Facial skin defects created by Mohs micrographic surgery are commonly reconstructed using local cutaneous flaps from surrounding skin. To provide optimal survival and aesthetic outcomes, the cutaneous surgeon must command a thorough understanding of the complex vascular anatomy and physiology of the skin as well as the imperative physiologic and biomechanical considerations when elevating and transferring tissue via local skin flaps.
Key points
- •
The skin is an incredibly complex organ system responsible for the 3 main functions of protection, thermoregulation, and sensation.
- •
The vascular anatomy of the skin comprises an intricate network of vascular plexuses that are responsible for maintaining the vitality of the cutaneous structures and facilitating the use of local skin flaps in Mohs reconstruction.
- •
Local skin flaps are categorized based on their vascular supply and include random cutaneous flaps, axial or direct cutaneous flaps, and fasciocutaneous flaps.
- •
Significant physiologic changes occur within the skin during flap elevation and transfer, enhancing and promoting flap survival. These changes can be further leveraged using the concepts of vascular delay.
- •
An understanding of the biomechanical properties of nonlinearity, anisotropy, and viscoelasticity is critical in the design and execution of local skin flap reconstruction.
Introduction
The closure of skin defects created by Mohs micrographic surgery routinely requires the use of cutaneous flaps borrowed from the surrounding skin to produce an aesthetically pleasing result. Flap creation results in tissue stresses that can have a significant impact on survival of the flap by potentially compromising the neurovascular supply to the cutaneous structures. With these stresses in mind, cutaneous surgeons must command a full knowledge and understanding of the vascular patterns, physiology, and biomechanics of skin flaps. That a skin flap is able to survive such insults is a direct result of these redundant and complex systems providing vitality and survivability to the flap when correctly designed. A full understanding of the physiology of integumentary structures and the biomechanics involved in tissue transfer results in improved flap survival and outcomes.
Introduction
The closure of skin defects created by Mohs micrographic surgery routinely requires the use of cutaneous flaps borrowed from the surrounding skin to produce an aesthetically pleasing result. Flap creation results in tissue stresses that can have a significant impact on survival of the flap by potentially compromising the neurovascular supply to the cutaneous structures. With these stresses in mind, cutaneous surgeons must command a full knowledge and understanding of the vascular patterns, physiology, and biomechanics of skin flaps. That a skin flap is able to survive such insults is a direct result of these redundant and complex systems providing vitality and survivability to the flap when correctly designed. A full understanding of the physiology of integumentary structures and the biomechanics involved in tissue transfer results in improved flap survival and outcomes.
Vascular anatomy and physiology of the skin
The skin is a complex organ system comprising the epidermis, the dermis, and the subcutaneous tissues. It is the body’s largest organ system, responsible for 3 main functions: sensation, protection, and thermoregulation. The thermoregulatory function and nutritional support of the integument result from the unique configuration and rheology of the blood supply to the skin. Although the sensory and barrier functions of the skin are of obvious and of vital importance, the main focus of this discussion is on the blood supply and thermoregulatory function of the skin.
Vascular Anatomy
The vascular supply to the skin is extraordinarily complex. It receives contributions from a variety of sources, coupled with a dizzying network of anastomosing vessels that forms several extensive and highly interconnected vascular plexuses at differing levels within the architecture of the skin. A fair degree of variance exists in the literature when describing the vascular anatomy, with certain terms used interchangeably by different investigators. To help alleviate any confusion, an attempt is made to simplify the anatomic descriptions and maintain consistency in the application of this terminology.
There are 3 main locoregional conduits of blood flow to the skin: musculocutaneous arteries, direct cutaneous arteries, and septocutaneous arteries. Musculocutaneous arteries are perforators from deeper muscular arteries that pass through their corresponding muscle tissue and enter the subcutaneous fat to ultimately drain into the subdermal and dermal plexuses. Less common than their musculocutaneous counterparts, direct cutaneous arteries are generally specific, named arteries or branches of named arteries that run through the subcutaneous tissues in a plane parallel to the skin. They have been described as confined to specific areas of the body and are generally accompanied by veins known as venae comitantes. Although they may branch off septocutaneous arteries, they are generally named branches of larger arteries. Examples include the parietal and frontal branches of the superficial temporal artery, the posterior auricular artery, the occipital artery, the supratrochlear artery, and the supraorbital artery. Septocutaneous arteries course through the fascial septa dividing the individual muscle segments to provide blood flow to the skin. The fasciocutaneous component of these arteries runs parallel to the skin surface atop and/or deep to the superficial muscular fascia, and they are also generally accompanied by a pair of veins. The direct cutaneous and septocutaneous arteries are responsible for supplying larger, more diffuse regions of skin, whereas the areas supplied by musculocutaneous arteries are generally smaller and more discrete in distribution. All 3 arterial sources drain into components of the rich network of plexuses that exist within the superficial soft tissues. It is particularly relevant that there is often more than one source of blood supply to a particular area of the skin, which contributes significantly to the hardiness of the cutaneous flaps commonly used in facial reconstruction.
