Abstract
Exposure of the skin to UV light leads to both acute, short-term effects (sunburn and tanning) and chronic, long-term effects (photocarcinogenesis and photoaging), all of which are wavelength-dependent. All organisms have evolved mechanisms for protecting against UV-induced killing and the genome-damaging properties of UV light. For human skin, these include DNA repair processes, immune-mediated removal of damaged cells, and anti-oxidative defense systems.
For photophysical and photochemical effects to occur, the energy of the UV light must be absorbed by a chromophore, e.g. DNA in the case of UVB. UV light induces different types of wavelength-dependent DNA damage, including pyrimidine dimers and oxidative guanine base modifications, which lead to mutation formation. Some base substitution mutations (C→T and CC→TT) are so typical for UV light that they have been termed “signature mutations” and they are commonly found in cutaneous carcinomas arising in sun-exposed skin. In order to address these changes in the DNA sequence, there are several repair pathways – nucleotide excision repair, base excision repair, translesional DNA synthesis, and recombination repair.
UV light clearly affects the skin’s immune system, exerting both pro- and anti-inflammatory responses. Proinflammatory effects are exemplified by sunburn and are mediated primarily by innate immunity. Anti-inflammatory/immunosuppressive effects are mediated in large part by adaptive immunity; by inhibiting immune recognition of UV-damaged cells, they play an important role in photocarcinogenesis. The positive aspect of UV-induced immunosuppression may be the prevention of autoimmune responses triggered by UV-induced cell damage and unmasking of autoantigens.
Keywords
photoimmunology, photoaging, photocarcinogenesis, sunburn, tanning, action spectrum, DNA photoproducts, DNA repair, photomutagenesis, xeroderma pigmentosum, UVA, UVB, UV light
- ▪
Exposure of the skin to UV light has acute, short-term effects and chronic, long-term effects, both of which are wavelength-dependent. Profound effects also occur with non-erythemogenic doses of UV light
- ▪
UV light affects the skin’s immune system, exerting both pro- and anti-inflammatory responses
- ▪
UV light induces several different types of DNA damage in a wavelength-dependent manner, such as pyrimidine dimers (UVB ≫ UVA) and oxidative guanine base modifications (primarily UVA); pyrimidine dimers are the most important pre-mutagenic DNA lesions
- ▪
Several DNA repair pathways are involved in the processing of UV light-induced DNA damage, including nucleotide excision repair, base excision repair, translesional DNA synthesis, and recombination repair
- ▪
UV light-induced mutations, which play a pivotal role in photocarcinogenesis, are different from those induced by other mutagens. Some base substitution mutations (e.g. C→T, CC→TT) are so typical for UV light that they have been termed “signature mutations”
- ▪
Disorders with an increased frequency of UV-induced skin cancers are characterized by an impaired cellular or host response to the effects of UV light
Introduction
Dermatologists are confronted daily with the effects of UV light on the skin, and often deal with them in a seemingly contradictory way. On the one hand, they have the responsibility of warning their patients against the deleterious effects of sunlight, such as sunburn, photoaging and sun-induced skin cancers, and on the other hand, they use UV irradiation to treat skin disease. As with all medical interventions, dermatologists are weighing the risks versus the benefits when they use UV light for therapeutic purposes. In the phototherapy of inflammatory skin diseases, dermatologists also try to avoid the proinflammatory properties of UV irradiation (e.g. the capability to induce sunburn) by choosing suberythemogenic doses (see Ch. 134 ). This clearly indicates that doses of UV light that do not induce sunburn still have profound effects on the skin. This applies not only to its short-term effects, but also to its long-term effects. A third and important aspect of photodermatology is the diagnosis and treatment of photosensitive or photoaggravated skin disorders (see Ch. 87 ).
Spectrum of UV Light and Absorption Spectra
The sun emits UV radiation as part of an electromagnetic spectrum (see Fig. 134.1 ). It is usually subdivided, rather arbitrarily, into UVC (200–290 nm), UVB (290–315 nm), and UVA (315–400 nm). UVA has been further subdivided into UVA1 (340–400 nm) and UVA2 (315–340 nm). Of note, some authors use 320 nm as the demarcation between UVA and UVB. More than 95% of the sun’s UV radiation that reaches the earth’s surface is UVA. Practically all of the UVC, and much of the UVB, are absorbed by the oxygen and ozone in the earth’s atmosphere, so that UV radiation below 290 nm is virtually undetectable at ground level. Nevertheless, the remaining UV radiation can still be absorbed by biologic molecules (DNA, protein, lipids), and it can damage and kill cells.
