Abstract
Melanoma is a malignant tumor that arises from melanocytes and is most commonly cutaneous in origin. However, it can also arise on mucosal surfaces or within the eye and leptomeninges. Due to its metastatic potential, melanoma leads to more than 90% of skin cancer-related deaths. The incidence rates of melanoma have increased over the past four decades by five- to seven-fold, whereas mortality rates began to stabilize in the early 1990s, due primarily to the early detection of thinner cutaneous melanomas. In situ and early invasive cutaneous melanoma can be subtle in appearance but dermoscopy has led to an improvement in diagnostic accuracy. Early-stage melanomas are often curable by surgical excision. Sentinel node biopsy provides additional prognostic information and those with resected stage III disease can be offered adjuvant immunotherapy. For metastatic melanoma, oral therapies that target mutant signaling pathways (e.g. BRAF and MEK inhibitors) and immunotherapies that act as checkpoint inhibitors (e.g. anti-CTLA-4, -PD-1, and -PD-L1 antibodies) represent breakthrough treatments.
Keywords
melanoma, cutaneous melanoma, melanoma risk factors, BRAF mutation, BRAF V600E, MAP kinase pathway, MAPK pathway, immune checkpoint inhibitors, melanoma targeted therapy, BRAF inhibitors, MEK inhibitors, melanoma and pregnancy, childhood melanoma, melanoma surveillance, sentinel lymph node biopsy, ipilimumab
- ▪
Melanoma represents a malignant tumor that arises from melanocytes, and, due to its metastatic potential, leads to >90% of skin cancer deaths
- ▪
The incidence rates of melanoma have increased over the past four decades by five- to seven-fold, whereas mortality rates began to stabilize in the early 1990s
- ▪
In situ and early invasive cutaneous melanoma can be subtle in appearance but dermoscopy has led to an improvement in diagnostic accuracy
- ▪
Early-stage melanomas are often curable by surgical excision
- ▪
For metastatic melanoma, oral therapies that target mutant signaling pathways (e.g. BRAF and MEK inhibitors) and immunotherapies that act as checkpoint inhibitors (e.g. anti-CTLA-4, -PD-1, and -PD-L1 antibodies) represent breakthrough treatments
Introduction
Melanoma is a malignant tumor that arises from melanocytes and is most commonly cutaneous in origin. It can also arise on mucosal surfaces (e.g. oral, conjunctival, vaginal) and within the uveal tract of the eye as well as the leptomeninges.
Rising incidence rates of cutaneous melanoma have been observed worldwide over the past four decades in white populations . While mortality rates increased slightly in the US and Europe during the 1970s and 1980s, a stabilization of mortality rates was observed in a number of countries during the 1990s, due primarily to the early detection of thinner cutaneous melanomas. Melanoma is also one of the most common forms of cancer in young adults . It therefore represents a significant public health problem, especially with respect to years of life lost.
Molecular Pathogenesis
Cancer usually develops through a stepwise evolutionary process, with tumor progression involving both genetic instability and selective growth of cells with advantageous mutations. Additional contributing factors include genetic predisposition, mutagenic environmental events, and antitumor host response. There are also several hallmarks of cancer, including self-sufficiency with regard to growth signals, insensitivity to antigrowth signals, evasion of apoptosis, limitless replicative potential, sustained angiogenesis, and tissue invasion and metastasis. As depicted in Figs 107.2 and 107.3 , this set of properties can be acquired by the activation of oncogenes or the inactivation of tumor suppressor genes via molecular mechanisms such as point mutations, deletions, and translocations as well as epigenetic mechanisms such as microRNA expression and promoter methylation. Genome-wide analysis of genetic aberrations has revolutionized our understanding of the complex interplay of signaling pathways .
In melanoma, initiating oncogenic events frequently affect genes involved in the mitogen-activated protein kinase (MAPK) and phosphoinositide 3-kinase (PI3K) signaling pathways such as BRAF , NRAS , and KIT ( Figs 113.1 & 113.2 ). While these alterations may initially result in senescence, secondary genetic alterations, including amplification of CCND1 which encodes cyclin D1, deletion or mutation of CDKN2A which encodes both p16 and p14 ARF , and/or mutation of TP53 which encodes p53, result in progression into a malignant tumor . Early on in tumorigenesis, genetic instability predominates (e.g. via telomere attrition), but with time melanomas regain telomerase expression and as a result acquire unlimited replicative potential .