As many as 5 different vascular plexuses within the skin and superficial soft tissues have been described in the literature, including the dermal, subdermal, subcutaneous, prefascial, and subfascial networks. The dermal and subdermal networks are sometimes considered a single unit, referred to as the dermal-subdermal plexus. The prefascial and subfascial networks are formed from the fasciocutaneous system off the septocutaneous arteries. The subcutaneous plexus is probably the least consistent and least described of the skin plexuses. Fig. 1 represents the manner in which all these vascular webs are extensively interconnected, creating a vast and elaborate system of collateral blood flow. This rich collateral blood flow, facilitated by the various plexuses, provides an impressive breadth of vascular redundancy to the cutaneous structures. It is this redundancy of flow that provides not only the mechanism for the thermoregulatory function of the skin but also the critical nutrient flow, allowing for cutaneous flap survival and optimal healing. These robust vascular networks form the foundation for the complex circulatory physiology of the skin.

Physiology of the Skin
Due to the important role of the integument in corporeal thermoregulation and homeostasis, the skin has a remarkable hemodynamic ability to vary the rate and quantity of flow through its vascular plexuses. Even under normal circumstances, the blood supply provided to the skin is far greater than that needed for its nutrient support. It has been estimated that the rate of flow through the skin at normal temperatures is approximately 10 times greater than the minimal requirement for adequate tissue nutrition. As the body is heated, cutaneous vasodilatation increases to such an extent that the flow through the cutaneous plexuses can elevate as high as 6 L/min to 8 L/min during periods of hyperthermia. Likewise, hypothermia or extreme local skin cooling can induce a thermoregulatory vasoconstriction in skin that can reduce blood flow rates to levels that are barely adequate to completely inadequate for cutaneous cellular nutrition. These autonomic vasoconstrictive and vasodilatory mechanisms also significantly contribute to reflex control of systemic blood pressure.
The variability of blood flow to and through the skin is the result of arterioles that serve as regulatory sphincters under conditions of adequate systemic pressure, controlling flow through the cutaneous subdermal and dermal networks. The dermal networks are divided into 2 main segments that are responsible for the primary functions of the integumentary circulation. The reticular dermis contains a capillary network that is responsible for nutrient flow to the skin. The arterioles serving as precapillary sphincters control flow through this intricate endothelial web and are chiefly responsive to conditions of hypoxemia and increased metabolic demand, resulting in sphincter dilation to increase local blood flow. Conversely, the papillary dermis contains a network of looping arteriovenous shunts ( Fig. 2 ) that allow for the thermoregulatory and hemodynamic functions of the skin. The arterioles serving as preshunt sphincters respond primarily to local autonomic release of norepinephrine or acetylcholine to either decrease or increase flow through the papillary network in response to the thermal or systemic pressure changes affecting the skin locally or the body globally. Other factors, such as inflammation, cytokines, nitric oxide, free radicals, tissue edema, and toxins, such as tobacco smoke, can also have a profound impact on cutaneous blood flow. Several of these factors are discussed in more detail, as the discussion broadens to include cutaneous flap physiology.

Local cutaneous flaps
Cutaneous flaps of the head and neck region are generally broken down into 2 categories: random pattern flaps and axial pattern flaps. A third category considered is the fasciocutaneous flap, which also has relevant applications in facial reconstruction. Fig. 3 illustrates the anatomic differences between the differing flaps.

Random Flaps
Random pattern flaps, also known as local cutaneous or random cutaneous flaps, are frequently used in reconstruction of facial skin defects. Dissection of these flaps occurs in the subcutaneous tissue plane, and they can be classified into advancement, transposition, and rotation flaps based on their geometric design. The vascular supply to random cutaneous flaps is through musculocutaneous perforators located near the base of the flap. Blood flow to the body, margins, and tip of the flap are supplied via the dermal-subdermal plexus. The maximal survival length of these flaps is reliant on the vascular perfusion pressure. In the past, a length-to-width ratio no greater than 3:1 had been advocated for random cutaneous flaps. This rule of thumb is now generally regarded as inaccurate. The vascular perfusion pressure of a random flap can be unpredictable based on several variables. Ultimately, if the perfusion pressure at any portion of the flap falls below the arteriole closing pressure, the distal soft tissues becomes ischemic and ultimately results in necrosis of that portion of the flap. Recruiting more vessels into the base of the flap does not change the perfusion pressure of those vessels, thus providing no change in the maximal survival length of the flap ( Fig. 4 ).