To survive in our environment, all living organisms had to develop protective mechanisms in order to prevent UV-induced killing and to maintain the stability of their genome. Such defenses include the development of UV-absorbing surface layers, enzymatic and non-enzymatic anti-oxidative defenses, repair processes, and removal of damaged cells. Through evolution, humans have lost most of the UV-protective fur, which remains an effective UV-protector only on the scalp. Nonetheless, the human skin is quite effective in protecting the rest of the organism from the harmful effects of solar UV irradiation, since UV radiation does not penetrate any deeper than the skin. Within the skin, the depth of penetration of UV light is wavelength-dependent – i.e. the longer the wavelength, the deeper the penetration ( Fig. 86.1 ). While UVA readily reaches the dermis, including its deeper portions, most of the UVB is absorbed in the epidermis, and only a small proportion reaches the upper dermis. UVC, if it reached the earth’s surface, would be absorbed or reflected predominantly by the stratum corneum and the upper layers of the epidermis.

However, when thinking about the biologic effects of different wavelengths in the different layers of the skin, it is necessary to consider that a particular wavelength can even have a biologic effect in a layer that it does not reach. For example, secretion of a proinflammatory mediator in the epidermis can produce subsequent signaling in the dermis and possibly even the subcutaneous fat .
Some of the UV light reaching the skin is absorbed by biomolecules. A light-absorbing molecule is called a chromophore. Upon absorption of the radiation’s energy, this chromophore is elevated to an excited state. Ensuing photochemical reactions may either change the chromophore directly, or, through energy transfer in a so-called photosensitized reaction, indirectly change a molecule other than the chromophore. Absorption is wavelength-dependent and if a photon (depending upon its wavelength) is not absorbed, a photochemical reaction cannot ensue. For UVB, the most important chromophore is DNA while a typical UVA- and visible light-absorbing chromophore is porphyrin.
Knowledge of absorption spectra of molecules is critical for understanding photobiologic consequences of UV absorption, including photomutagenesis of DNA by UVB, the efficacy of UV filters in sunscreens, and the elicitation of photodermatoses by certain wavelengths of UV light. An absorption spectrum defines the probability that a molecule will be excited by a certain wavelength. While the absorption maximum predicts the wavelength at which absorption is most likely, other wavelengths can still be absorbed. For example, the absorption maximum of DNA is 260 nm, which is within the UVC range. However, UVB still effectively excites DNA, and even UVA and visible light can do so. This explains why DNA photoproducts, a type of DNA damage induced by a photochemical reaction following direct excitation of the DNA molecule, are not only induced by UVB, but also by UVA, and even by visible light, albeit at a much lower efficacy.
Both short- and long-term effects of exposure to UV light are wavelength-dependent. However, when comparing the photobiologic properties of UVB with those of UVA, it is important to remember that they are not two different entities, but rather a continuum of wavelengths, with gradually changing properties, and that the division between UVA and UVB is rather arbitrary.
Short-Term Effects of Ultraviolet Light: Sunburn and Tanning
Visible, short-term, cutaneous effects of UV irradiation include sunburn (solar erythema and possibly blister formation, followed by desquamation) and tanning. Microscopically, short-term effects include epidermal edema with spongiosis, formation of sunburn cells (apoptotic keratinocytes), acanthosis, hyperkeratosis, depletion of Langerhans cells, and an increase in basal layer and suprabasal melanin content, in addition to inflammatory infiltrates of lymphocytes and neutrophils and vasodilation. On a cellular and molecular level, UV exposure induces a multitude of damage responses, including the induction of stress proteins, repair processes, and cytokine production (e.g. IL-1, IL-6, TNF). In a dose-dependent manner, exposed cells can either undergo apoptosis (visible histologically as sunburn cells) or cease proliferating (cell cycle arrest) in order to undergo repair. Hyperproliferation may follow the initial growth arrest, visible histologically as epidermal thickening. The inflammatory response in the sunburn reaction is likely due to an activation of innate immunity . Lastly, UVB-induced activation of transient receptor potential ion channels in keratinocytes with subsequent calcium influx has been shown to mediate sunburn pain .