Nowadays, melanomas can be classified based upon molecular findings , providing a rationale for targeted therapy (see below). As depicted in Fig. 113.1 , melanomas from different body sites and from locations with different amounts of ultraviolet radiation (UVR) damage also differ with regard to frequencies of mutated oncogenes. For example, when melanomas arise within intermittently sun-exposed skin that does not have chronic sun-induced damage histologically, they are more likely to have BRAF mutations; these melanomas also carry a lower mutation burden and contain less UV-signature mutations. In comparison, melanomas from chronically sun-exposed skin are less likely to have BRAF mutations and contain a higher overall mutation burden as well as a higher percentage of UV-signature mutations . These findings may be explained in part by direct induction of pyrimidine dimers by UVB in the latter group versus indirect mutagenesis by UVA and formation of free radicals in intermittently sun-exposed skin . Patterns of changes in DNA copy number also vary in different body sites.
Cellular Signaling Pathways in Melanoma
Genetic aberrations in melanoma frequently affect signaling pathways that play an essential role in normal melanocyte biology. As noted previously, discovery of specific sites of dysfunction within these pathways has led to the era of targeted therapies, e.g. the use of vemurafenib in patients with a somatic mutation in BRAF that leads to substitution of glutamic acid (E) for valine (V) at codon 600 (i.e. V600E) and subsequent activation of the mitogen-activated protein kinase (MAPK) pathway.
MAPK signaling
The MAPK pathway regulates cellular proliferation, growth, and migration. Via this pathway, interactions between growth factors (mitogens) and receptor tyrosine kinases (RTKs) such as the KIT receptor on the cell surface eventually lead to changes in the activity of transcription factors and gene expression within the nucleus (see Fig. 113.2 ). Upon ligand binding, RTKs dimerize and become activated by autophosphorylation of intracellular tyrosine residues . The phosphorylation of tyrosine residues creates binding sites for adaptor proteins (e.g. GRB2, SOS) and initiates a signaling cascade that requires the GTPase activity of NRAS and the kinase activity of BRAF, MEK, and ERK. Phosphorylated ERK activates nuclear cyclin D1 and a complex between cyclin D1 and cyclin-dependent kinases 4 and 6 (CDK4/6) is formed that phosphorylates retinoblastoma tumor suppressor protein (Rb), resulting in release of E2F from the Rb–E2F complex. E2F represents a family of transcription factors that play an important role in the regulation of cell cycle progression (see Fig. 107.1 ). An important tumor suppressor is p16, encoded by CDKN2A . By binding to CDK4/6, p16 prevents the formation of the cyclin D1–CDK4/6 complex and as a result prevents Rb activation and E2F release.
The key role of the MAPK pathway in melanoma is highlighted by the high frequency of mutations in the genes that encode its components, in particular BRAF and NRAS . With regard to RTKs, 30% to 40% of acral and mucosal melanomas as well as melanomas from chronically sun-exposed skin harbor activating mutations or copy number amplifications of KIT . In addition, constitutively activating mutations are present in NRAS (15–20% of melanomas overall) and BRAF (50–60%) (see Fig. 113.1 ). Of note, MAPK pathway activation in melanoma can also be induced by inherited mutations in CDKN2A which underlie familial melanoma , as well as by acquired inactivating mutations and deletions of CDKN2A .
PI3K signaling
Phosphoinositide 3-kinases (PI3Ks) are enzymes that regulate cell growth, proliferation, differentiation, motility, and survival (see Fig. 113.2 ). PI3Ks are activated by RTKs and phosphorylate PIP2 (phosphatidylinositol 4,5-bisphosphate) to PIP3 (phosphatidylinositol 3,4,5-triphosphate). PIP3 then acts as a second messenger and AKT is activated via phosphorylation. It is important to note that by converting PIP3 back to PIP2, AKT activation can be inhibited by PTEN . Activated phospho-AKT inhibits apoptosis by phosphorylating BAD which leads to its loss of pro-apoptotic function, enhances survival by increasing the transcription of survival genes, and accelerates cell growth via mTOR (see Fig. 113.2 ).
PI3K signaling is activated in a high percentage of melanomas by multiple mechanisms. Frequent activating events include inactivation of the gene that encodes the inhibitor PTEN via mutations, deletions or promoter methylation , activating mutations of NRAS , and overexpression of AKT . However, direct activation of the PI3K pathway by activating mutations in the genes that encode PI3K subunits occurs infrequently .
WNT signaling
As a group, WNT signaling proteins are involved in cellular processes such as differentiation, migration, proliferation, and stem cell maintenance (see Fig. 55.6 ). The role of WNT signaling in melanoma is complex, as WNT signaling is tightly regulated. In melanoma, mutations that stabilize β-catenin and increase intracellular β-catenin have been described. However, β-catenin and APC mutations are rare events in melanoma . The controversial role of β-catenin signaling in melanoma is highlighted by the observation that gene silencing of β-catenin in melanoma cells slows their growth but promotes the formation of pulmonary metastases in mice . In sum, WNT signaling can promote tumor growth by activating proliferation and cell migration, but it can also inhibit tumor growth by inducing cell differentiation and acting as a tumor suppressor .
MC1R–MITF signaling
The melanocortin 1 receptor (MC1R) is a G-protein-coupled receptor that is activated by melanocortins (ACTH, α-MSH; see Table 65.4 ). MC1R is one of the key receptors involved in regulating skin and hair color . When bound to its ligands, MC1R activates adenylate cyclase and cAMP is formed as a second messenger (see Fig. 65.4 ). cAMP activates protein kinase A (PKA) and PKA activates the cAMP response-element binding protein (CREB); the latter is a transcription factor that enhances expression of microphthalmia-associated transcription factor (MITF) . MITF is also a transcription factor and it regulates expression of a number of melanocyte lineage-specific genes that encode enzymes of the melanin biosynthetic pathway (e.g. tyrosinase, tyrosinase-related proteins) as well as other genes such as CDK2 , CDKN2A , and BCL2 . MITF signaling is also tightly linked and regulated by MAPK and WNT signaling (see Fig. 65.4 ) .
MCIR variants have been associated with a red hair/fair skin phenotype, and independent of phenotype, have been found to be a risk factor for the development of cutaneous melanoma (see Fig. 65.14 ) . In addition, MITF has been shown to be an oncogene amplified in melanoma . However, MITF can also act as an antiproliferative transcription factor by inducing cell cycle arrest. Interestingly, mutant oncogenic BRAF can regulate MITF expression to ensure that its protein levels are compatible with proliferation and survival of melanoma cells .
Better understanding of the molecular mechanisms of melanoma development will hopefully translate into improvements in melanoma risk assessment, prevention, diagnosis, and molecularly targeted therapies.
Host Immune Response to Melanoma
Clinical observations such as incomplete or complete regression of melanoma ( Fig. 113.3 ), associated development of vitiligo-like depigmentation and halo nevi, and a higher incidence of melanoma in immunosuppressed patients point to the fact that melanoma is an immunogenic tumor. Numerous melanoma antigens recognized by autologous T cells or antibodies have been described including: (1) mutant tumor antigens (e.g. mutant p16 [ CDKN2A ]); (2) shared tumor-specific antigens of the cancer/testis family (e.g. MAGE-1, MAGE-3, NY-ESO-1); and (3) cell type-specific differentiation antigens (e.g. tyrosinase, PMEL17/gp100, MART-1/Melan-A). These proteins are processed and presented on the melanoma cell surface as MHC/peptide complexes. CD8 + cytotoxic T cells recognize these antigens and, if appropriately activated, are able to kill tumor cells. In addition to CD8 + T cells, CD4 + helper T cells as well as antibodies also play a critical role.

Activation of melanoma-specific CD8 + T cells is dependent on the migration of tumor antigen-loaded dendritic cells to a draining lymph node. Here, melanoma antigens are presented to CD8 + T cells in the presence of a costimulatory signal provided by the binding of B7 on the antigen-presenting cell to CD28 on the T cell (see Ch. 4 ). Cytotoxic T lymphocyte-associated antigen-4 (CTLA-4) is an inhibitory homolog of CD28 that is upregulated following T-cell activation and acts as natural inhibitor of T-cell activation by removing the costimulatory signal. Programmed cell death protein 1 (PD-1) is another immuno-inhibitory receptor in the CD28 family ( Fig. 128.9 ). The interaction of PD-1 with its ligands PD-L1 and PD-L2 promotes apoptosis of tumor antigen-specific T cells and reduces apoptosis in regulatory T cells. Recently, immunotherapy based upon CTLA-4, PD-1, and PD-L1 blockade has been introduced for the treatment of melanoma (see below).
A variety of immune escape mechanisms may be found in advanced tumors, including melanoma. These include loss of tumor-specific antigens, loss of MHC class I molecules, and secretion of immuno-inhibitory cytokines such as interleukin (IL)-10 and TGF-β. Down-regulation of a melanoma-specific immune response might also involve naturally occurring CD4 + /CD25 + regulatory T cells, inducible IL-10-producing regulatory T cells, negative signals delivered via cell surface CTLA-4, or aberrant expression of PD-L1 . Insights into mechanisms of melanoma-specific host immune responses and immune escape have led to improved immunotherapy in the form of checkpoint inhibitors and hopefully will lead to other more effective therapies, including vaccines.
Epidemiology
Over the past several decades, the incidence rates of cutaneous melanoma have increased significantly ( Fig. 113.4A ). It develops primarily in Caucasian populations, with its incidence remaining very low amongst darkly pigmented populations of African, Asian, and Hispanic origin .