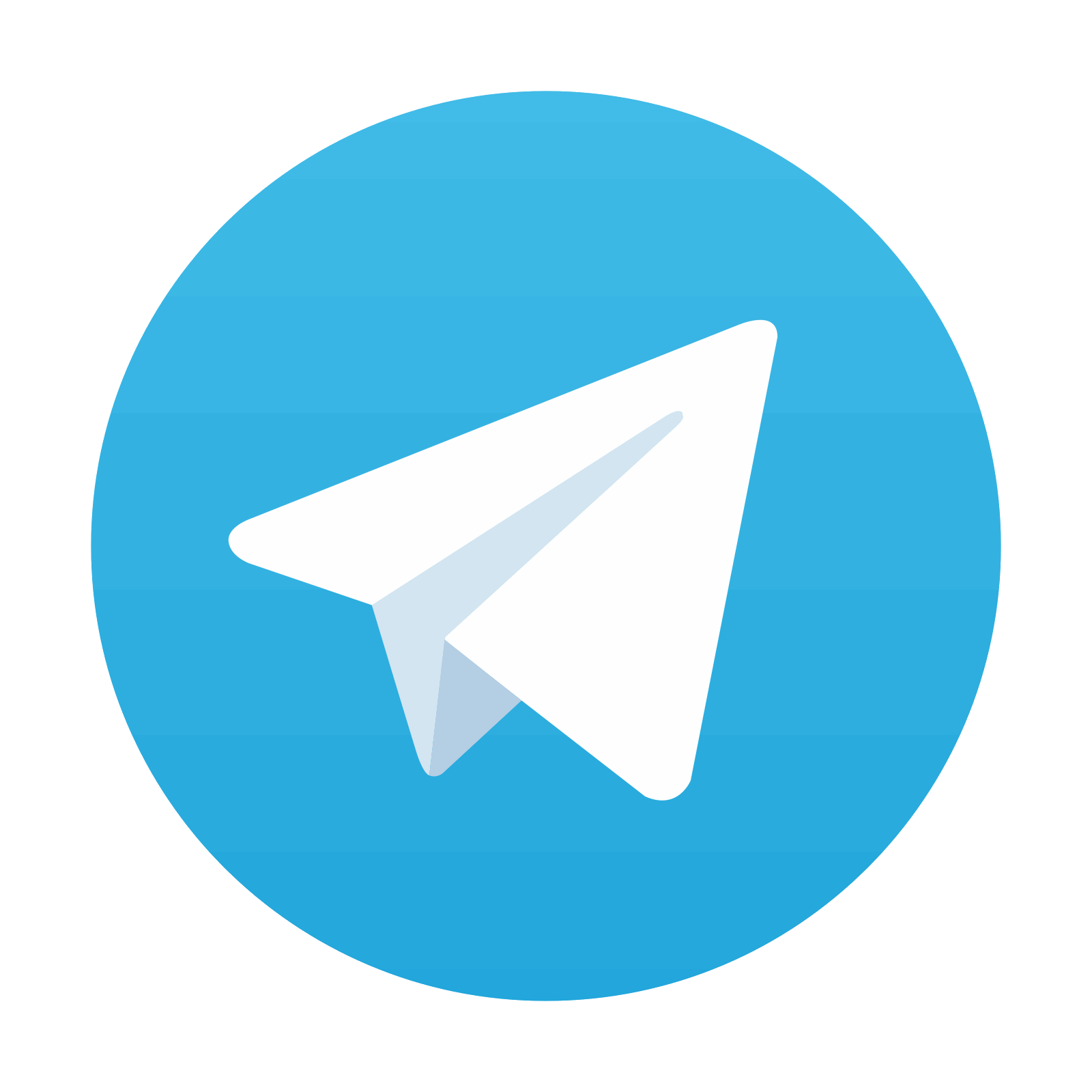
Stay updated, free articles. Join our Telegram channel

Full access? Get Clinical Tree
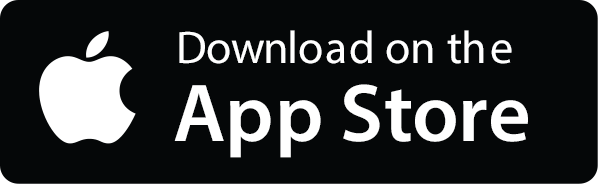
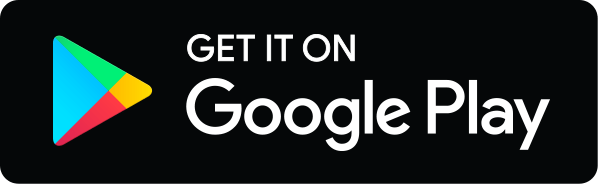