The ability to induce sunburn ( Fig. 86.2 ) rapidly declines with increasing wavelength. For example, UV light with a wavelength of 360 nm is approximately 1000-fold less erythemogenic than light with a wavelength of 300 nm. A UVB-induced sunburn reaches its peak between 6 and 24 hours after exposure. An immediate erythema reaction is rarely observed. In contrast, after exposure to a very high dose of UVA, an immediate erythema reaction can occur, followed by a distinct delayed erythema after 6 to 24 hours. However, unless patients have increased UV sensitivity (e.g. decreased minimal erythema dose [MED] to UVA; see Ch. 134 ), the doses of UVA present in natural sunlight are not sufficient to induce a sunburn. DNA is thought to be the chromophore for the delayed erythema reaction associated with UVB .

The tanning response of human skin to sun exposure is biphasic and also wavelength-dependent. Immediate pigment darkening occurs during and immediately after exposure, and is due to alteration (e.g. oxidation) and redistribution of existing melanin. It is most prominent with UVA. Delayed tanning is usually the result of an exposure to UVB, and it peaks approximately 3 days after sun exposure. Fair skin (skin type II) usually tans only with UVB doses above the erythema threshold, i.e. only with prior sunburn. Darker skin (skin types III and higher) also has a significant tanning response to suberythemogenic doses, i.e. without a prior sunburn. A UVB-induced tan is characterized by an increased number of melanocytes, increased melanin synthesis, increased arborization of melanocytes, and increased transfer of melanosomes to keratinocytes (see Ch. 65 ). A UVA-induced tan, such as from the use of a tanning bed ( Fig. 86.3A ), provides 5–10 times less protection against a sunburn from subsequent UV exposure than a UVB-induced tan, probably because a UVA-induced tan is characterized by less pronounced epidermal thickening and hyperkeratosis.

An individual’s tendency to develop sunburn and tanning after sun exposure has been used to categorize skin phototypes (see Table 134.3 ). These categories of an individual’s susceptibility to short-term effects also correlate with the individual’s susceptibility to long-term effects following sunlight exposure. In general, those individuals with higher acute sun sensitivity are also at greater risk for developing skin cancer after chronic UV exposure.
Effects on the Cutaneous Immune System
A sunburn exemplifies the profound effect that UV light has on the skin’s immune function, in this instance a proinflammatory effect. This effect, plus the clinical observations outlined in Table 86.1 , provide evidence for the proinflammatory/immune-stimulating effects of UV light on the skin. On the other hand, UV light clearly has anti-inflammatory/immunosuppressive properties as well, exemplified by the efficacy of UV phototherapy in the treatment of inflammatory skin disorders. Currently, immunostimulatory effects of UV light are thought to be due primarily to an activation of innate immunity, with immunosuppressive effects mostly due to an inhibition of adaptive, antigen-specific immunity . However, a simple dichotomy is unlikely as there are multiple individual factors that also influence these immune effects, including varying dose- and wavelength-dependent differences that go beyond variations in skin phototype. An example of individual factors would be the observation that the majority of patients with psoriasis improve with UV phototherapy, but a small minority flares. Another example would be patients with polymorphic light eruption in whom inhibition of contact hypersensitivity by UV light is reduced , presumably predisposing them to reactions to photo-induced allergens or toxins .
EFFECTS OF ULTRAVIOLET LIGHT ON THE IMMUNE SYSTEM |
Clinical observations |
Suggestive of proinflammatory/immune-stimulating effects |
|
Suggestive of anti-inflammatory/immunosuppressive effects |
|
Cellular and molecular events in UV-irradiated skin |
Mediation of proinflammatory/immune-stimulating effects (innate immunity) |
|
Mediation of anti-inflammatory/immunosuppressive effects (adaptive immunity) |
|
UV light-induced effects on the immune system involve not only the areas of skin directly exposed to UV light, but also non-irradiated sites. An example of systemic immunosuppression is the development in non-exposed skin of antigen-specific immune tolerance to antigens that were initially applied to UV-exposed skin. This effect is known to be mediated by the release of immunosuppressive cytokines such as interleukin (IL)-10 and then ultimately by UV-induced regulatory T cells. An example of systemic immune-stimulating effects is the UV-induced exacerbation of systemic disease in patients with systemic lupus erythematosus (LE).
The downside of photo-induced immunosuppression and immune tolerance is that they inhibit recognition of tumor antigens and thereby contribute to photocarcinogenesis. The upside is the prevention of autoimmune reactions, e.g. conditions like LE would probably be more prevalent without effective suppression of immune reactions against self-antigens unmasked by UV-induced cell damage. Such equilibrium is exemplified by polymorphic light eruption, where less efficient UV-induced immunosuppression is counterbalanced by a lower risk of UV-induced skin cancers .