The annual increase in melanoma incidence varies depending upon the population, but in general it has been estimated to be between 3% and 7%. These estimates predict a doubling of rates every 10–20 years, making cutaneous melanoma the most rapidly increasing cancer in white populations. For example, in the state of Connecticut, over a period of six decades (1950 to 2007), the overall incidence rate rose greater than 17-fold in men (1.9 to 33.5 per 100 000) and more than nine-fold in women (2.6 to 25.3 per 100 000) . Cohort studies from several countries indicate that the trend of increasing incidence rates will continue for at least the next two decades.
Based upon data from the Surveillance Epidemiology and End Results (SEER) database, an estimated 76 380 men and women in the US were expected to be diagnosed with melanoma in 2016 and an estimated 10 130 were expected to die from melanoma that same year ( seer.cancer.gov ). In Europe, the highest incidence rates have been noted in Scandinavian countries, with the lowest incidence rates observed in Mediterranean countries. One explanation for this North–South gradient is a darker skin phototype (types III–IV) in Mediterranean populations. Worldwide, the highest incidence rates have been reported in Australia and New Zealand, with 39 (women) to 60 (men) cases per 100 000 inhabitants per year in Australia in 2016. Within Australia, the highest incidence rates have been found in northern equatorial regions such as Queensland .
Mortality rates from melanoma increased throughout the 1980s in most European countries, as well as in North America ( Fig. 113.4B ), Australia and New Zealand, then peaked in the early 1990s. Subsequently, trends have been less uniform, with mortality rates still rising in several European countries among middle-aged adults, but with more favorable trends in women and some stabilization in young adults; rates have remained relatively constant among men .
Vertical tumor thickness (Breslow depth) is the most important local prognostic factor in primary cutaneous melanoma. One explanation for the progressive reduction in mean tumor thickness that has occurred over the past several decades is more effective detection of melanoma at an earlier stage . In Germany, there has been a trend towards diagnosing thinner melanomas since the 1980s, with the median tumor thickness decreasing from 1.81 mm (1980s) to 0.53 mm (2000). The percentages of in situ and Clark level II melanomas also increased . Diagnosis of thinner tumors should translate into stable or decreasing mortality rates, despite the increase in incidence rates.
The tumor thickness at the time of primary diagnosis is also age-dependent . In general, there are significantly fewer melanomas ≤1.0 mm in older age groups. As a corollary, the percentage of thick melanomas increases significantly with age and reaches 20% by age 80 years in both genders .
Risk Factors for Cutaneous Melanoma
Recognition of risk factors for the development of melanoma is important from a public health and a clinical care perspective ( Table 113.1 ). Identification of high-risk cohorts improves the efficiency and efficacy of public health efforts. Individual risk assessment influences clinical decision-making including the threshold for performing a biopsy, prevention counseling, and surveillance. Risk factors may be divided into three categories: (1) genetic factors; (2) phenotypic manifestations of gene–environment interactions; and (3) environmental factors.
RISK FACTORS FOR THE DEVELOPMENT OF CUTANEOUS MELANOMA |
Genetic factors |
|
Environmental factors |
|
Phenotypic expressions of gene/environment interactions |
|
Genetic Risk Factors
Germline genetic mutations and polymorphisms can predispose individuals to melanoma. The genes involved range from the rare high-penetrance genes responsible for some familial clustering of melanoma to the very common pigmentation genes responsible for the relative propensity for fair-skinned individuals to develop melanoma .
The major high-penetrance susceptibility gene locus associated with familial melanoma is CDKN2A . Approximately 2% of cutaneous melanomas can be specifically attributed to pathogenic germline mutations in CDKN2A . This locus encodes two distinct protein products, p16 and p14 ARF , with the latter via an alternative reading frame, hence the designation ARF. p16 and p14 ARF exert regulatory effects on cell cycle progression through the retinoblastoma protein (Rb) and p53 pathways, respectively (see Fig. 107.1 ) . Germline CDKN2A mutations are observed in ~20% of familial melanoma kindreds ( Fig. 113.5 ). The penetrance of CDKN2A mutations is modified by germline variants of other genes, including MC1R . In some families, carriers of germline CDKN2A mutations are also at increased risk of developing pancreatic cancer .

While assays for these CDKN2A mutations have been commercialized and have been advocated by some in the field, it is the recommendation of the International Melanoma Genetics Consortium that at present genetic testing for CDKN2A mutations be done only as part of research protocols . However, other experts have suggested that individuals with three or more primary invasive melanomas or families with at least one invasive melanoma and two or more other diagnoses of invasive melanoma or pancreatic cancer among first- or second-degree relatives (on the same side of the family) were appropriate candidates for referral for genetic consultation and possible testing . Fig. 113.5 depicts the less common genes that when mutated can also lead to familial melanoma (e.g. CDK4 , BAP1 ). It also emphasizes the high percentage of families for whom the genetic basis is unknown, a point that should be discussed with patients contemplating genetic screening .
Among pigmentation genes associated with an increased risk for developing melanoma, specific germline variants of the melanocortin 1 receptor ( MC1R ) gene have been found to correlate with melanoma risk above and beyond clinically apparent skin phenotype . A strong association between MC1R as a susceptibility gene and the risk of developing BRAF -mutant melanomas has also been observed. While the risk of developing any cutaneous melanoma lies between 1.5-fold and 3-fold for individuals with MC1R variants, the risk of developing BRAF -mutant melanomas was as high as 17-fold for those with two MC1R germline variants . In addition to MC1R , single nucleotide polymorphisms in the tyrosinase ( TYR ) gene, the tyrosinase-related protein 1 ( TYRP1 ) gene, and the SLC45A2 gene are significantly associated with melanoma risk (see Ch. 65 ) .
Phenotypic Risk Factors Reflecting Gene/Environment Interactions
The strongest independent risk factors for the development of cutaneous melanoma are those that reflect a combination of genetic susceptibility and environmental exposure: melanocytic nevi, atypical melanocytic nevi, ephelides, and solar lentigines. Both an increased number of common melanocytic nevi and the presence of atypical melanocytic nevi are independent risk factors for melanoma, as is the presence of solar lentigines. Acquired melanocytic nevi play a dual role as a risk factor: (1) they are indicators of UV exposure and DNA damage; and (2) they can be formal precursors of melanoma. However, remnants of benign melanocytic nevi are found in only a minority of melanomas . Based on epidemiologic data, the risk of malignant transformation in an individual melanocytic nevus has been estimated to be one melanoma in hundreds of atypical nevi and one in thousands of common nevi . Consequently, prophylactic removal of clinically unsuspicious melanocytic nevi is not justified.
Common melanocytic nevi
Although performed on populations from different regions and with varying skin phototypes, studies have consistently reported a continuous and almost linear increase in melanoma risk with greater numbers of common melanocytic nevi ( Fig. 113.6 ). Also, light-skinned populations have higher melanocytic nevus counts than do darker-skinned populations. For those with >50 common melanocytic nevi, the relative melanoma risk has ranged from 6.9 in southern Spain to 53.9 in Scotland . Nonetheless, the dose-dependent relationship between number of common melanocytic nevi and risk of cutaneous melanoma holds true for all Caucasian populations.

