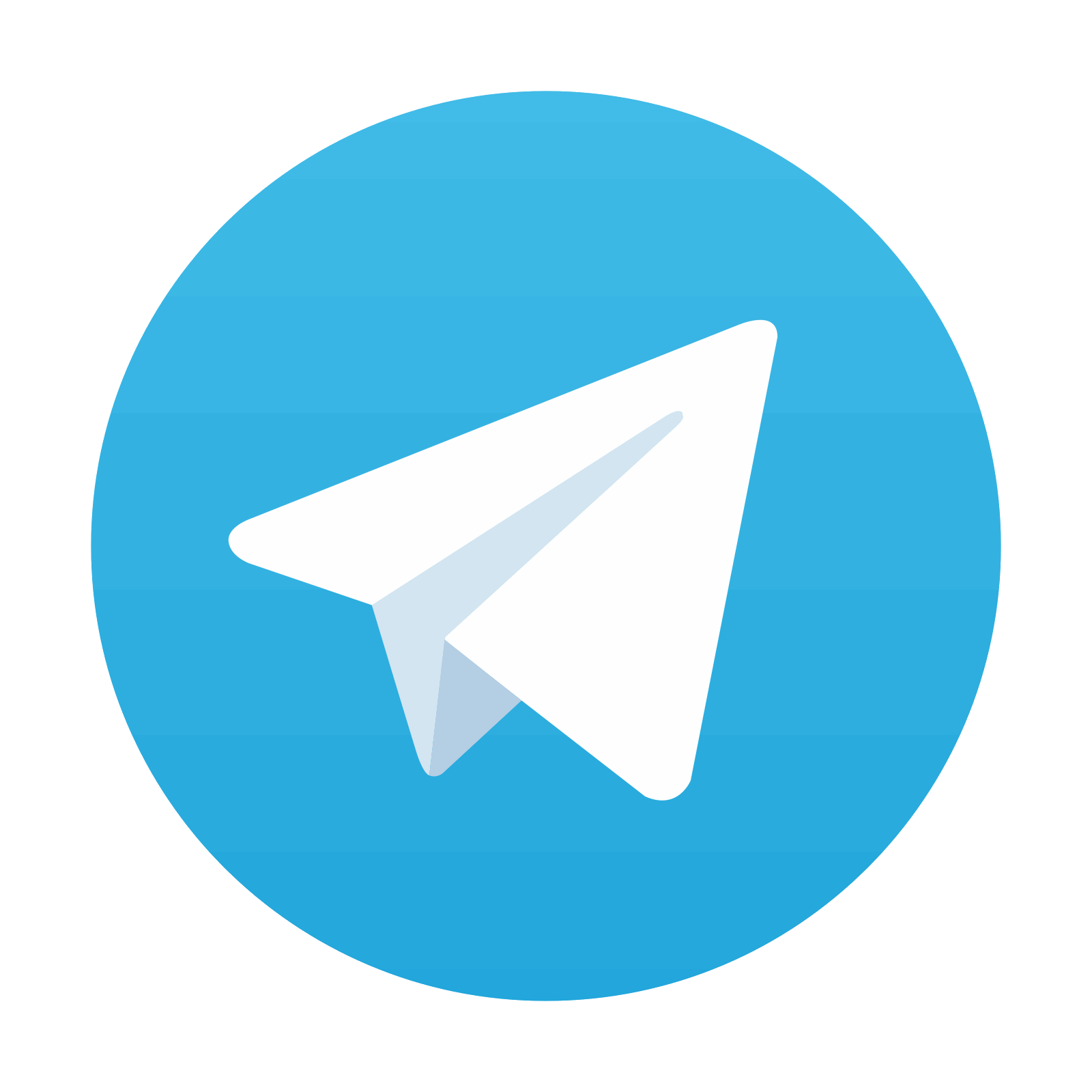
Stay updated, free articles. Join our Telegram channel

Full access? Get Clinical Tree
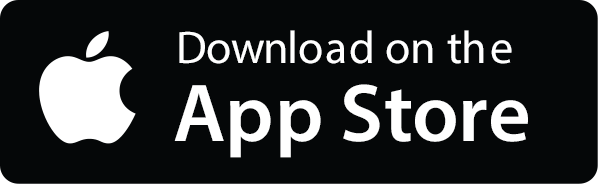
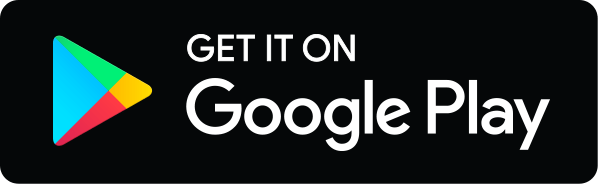