Cellular photoreceptors that mediate the signaling that leads to UV-induced immune responses are: DNA (via formation of DNA damage), urocanic acid in the stratum corneum (via UV-induced isomerization from the trans – to the cis -isoform), and membrane lipids (via UV-induced alteration of the membrane redox potential). While the immunosuppressive effects of UVB are well established, the relative contribution of UVA to the immunomodulatory properties of solar UV irradiation is still a matter of debate, but evidence is accumulating that UVA also has prominent effects .
Long-Term Effects of Ultraviolet Light: Photoaging and Photocarcinogenesis
Long-term effects of chronic sun exposure include photoaging and photocarcinogenesis. In the case of photocarcinogenesis, UV exposure provides a one-two punch – not only does it generate DNA damage that leads to mutation formation and malignant transformation, but its immunosuppressive properties, including the induction of specific tolerance to antigens from UV-induced skin tumors, reduce the ability of the host immune-defense system to recognize and remove malignant cells. UV irradiation also impairs immune surveillance against cells infected with oncogenic viruses (e.g. certain HPV types) and thereby further promotes skin cancer formation. This may explain why cutaneous squamous cell carcinomas (SCCs) develop more frequently than basal cell carcinomas (BCCs) in immunosuppressed patients (e.g. solid organ transplant recipients), in contrast to immunocompetent individuals, in whom BCCs occur more commonly than SCCs.
The advantage of reduced immune reactivity following exposure to UV light is the prevention of illicit (auto)-immune reactions to transiently UV-altered cells (see above). Any shift in the balance between pro- and anti-inflammatory responses toward a more immunosuppressed state increases the risk of UV-induced tumor formation, as occurs in chronically immunosuppressed patients. Tilting this balance toward proinflammatory responses and autoimmunity increases the risk of developing photodermatoses or photoaggravated skin diseases such as LE. The critical nature of this balance is also exemplified by the observation that effective photoprotection commonly leads to spontaneous resolution of actinic keratoses, even though these precursor lesions have identifiable genetic changes that provide growth advantage over non-mutated cells (see Ch. 107 ).
Photoaging
Photoaging encompasses the clinical, histologic and physiologic changes present in chronically sun-exposed skin. This extrinsic skin aging is classically distinguished from intrinsic aging, which refers to age-dependent changes in sun-protected skin. Photoaging affects all components of the skin, including the epithelium, the pigmentary system, the vasculature, the dermal connective tissue, and possibly even the subcutaneous fat .
Due to its ability to penetrate deeper into the dermis, UVA is thought to play an important role in inducing the dermal changes of photoaging. Figure 86.4 depicts an office worker with unilateral facial photoaging that reflects consistent exposure of one side of her face via a window at work for 15 years . This is not an isolated event as additional examples of unilateral photoaging have been reported . Because UVA, but not UVB, penetrates through window glass, these cases convincingly demonstrate the ability of UVA to induce photoaging. That said, wavelengths of UV light that do not reach the dermis, in particular UVB, could still contribute to photoaging of the dermis via signaling originating from the epidermis . Such signaling may even mediate the loss of subcutaneous fat seen with photoaging .

In addition to differences in their depths of penetration, UVA and UVB also produce different cellular effects, likely influencing their photoaging effects. For example, UVA is particularly effective in inducing the following: a common deletion in mitochondrial DNA; the aging-associated and senescence-inducing protein progerin (see Ch. 63 ); and the elastin-degrading intracellular protease cathepsin K. These effects are likely mediated by UVA-induced oxidative stress and formation of reactive oxygen species . Of note, intrinsic aging, not just of the skin, has also been linked to oxidative stress. Thus, at least some features of photoaging could be considered as a process of accelerated intrinsic aging.
The deeper penetration of UVA into the skin is not thought to be as important in photocarcinogenesis as it is in photoaging, since UV-induced skin cancers – SCCs, BCCs and melanomas – arise from cells that reside within the epidermis, not from dermal cells. It is somewhat difficult to reconcile that this difference (between epidermal and dermal cells) in their susceptibility to UV-induced carcinogenesis is due solely to the more protected location of fibroblasts within the skin, given that fibroblasts are located just underneath the basal layer of epidermal cells. In mice, fibrosarcomas are readily induced by UVB, suggesting that the fibroblast is also susceptible to UV irradiation; therefore, it may be that it is solely the thickness of the human epidermis that prevents the malignant transformation of the fibroblast.