The association of increased nevus counts and melanoma risk is strongest for superficial spreading melanoma and nodular melanoma, in contrast to lentigo maligna melanoma which is highly correlated with skin type and hair color . This suggests there are additional genetic susceptibility factors and molecular mechanisms involved in the formation of melanoma in chronically versus intermittently sun-exposed skin (see Fig. 113.1 ). Melanoma at a particular anatomic site is more strongly related to the number of melanocytic nevi within that respective site than to the number of nevi in other body sites , highlighting the key role of local mutagenic effects of UV radiation in addition to genetic susceptibility.
Atypical melanocytic nevi
Since the original description of BK-mole syndrome in 1978 , several definitions of atypical melanocytic nevi as well as the atypical mole syndrome have been published. In general, a diagnosis of atypical melanocytic nevi is based upon the presence of at least three of the following criteria: (1) diameter ≥5 mm; (2) ill-defined borders; (3) an irregular margin; (4) varying shades within the lesion; and (5) the simultaneous presence of papular and macular components . A relative risk of up to 500-fold for developing cutaneous melanoma has been described for specific subgroups of the atypical mole syndrome . However, sporadic atypical melanocytic nevi are often diagnosed in “normal” individuals outside of the setting of the atypical mole syndrome. One possible explanation for the range of estimates of risk is the use of different definitions of clinically “atypical” melanocytic nevi by various investigators.
Several studies have found clinically atypical nevi to be associated with increased melanoma risk outside of the context of familial melanoma ( Fig. 113.7 ). The maximum reported relative risk for developing melanoma was as high as 32-fold when there were 10 or more atypical melanocytic nevi . The consistency of these findings among multiple studies and across geographic locations supports the significance of atypical melanocytic nevi as an independent risk marker for sporadic melanoma.

In contrast to the continuous increase in melanoma risk with greater numbers of common melanocytic nevi, for atypical melanocytic nevi a threshold level of five or more such lesions was associated with a clearly higher relative risk in several studies; however, there was no significant increase in the risk beyond this number . This finding suggests that at least five atypical melanocytic nevi are needed to establish the diagnosis of atypical mole syndrome. In patients with familial melanoma, atypical melanocytic nevi are an indicator of a markedly elevated melanoma risk .
Ephelides and solar lentigines
Ephelides (freckles) have been reported to be a risk factor independent of numbers of common melanocytic nevi. The independence of freckling and common melanocytic nevi indicates that these cutaneous findings represent separate host characteristics, both of which are related to sun exposure . Additional studies found that solar lentigines were a risk factor for the development of cutaneous melanoma, independent of the number of common melanocytic nevi .
Environmental Risk Factors
Ultraviolet radiation
It is widely believed that the total risk for developing melanoma is determined by the interplay between genetic factors and exposure to UVR. Approximately 80% of cutaneous melanomas develop in intermittently sun-exposed regions, and both intermittent sun exposure and sunburn history have been identified as risk factors in epidemiologic studies .
The role of sunlight in melanoma development has been a matter of debate for decades, in part because the etiologic role of sunlight is far less clear than in other tumors such as cutaneous squamous cell carcinoma (SCC) . Also, melanoma is clearly a multi-factorial disease and forcing an all-or-none stance regarding sun exposure represents an erroneous oversimplification. That said, the following clinical and epidemiologic features have raised doubts regarding the impact of sunlight on melanoma development:
- •
With the exception of lentigo maligna melanoma, the anatomic distribution of melanoma does not closely match sites of greatest cumulative sun exposure as in cutaneous SCC.
- •
Melanoma most commonly develops in middle-aged adults, not the elderly with the greatest cumulative sun exposure.
- •
In most of the reported case–control studies, neither greater cumulative sun exposure during adulthood nor sunburns during the preceding years were associated with an elevation in melanoma risk.
The total number of melanocytic nevi has been identified as the most important risk factor for cutaneous melanoma, and as the number of melanocytic nevi increases, the melanoma risk increases nearly linearly (see Fig. 113.6 ). Therefore, to gain more insight into the relationship between sun exposure and nevus development, epidemiologic studies have been performed in young children. With exposure to intense UVR, children in Australia developed melanocytic nevi early in life and in large numbers ; of note, duration of sun exposure was a stronger risk factor than the occurrence of sunburns. In a study of 1812 German nursery school children, high nevus counts in children were preferentially associated with the number of weeks on sunny holidays and with outdoor activities at home that were linked to moderate sun exposure . These findings suggested that sunburns were not required and that moderate sun exposure appeared to be sufficient for the induction of melanocytic nevi in children.
A possible explanation for the differences in the epidemiology of melanoma as compared with non-melanoma skin cancer involves apoptosis . After exposure to UVR, the most severely damaged keratinocytes undergo apoptosis (sunburn cells), with the less damaged keratinocytes upregulating their DNA-repair capacities and undergoing nearly perfect DNA repair. As a result, the development of non-melanoma skin cancer would favor elderly adults. In contrast, melanocytes do not undergo apoptosis as readily. Their function is to provide protective melanin to the surviving cells and so they are retained within the epidermis, even with damage. In addition, some UVR-induced mutations are thought to enable melanocytes to proliferate and give rise to junctional nevi. Subsequently, melanoma could arise in anatomic sites with intermittent sun exposure, with the sites of melanoma development reflecting the distribution pattern of melanocytic nevi . This hypothesis might also explain why melanomas tend to develop in a younger age group (i.e. middle-aged adults).
Both UVB and UVA radiation are thought to be associated with the development of cutaneous melanoma, with UVB believed to be the stronger risk factor. Data supporting UVB as a causative factor come from: (1) animal studies in which UVB was shown to induce melanoma in susceptible mice and in xenografted human skin engineered to express melanocytic growth factors ; and (2) the fact that there is a higher incidence of cutaneous melanoma in equatorial regions where UVB is most intense. Data implicating UVA in the development of cutaneous melanoma derive from: (1) animal studies in which UVA was shown to initiate melanoma (fish) and melanocytic hyperplasia (pigmented opossums) ; and (2) epidemiological studies showing an increased incidence of melanoma developing in users of tanning beds and perhaps recipients of PUVA. In 2009, the World Health Organization (WHO) International Agency for Research on Cancer (IARC) categorized tanning beds as a human carcinogen. Of note, exposure to tanning beds before the age of 35 years has a statistically significant association with melanoma development.
Sun protection
With regard to sun protection, there is a significant misunderstanding by the public that avoidance of sunburns and use of sunscreens is sufficient to prevent skin cancer. It is well established that suberythemal doses of UVR cause DNA mutations. Chronic irradiation with 10–20% of the minimal erythema dose of UVB induced DNA damage in mouse skin and this damage persisted in the dermis and epidermis for several weeks following discontinuation . Therefore, low-dose UVB exposure in a repeated fashion could cause skin cancer.
Regular use of sunscreen has been shown to decrease the development of actinic keratoses and cutaneous SCCs, but evidence regarding its ability to prevent cutaneous melanoma has been controversial to non-existent, until the past decade (see Ch. 132 ). In 2010, a randomized sunscreen trial in Australia found that the regular application of SPF 15+ sunscreen in 1621 adults over 5 years reduced the incidence of new primary cutaneous melanoma, even 10 years after completion of the trial. Whether or not the regular use of sunscreen in children, when sun exposure is thought to be critical for the development of cutaneous melanoma, will be beneficial is less clear .
There are additional sun-protective measures that should be encouraged, including physical means of blocking the sun (e.g. non-transparent clothing, hats) and reducing UVR exposure (e.g. shade coverings, limiting outdoor activities between the hours of 10:00 AM and 4:00 PM).
Types of Primary Melanomas
Until the 1960s, melanoma was regarded as a large black nodule that, as a rule, metastasized and caused death. Therefore, the recognition of early-stage melanoma has been a major advancement. Four major subtypes (growth patterns) of primary cutaneous melanoma have been described ( Table 113.2 ). These different growth patterns characterize the early phase of development, but do not predict the prognosis.
DIFFERENT TYPES OF PRIMARY CUTANEOUS MELANOMA | |||
---|---|---|---|
Clinico-histopathologic subtype | Abbreviation | Percentage | Median age |
Superficial spreading melanoma | SSM | 57.4% | 51 years |
Nodular melanoma | NM | 21.4% | 56 years |
Lentigo maligna melanoma | LMM | 8.8% | 68 years |
Acral lentiginous melanoma | ALM | 4% | 63 years |
Unclassifiable melanoma | UCM | 3.5% | 54 years |
Others | 5% | 54 years |
Superficial Spreading Melanoma
Superficial spreading melanoma (SSM) is the most common type of cutaneous melanoma in fair-skinned individuals ( Fig. 113.8 ) and it is diagnosed most frequently between the ages of 40 and 60 years. It accounts for ~60–70% of all melanomas and occurs at any site, but is most frequently seen on the trunk of men and the legs of women. It begins as an asymptomatic brown to black macule with color variations and irregular, notched borders ( Fig. 113.9 ). SSM can arise de novo or in a pre-existing nevus ( Fig. 113.10 ). When in situ , the melanoma is usually macular with an irregular outline and variable pigmentation ( Fig. 113.11 ). SSMs may have a diameter ≤5 mm ( Fig. 113.12 ). After a typically slow horizontal (radial) growth phase limited to the epidermis or focally within the papillary dermis, a more rapid vertically oriented growth phase, which presents clinically with the development of a papule or nodule, can then occur. In up to two-thirds of tumors, regression (visible as gray, hypo- or depigmentation) of part of the lesion is observed, reflecting the interaction of the host immune system with the progressing tumor. About half of SSMs arise in a pre-existing nevus , although the likelihood of an individual nevus progressing to melanoma is exceedingly low (see above).





Nodular Melanoma
Nodular melanoma is the second most common type of cutaneous melanoma in fair-skinned individuals and is diagnosed most frequently in patients in their sixth decade of life. It accounts for ~15% to 30% of all melanomas and occurs at any body site, but is most frequently seen on the trunk, head and neck. Nodular melanomas are observed more frequently in men than in women. They usually present as a blue to black, but sometimes pink to red, nodule which may be ulcerated or bleeding and in some patients have developed rapidly over months ( Fig. 113.13 ). Although nodular melanomas are rare in prepubertal children, they are often amelanotic. Nodular melanoma is believed to arise as a de novo vertical growth phase tumor without the pre-existing horizontal growth phase that characterizes the other histologic types. These melanomas tend to be diagnosed at a thicker, more advanced stage, with an associated poorer prognosis. The incidence of nodular melanoma has remained fairly stable in recent decades .

Lentigo Maligna Melanoma
Lentigo maligna melanoma (LMM) represents a minority of cutaneous melanomas (~10%). Although it is diagnosed most frequently during the seventh decade of life, it often occurs in younger patients who have had significant cumulative sun exposure. LMM develops within chronically sun-damaged skin, most commonly on the face, with a preference for the nose and cheek. LMM usually appears as a slowly growing, asymmetric, brown to black macule with color variation and an irregular, indented border ( Fig. 113.14 ). Invasive LMM arises in a precursor lesion termed lentigo maligna ( in situ melanoma in sun-damaged skin; Fig. 113.15A,C ). It has been estimated that 5% of lentigo maligna lesions progress to invasive melanoma . Because lentigo maligna typically arises within a background of severely sun-damaged skin, it can be difficult to histologically distinguish lentigo maligna from the atypical melanocytic hyperplasia seen in severely sun-damaged skin (see Fig. 150.6 ).


Lentigo maligna displays characteristic features on dermoscopic examination ( Fig. 113.15B,D ). In facial skin, hyperpigmented follicular openings (circle in a circle) are slowly overgrown by irregular pigmented dots around the follicles (annular granular pattern) . Rhomboidal structures can also be seen (see Table 0.15 ).
Acral Lentiginous Melanoma
Acral lentiginous melanoma (ALM) is a relatively uncommon type of cutaneous melanoma and is diagnosed most frequently in the seventh decade of life. It typically occurs on the palms and soles or in and around the nail apparatus. ALM accounts for ~5% of all melanomas and the incidence of ALM is similar across all racial and ethnic groups. As more darkly pigmented Africans and Asians do not typically develop sun-related melanomas, ALM represents a disproportionate percentage of melanomas diagnosed in blacks (up to 70%) and Asians (up to 45%) .
ALM typically presents as an asymmetric, brown to black macule with color variation and irregular borders ( Fig. 113.16 ). A disproportionate percentage of ALMs are diagnosed at an advanced stage. This is likely the result of the difficulty of clinically distinguishing many ALMs from benign lesions and traumatic skin changes, as well as an elevated threshold for biopsy due to the morbidity associated with surgery at acral sites. Primary misdiagnosis of ALM occurs in about one-third of all patients and leads to substantial delay in establishing the correct diagnosis .

Melanoma of the nail matrix can present as longitudinal melanonychia ( Fig. 113.17 ) or as hyperpigmentation extending onto the hyponychium or beyond the lateral or proximal nail fold (Hutchinson sign) (see Ch. 71 ). The possibility of melanoma should be considered for all pigmented nail bands in fair-skinned individuals, especially if they are darkly pigmented, have irregular pigmentation and/or have a width ≥3 mm.

ALM is a genetically distinct subtype of melanoma and clearly differs from the other types of cutaneous melanoma. It harbors more and different genetic changes than the other types and can display activating KIT mutations in exons 11, 13 and 17, which can make the tumor sensitive to KIT-inhibiting drugs (e.g. imatinib; see Figs 113.1 & 113.2 ) .
Other Melanoma Variants
There are cutaneous melanomas that may present with unusual manifestations. Some of these are defined by clinical features, others by histologic findings.
Amelanotic Melanomas
Fortunately, the overwhelming majority of melanomas are pigmented, aiding visual diagnosis. Melanomas lacking clinically evident pigment are termed “amelanotic” ( Fig. 113.18 ). All four histologic subtypes of melanoma discussed above can occur as amelanotic variants that largely defy clinical diagnosis. Amelanotic SSMs, nodular melanomas, and LMMs are often biopsied due to clinical suspicion of basal cell carcinoma. Amelanotic ALMs are especially challenging and may be mistaken for warts or SCC. Amelanotic melanomas do not differ from pigmented melanomas in terms of prognosis or therapy.

Melanoma With Features of a Spitz Nevus (“Spitzoid” Melanoma)
This variant shows histologic features suggestive of Spitz nevus with overall symmetry and a dermal nodule of epithelioid melanocytes that do not mature with progressively deeper dermal extension. Other important histologic clues that point to the diagnosis of melanoma are sheets of atypical melanocytes in the dermis and mitotic figures at the base of the lesion (see Table 112.7 ). While several approaches have been taken to distinguish spitzoid melanomas from benign Spitz nevi (e.g. immunohistochemistry, fluorescence in situ hybridization [FISH], comparative genomic hybridization [CGH] analysis), some of these lesions continue to defy definitive histologic diagnosis .
Desmoplastic Melanoma
While this type of melanoma is histologically defined, the typical clinical lesion consists of a skin-colored, red or brown–black nodule or plaque, usually in sun-exposed sites. It may develop de novo , but more commonly arises in conjunction with a lentigo maligna, ALM, or mucosal radial growth phase melanoma. Deep tissue samples are necessary to establish the diagnosis ( Fig. 113.19 ), as superficial portions of the tumor show subtle or non-diagnostic findings which may be mistaken for the fibrosis of a scar or other spindle cell neoplasms. Metastasis to lymph nodes is uncommon, but the tumor is highly infiltrative and, thus, locally aggressive, with recurrence being common after incomplete excision. While these tumors do have significant potential for distant metastasis, conventional T staging tends to overestimate the likelihood for metastasis since these tumors are typically quite deep at the time of diagnosis .