In contrast to photocarcinogenesis, where the anti-inflammatory effects of UV light play a pivotal role, photoaging is thought to be the result of chronic and repetitive inflammatory responses to UV light. This results in upregulation of extracellular matrix-degrading proteases and down-regulation of collagen synthesis, with subsequent loss of collagen and deposition of abnormal degenerative elastotic material within the dermis (actinic elastosis) over time. However, if the latter were merely due to UV-induced inflammation, wrinkling and other features of photoaging should be reversible with complete photoprotection, but of course this does not occur. Explanations for this lack of reversibility include UV-induced mutations of mitochondrial DNA , leading to loss of cellular functions, and shortening of telomeres as a consequence of cellular DNA damage responses, leading to cellular senescence .
UV-Induced Tumor Formation
It is well established that exposure of the skin to UV light is a major risk factor for the development of cutaneous melanoma and non-melanoma skin cancer . It is a process that, like cancer in general, involves a stepwise accumulation of specific genetic changes in a single cell, with subsequent clonal expansion. Usually, it takes decades until a tumor arises and in one study, over 25% of epidermal cells in aged chronically sun-exposed skin were found to contain cancer-causing mutations . UV-induced skin cancers (BCCs, SCCs, and melanomas of sun-exposed sites) develop along the photocarcinogenesis chain of events outlined in Figure 86.5 . The three major steps are:
- •
DNA damage formation after UV exposure
- •
mutation formation following DNA damage formation
- •
malignant transformation following mutation formation in specific oncogenes and tumor suppressor genes.

There is still some controversy regarding which wavelengths of the UV spectrum are responsible for these effects. While the carcinogenic properties of short-wavelength UV light, in particular UVB, have been well established for some time, UVA was originally thought to be harmless due to its relative inability to induce sunburn. More recently, however, it was shown that UVA is capable of inducing cutaneous SCC in mice and is now recognized as an independent class I carcinogen . Although a published photocarcinogenesis action spectrum for cutaneous SCC in mice showed a steep decline in tumor rate with increasing wavelength , it does not completely exclude a role for UVA. This is because natural sunlight contains much more UVA than UVB (depending on the time of day and weather: 20- to 100-fold more), which offsets at least some of the weaker effects of UVA. Additionally, UVA is less filtered by window glass or clothing and it penetrates more effectively to the basal layer of the epidermis, representing two factors that further increase the relative contribution of UVA to the carcinogenic properties of sunlight.
Although even less is known about the action spectrum for the induction of melanoma, it is known that the burden of UV-signature mutations varies from low in acral and mucosal melanomas to high in lentigo maligna melanoma. Support for a role for UVA comes from several sources , including the increased risk of developing melanoma following the use of tanning beds . A recent case–control study found adjusted overall melanoma risks of 2.86 and 4.44 for users of UVB-enhanced and primarily UVA-emitting indoor tanning units, respectively . In a mouse melanoma model, both UVB and UVA were shown to induce melanoma . However, for UVA this required the presence of melanin, as melanomas were not induced by UVA in albino mice, suggesting that UVA induces melanoma via a different mechanism than UVB . Oxidative photosensitized reactions via photoexcitation of melanin have been proposed, but not yet proven .
One hypothesis to explain why melanomas commonly arise in intermittently sun-exposed skin rather than chronically sun-exposed areas is that because of their relative inability to undergo apoptosis, melanocytes might be more prone to UV-induced mutagenesis following a single high dose of UV light . Melanocytes contain high levels of anti-apoptotic proteins such as Bcl-2, thus providing a possible explanation for why sunburn-induced apoptosis has not been described in melanocytes. As a result, melanocytes with a high amount of DNA damage are more likely to survive than are keratinocytes, which readily undergo apoptosis.
In chronically sun-exposed skin, adaptive responses that follow repeated exposures to low-dose UV radiation, such as increased pigmentation and upregulation of DNA repair, help to protect both melanocytes and keratinocytes against further mutagenic assaults from continued low-dose UV exposures. However, in this setting, keratinocytes are more prone to UV-induced mutagenesis because they are proliferating more rapidly and are therefore more likely to replicate unrepaired DNA. This could explain why keratinocytes are more vulnerable than melanocytes to the mutagenic effects of the repeated low-dose UV irradiation that are observed in chronically sun-exposed skin.