Clear Cell Sarcoma: Melanoma of Soft Parts
Clear cell sarcoma most often presents on the distal extremities of adolescents and young adults. The tumors usually arise in association with tendons and aponeuroses, and they are composed of nests and fascicles of oval to spindled cells with vesicular nuclei, basophilic nucleoli, and eosinophilic to clear cytoplasm. Multinucleated giant cells and melanin can frequently be seen. Despite its unique clinical and histologic presentation, several lines of evidence, including immunohistochemical studies, presence of melanosomes on electron microscopy and gene profiling, support the classification of clear cell sarcoma as a subset of melanoma . Clear cell sarcoma pathogenesis differs from that of other melanoma subtypes by a frequent and characteristic reciprocal translocation t(12; 22)(q13; q12) that results in fusion of EWS and ATF1 genes . The clinical course of these lesions resembles that of other melanomas, with a high likelihood of regional and distant metastasis.
Malignant Blue Nevus
Malignant blue nevus is a rare dermal tumor of melanocytes, most commonly located on the head and particularly the scalp ( Fig. 113.20 ). It appears as a blue–black, deeply situated nodule, generally >1 cm in diameter. Histologically, elements of a benign cellular blue nevus are associated with nodular areas of atypical spindle-shaped and bipolar dendritic melanocytes, mitotic figures, necrosis and melanophages. The clinical course is characterized by a high rate of recurrence and metastasis. The associated cellular blue nevus may have mutations in GNAQ or less often GNA11 (see Table 112.3 ), with mutations in BAP1 , SF3B1 , and EIFA1X detectable within the malignant portion.

Ocular Melanoma
Primary ocular melanomas, which are relatively rare (5% of all melanomas), can be divided into conjunctival melanomas and uveal melanomas (iris, choroidal, and ciliary body melanomas). Uveal melanomas frequently (up to 85%) harbor somatic activating mutations in GNA11 or GNAQ . These genes encode members of the q class of G protein α-subunits. Heterotrimeric G proteins are involved in mediating signals between G-protein-coupled receptors (GPCRs) and downstream effectors (see Fig. 65.15 ) . As noted previously, GNAQ (more frequently than GNA11 ) mutations have been detected in blue nevi but not in cutaneous melanomas. Chromosomal changes of prognostic significance in ocular melanoma have been described and include monosomy 3 and alterations of 8q and 6p. Monosomy 3 reduces the 5-year survival from ~100% to <50%, and a particularly poor outcome has been associated with combined abnormalities in chromosomes 3 and 8 . However, chromosomal studies of fine needle biopsies can be limited due to sampling error resulting from tumor heterogeneity and technical failures.
Nowadays, uveal melanomas are divided into Class 1 (low metastatic risk) and Class 2 (high metastatic risk) based upon a 15-gene expression profile (GEP) . The GEP of Class I uveal melanomas resembles normal uveal melanocytes with >95% of patients free of metastasis at 4 years. In contrast, the GEP of Class 2 uveal melanomas resembles primitive neural/ectodermal stem cells with <20% of these patients free of metastasis at 4 years . BAP1 mutations are associated with Class 2 uveal melanomas .
The management of uveal melanoma has also shifted to eye-sparing approaches, without significantly impacting patient survival. Radiotherapy with proton beams has replaced eye enucleation as the standard treatment.
Mucosal Melanoma
Mucosal melanomas are rare lesions that may occur in the mouth, nasopharynx, larynx, and anogenital mucosae. They tend to occur near the mucocutaneous junctions of squamous and columnar epithelia and account for ~1% of melanomas. As many as 35% of mucosal melanomas are reportedly amelanotic, further complicating a difficult clinical diagnosis . Not surprisingly, these tumors tend to be diagnosed at a locally advanced stage with associated poor prognosis. Similar to ALM, mucosal melanomas are a genetically distinct subtype of melanoma. They harbor more and different DNA copy number changes than do cutaneous melanomas and they can harbor activating KIT mutations, which can make the tumor sensitive to KIT-inhibiting drugs (e.g. imatinib; see Figs 113.1 & 113.2 ) .
Melanoma and Pregnancy
During pregnancy, levels of hormones and growth factors that stimulate melanocytes are elevated and increased pigmentation occurs in some women. More than 10% of women experience darkening of melanocytic nevi during the first 3 months of pregnancy . However, an association between hormonal changes during pregnancy and development of melanoma or worsening of the prognosis of an existing melanoma has not been demonstrated . Transplacental metastases are very rare in pregnant women with melanoma and only a limited number of cases have been described in the literature . Surgical excision and sentinel lymph node biopsy (if chosen by the patient) are performed as indicated, based upon stage of disease and current recommendations (see below). In more advanced stages, the advantages and disadvantages of immunotherapy and targeted therapy should be discussed with the patient. Ultrasound and magnetic resonance imaging can be performed in pregnant patients, but computed tomography (CT) scans should be avoided. In women with a history of high-risk melanoma, it may be reasonable to wait 2 years after diagnosis before becoming pregnant again, because two-thirds of recurrences occur within this period. Lastly, there have been no studies demonstrating an adverse effect of hormonal contraception on melanoma development .
Childhood Melanoma
Childhood melanoma is very rare. Approximately 2% of melanomas occur in patients younger than 20 years of age, and 0.3% in those younger than 14 years . Risk factors for melanoma in children parallel those in adults. The exceedingly rare conditions of xeroderma pigmentosum and giant congenital melanocytic nevi contribute minimally to the prepubertal incidence of melanoma.
Pediatric melanomas, especially in prepubertal children, were found in a series of 70 patients to frequently lack conventional criteria. As a result, alternative ABCD criteria were proposed: A – amelanotic; B – bleeding, bump; C – color uniformity; and D – de novo appearance, any diameter . Histologically, melanomas in children may resemble those of adults, but melanomas with features of Spitz nevus are reported to be more common in this age group . As noted above, the differentiation of melanoma from atypical Spitz nevus and from deep penetrating nevus remains a major challenge, and overdiagnosis of childhood melanoma may result from this uncertainty . Overall survival and prognosis appears to be stage-dependent and similar to that in adults . Treatment follows the same rationale as in adults.
Diagnosis
Early detection is a key factor for improving survival in patients with melanoma. The clinical diagnosis of cutaneous melanoma continues to be based on visual inspection and dermoscopy. A history of change in the color, shape or size of a pigmented skin lesion over the course of months or years is the most sensitive clinical sign for melanoma, and may be detected by the patient himself or by serial screening examinations plus dermoscopy. In high-risk patients, the latter may be supplemented with dermoscopic image storing and lesional or total body photography .
Public awareness campaigns have highlighted the ABCDs of melanoma: A symmetry, B order irregularity, C olor variegation, and D iameter >5 mm. However, the ABCD rule widely overlaps with the definition of benign atypical melanocytic nevi. Furthermore, it has been shown that patients recognize a melanoma by the development of a new pigmented lesion or by an increase in size or change in color of a pre-existing lesion instead of by the ABCD features . Thus, an “ E ”, for E volving, has been added to highlight the importance of a changing lesion.
Dermatologists seem to unconsciously rely on cognitive (overall pattern) and comparative (ugly duckling sign) processes rather than an algorithm of morphologic criteria (ABCD) . A diagnostic clue is the so-called “ugly duckling” sign , which focuses on the morphologic differences between a single striking lesion and the surrounding benign “signature” nevi . There is a good interobserver reproducibility of this sign .
Two other helpful clinical clues are the “Little Red Riding Hood” sign and the “EFG” rule . The former represents the finding of erythema or inflammation that at times will surround a cutaneous melanoma. “EFG” stands for an E levated, F irm, or G rowing lesion and is helpful to keep in mind for accurately diagnosing an amelanotic or nodular melanoma.
It is important to keep in mind that not all cutaneous melanomas present with these typical clinical clues. Diagnostic accuracy for the clinical diagnosis of melanoma does not exceed 75% and may be increased to up to 90% with the use of dermoscopy by experts. However, 10–25% of melanomas may still be missed despite all these diagnostic efforts. The term “featureless melanoma” has been coined for early and difficult-to-diagnose melanomas and may partially explain this statistic . Sometimes, patients are the first to recognize these featureless cutaneous melanomas and infrequently patients are very suspicious about a single such skin lesion. Therefore, the following principle for the management of skin cancer patients is suggested, which may be called “Garbe’s rule”: If a patient is worried about a single skin lesion, do not ignore their suspicion and have a low threshold for performing a biopsy.
Differential Diagnosis
A variety of conditions may simulate melanoma, either clinically or histopathologically, or both. Awareness of these simulators is of great practical importance in order to avoid misdiagnosis or overdiagnosis of melanoma . Tables 113.3 and 113.4 list several melanocytic and non-melanocytic lesions that can mimic melanomas. Rare primary melanomas arising in the dermis with no epidermal component may be misdiagnosed as a dermal metastasis of melanoma.