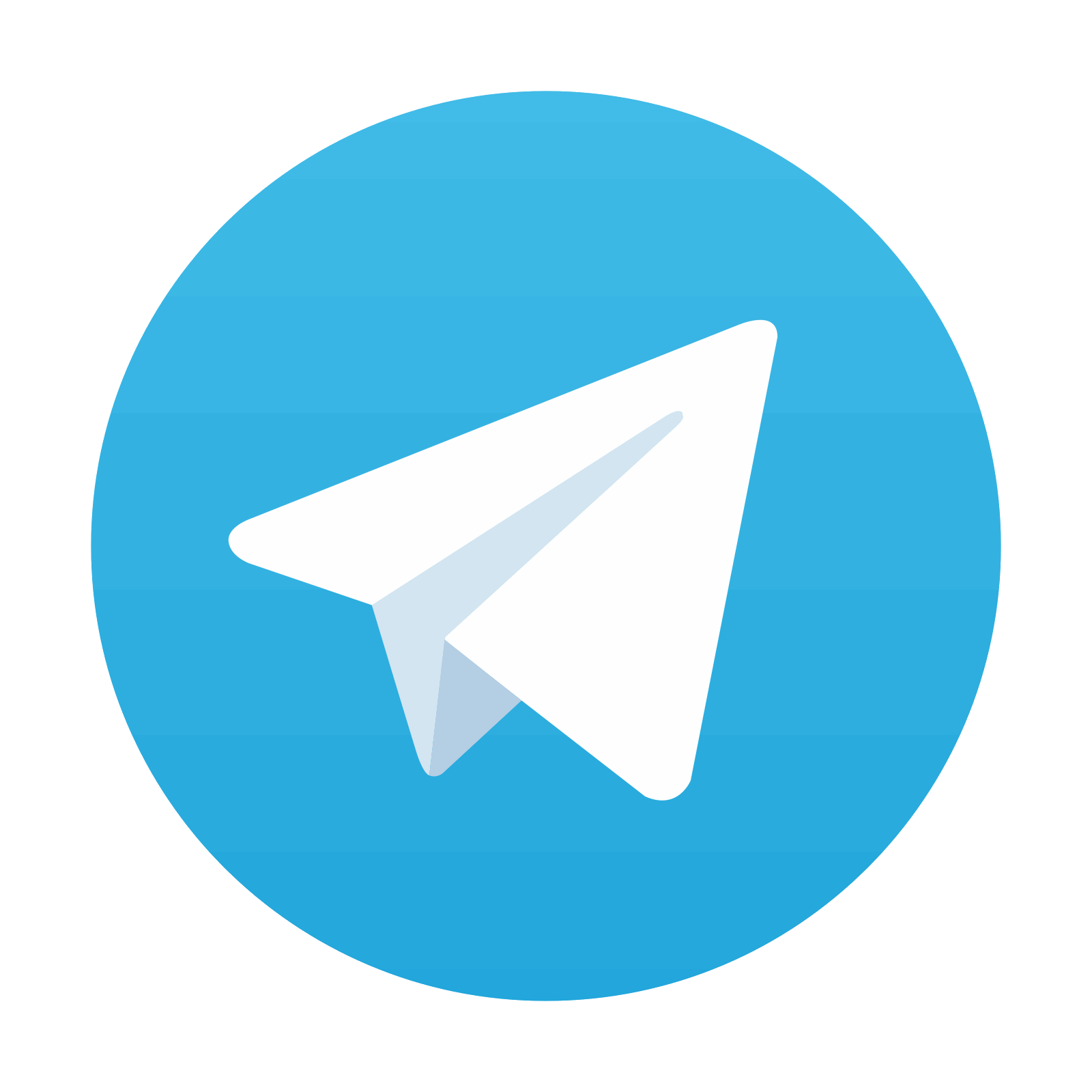
Stay updated, free articles. Join our Telegram channel

Full access? Get Clinical Tree
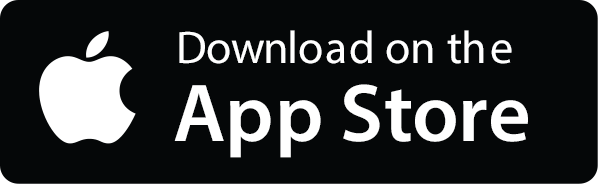
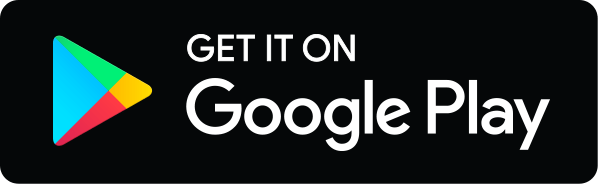