NON-MELANOCYTIC CLINICAL SIMULATORS OF MELANOMA |
Simulating superficial spreading melanoma |
|
Simulating nodular melanoma |
|
Simulating lentigo maligna melanoma |
|
Simulating acral lentiginous melanoma of the palms and soles |
|
Simulating subungual acral lentiginous melanoma |
|
Simulating amelanotic melanoma |
|
Dermoscopy
Dermoscopy, also known as skin surface microscopy or epiluminescence microscopy (ELM), is a helpful non-invasive tool for diagnosing pigmented skin lesions and for recognizing cutaneous melanoma (see Figs 113.9, 113.11, 113.13, 113.15 ). Clinical diagnostic sensitivity is significantly enhanced by the use of dermoscopy and the correct diagnosis of melanoma is improved by nearly 50% by utilizing this diagnostic technique .
In one form of dermoscopy, a magnifying lens system is combined with a fluid interface in order to eliminate skin surface reflection. Hand-held lenses or cameras equipped with lenses and coupled with digital imaging systems are used. A glass plate makes direct contact with the skin and for the fluid interface, ultrasound contact gel or a disinfectant spray can be used. Morphologic structures within the epidermis, the dermal–epidermal junction, and the superficial dermis can be visualized with this technique. An alternative form of dermoscopy employs polarized light to eliminate the surface reflection so that neither a liquid interface nor direct skin contact is required. The magnifications of these instruments range from 6- to 100-fold, but the most widely used dermoscope provides a 10-fold magnification, which is sufficient for routine assessment of pigmented skin lesions.
The dermoscopic evaluation of cutaneous pigmented lesions involves a two-step algorithm. First, the observer determines whether the lesion under investigation is of melanocytic origin or not. For a lesion to be considered melanocytic, it needs to have at least one of the following dermoscopic structures/features: pigment network, streaks, aggregated globules, homogeneous blue pigment, or parallel pattern (acral lesions). If the lesion does not possess one of the aforementioned melanocytic features, then it needs to be evaluated further to determine if it has any dermoscopic characteristics consistent with other entities such as a seborrheic keratosis, dermatofibroma, or pigmented basal cell carcinoma (see Ch. 0 ). However, if the lesion does not have any features of a melanocytic lesion and it does not have any features of a non-melanocytic tumor, then, by default, the lesion is considered to be of melanocytic origin.
Once a lesion is deemed to be of melanocytic origin, the second step of the algorithm is to differentiate between benign melanocytic nevi and melanoma. To this end, multiple algorithms have been created, including pattern analysis, the ABCD rule, Menzies method, and the 7-point checklist, among others, which assist the clinician in deciding which lesions require a biopsy (see Table 0.17 , Table 0.18 , Table 0.19 , Table 0.20 ) .
The major dermoscopic criteria for melanoma can be broken down into global features, patterns, and local features. Global features of melanoma include dermoscopic asymmetry and the presence of multiple colors. Patterns seen by dermoscopy include reticular, globular, reticular–globular, homogeneous, reticular–homogeneous, and starburst. In melanoma, the most common patterns are the multi-component pattern (three or more dermoscopic structures distributed asymmetrically), asymmetric starburst pattern, and nonspecific pattern (does not fit one of the known benign patterns). Lastly, the presence of any of the following local features should raise concern for melanoma: atypical network, streaks, atypical dots or globules, irregular blood vessels, regression structures, and blue–white veil ( Table 113.5 ).
DERMOSCOPIC CRITERIA AND THEIR CORRESPONDING HISTOPATHOLOGIC FEATURES | |||
---|---|---|---|
Criterion | Morphologic definition | Associated histopathologic changes | Diagnosis |
Pigment network | Network of brownish lines over a diffuse tan background | Pigmented rete ridges | Melanocytic lesion |
Typical network | Brown-colored, regularly meshed and narrowly spaced network | Regular and elongated rete ridges | Benign melanocytic lesion |
Atypical network | Black, brown or gray network with irregular meshes and thick lines | Irregular and broadened rete ridges | Melanoma |
Dots/globules | Black, brown and/or gray, round to oval, variably sized structures regularly or irregularly distributed within the lesion | Pigment aggregates within the stratum corneum, epidermis, dermal–epidermal junction or papillary dermis | If regular: benign melanocytic lesion; if irregular: melanoma |
Streaks | Irregular, linear structures not clearly combined with pigment network lines at the margins | Confluent junctional nests of melanocytes | Melanoma |
Blue–white veil | Irregular, confluent, gray–blue to whitish-blue diffuse pigmentation | Acanthotic epidermis with focal hypergranulosis above sheets of heavily pigmented melanocytes in the dermis | Melanoma |
Blotches | Black-, brown- and/or gray-colored areas with regular or irregular shape/distribution | Hyperpigmentation throughout the epidermis and/or upper dermis | If regular: benign melanocytic lesion; if irregular: melanoma |
Regression structures | White (scar-like) areas, blue (pepper-like) areas or combinations of both | Thickened papillary dermis with fibrosis and/or variable amounts of melanophages | Melanoma |
Milia-like cysts | White–yellowish, roundish dots | Intraepidermal horn globules, also called horn pseudocysts | Seborrheic keratosis (occasionally observed in papillomatous melanocytic nevi) |
Comedo-like openings | Brown–yellowish, round to oval or even irregularly shaped, sharply circumscribed structures | Keratin plugs situated within dilated follicular openings | Seborrheic keratosis |
Leaf-like areas | Brown–gray to gray–black patches revealing a leaf-like configuration | Pigmented, solid aggregations of basaloid cells in the papillary dermis | Basal cell carcinoma |
Red–blue lacunas | Sharply demarcated, roundish to oval areas with a reddish, red–bluish, or red–black color | Dilated vascular spaces situated in the upper dermis | Vascular lesion |
Vascular structures | Comma-like vessels | Benign melanocytic lesion | |
Arborizing vessels | Basal cell carcinoma | ||
Hairpin vessels | Seborrheic keratosis | ||
Dotted or irregular vessels | Melanoma |
Over the past several years, dermoscopy has been further refined in order to recognize difficult-to-diagnose melanomas such as ALMs and subungual melanomas . Additionally, diagnostic clues for early nodular melanomas and for amelanotic melanomas have been described, as these melanomas do not display the classic features (see above) . It is noteworthy that not all melanomas can be clearly recognized by dermoscopy and that “featureless” melanomas exist .
Photography
In high-risk patients, especially those with complex mole patterns, baseline photographs can be a helpful adjunct in the identification of new and changing lesions. The advent of digital photography and computerized image archives has improved the practicality of this approach. The availability of baseline images for comparison, including dermoscopic images, permits the detection of melanomas that are growing or changing without manifesting obvious clinical characteristics, while simultaneously avoiding the excision of stable, albeit clinically concerning, atypical nevi.
Reflectance Confocal Microscopy (RCM)
Reflectance confocal microscopy is a non-invasive method for in vivo imaging of the skin. In particular, structures within the epidermis and papillary dermis are visualized with high resolution. This technique has been used to analyze cutaneous tumors, including melanoma ( Fig. 113.21 ).

Histopathology
Histopathology remains the gold standard for melanoma diagnosis. While most melanomas are readily distinguished from melanocytic nevi based on standard criteria ( Table 113.6 ), some melanocytic lesions remain diagnostically challenging despite the advent of immunohistochemical and molecular techniques. There is significant variability in the application of diagnostic criteria for thin and in situ melanomas among pathologists .
CRITERIA FOR THE HISTOPATHOLOGIC DIAGNOSIS OF MELANOMA |
Architectural pattern |
|
Cytomorphology |
|
Other features |
|
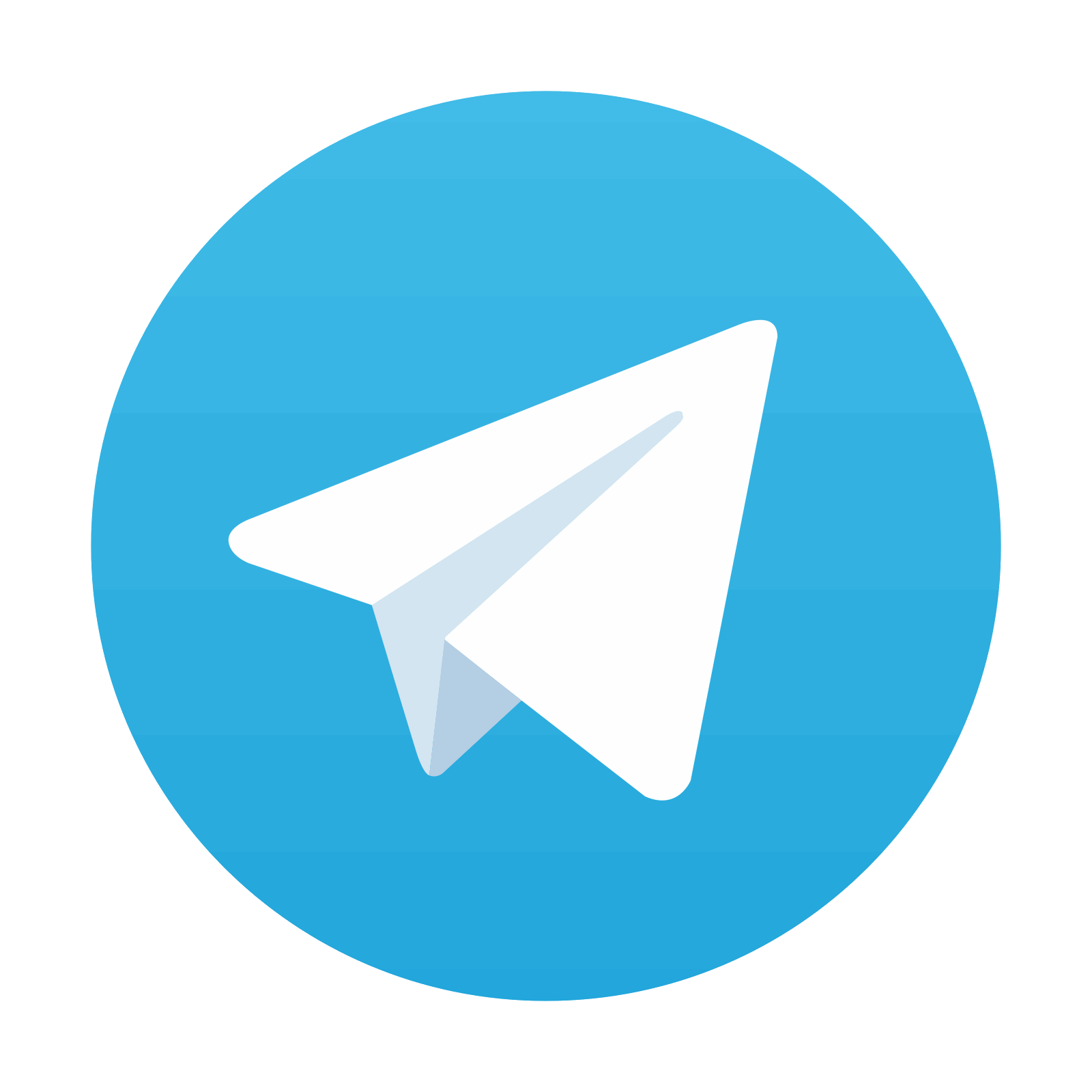
Stay updated, free articles. Join our Telegram channel

Full access? Get Clinical Tree
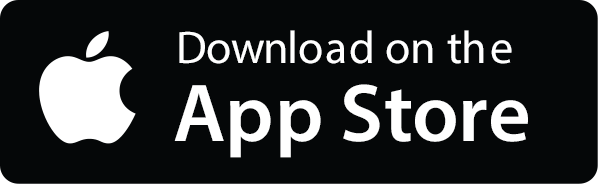
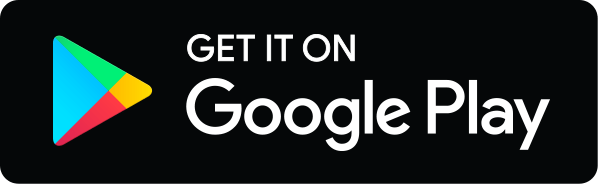
