Chapter 6 Gene Therapy for Tendon Healing
Outline
Improvement in tendon healing through biological approaches, molecular manipulations in particular,1–8 is a relatively new area of investigation related to flexor tendon surgery. These biological manipulations are intended to overcome a major obstacle to success in tendon repair—low intrinsic healing potential. The development of molecular treatment of tendon healing has benefited from advances in biotechnology and a recent exponential expansion in available genetic information. We have noted that biological investigations are catching up with mechanical studies, making an increasingly greater contribution to the efforts to improve tendon repairs.9–29 From these biological investigations, we are rapidly accruing information regarding the molecular mechanisms and efficacy of manipulations of tendon healing, which may bear fruit in the future.
Of gene therapy applications in tissue healing, the unique advantage is that this strategy necessitates production of the encoded factors for a fairly short period.30–32 Gene therapies traditionally aimed at curing tumors or congenital diseases, in contrast, necessitate long-term transgene expression, usually over the entire lifetime. Such prolonged transgene expression is still a difficult goal to reach reliably for chronic conditions, which constitutes an obstacle to ultimate clinical success in curing these diseases. However, for tissue repair, gene therapy can establish a local, endogenous synthesis of the authentically processed materials critical to the healing. Long-term gene expression is neither necessary nor desired.30 The required period of transgene expression for tissue healing exactly fits current possibilities offered by gene therapy.
Innate Weakness in Intrinsic Tendon Healing and “no-Gain” Period in Strength
Tissues nourished with rich blood supplies usually acquire mechanical strength rapidly in the first and second postsurgical weeks. However, the tendon healing process is characterized by a “no-gain” period in strength over the first 3 to 4 weeks (Figure 6-1)5,33–35 due to the weak healing capacity, yielding slow healing responses after injury. The innate weakness in tendon healing relates to the following structural facts: (1) they lack sufficient blood supply, (2) have sparse cellularity, and (3) are mainly composed of tightly packed collagen bundles. The characteristic no-gain period in strength is the central issue underlying the difficulties and clinical problems associated with tendon repairs. The goal of present efforts through biological manipulations is to elicit an earlier increase in strength, helping the tendon “leap over” this lag period. Theoretically, this goal is achievable through targeting major causes of weak tendon healing, either by supplementation of cells involved in healing or by increasing the activity levels of growth factors. Because healing strength is structurally based on collagen connections across the tendon repair site, we expect that addition of specific cells or growth factors will ensure speedy deposition and maturation of collagens at the healing site, resulting in an earlier gain in mechanical strength.
Overall Concepts and Methods of Gene Therapy
The ideas behind gene therapy date back to the early 1970s. In 1972, Friedmann and Roblin advocated introducing exogenous genes to cure human diseases in an article entitled, “Gene Therapy for Human Genetic Disease?”36 Major advances were made in the technical aspects of gene therapy in the 1990s. Over the past two decades, investigators have taken logical steps of using gene therapy to pursue curing diseases caused by single-gene defects (e.g., cystic fibrosis, hemophilia, muscular dystrophy, and sickle cell anemia) or in the treatment of certain cancers. More recently, clinical work has included a trial for treating Leber congenital amaurosis,37 a disease caused by mutations in the RPE65 gene. The investigators proved the safety of subretinal delivery of recombinant adeno-associated virus (AAV) carrying the RPE65 gene and found a modest improvement in vision of the patients.37 In another study, investigators restored trichromatic vision to squirrel monkeys using gene therapy to cure red-green color blindness.38 Other investigators succeeded in treating adrenoleukodystrophy (ADL), a fetal brain disease, by delivering the gene for the missing enzyme to patients via a lentiviral vector.39
Various techniques have been developed to achieve successful gene transfer,32 including both nonviral methods (e.g., liposomal, electroporation, sonoporation, shots from a “gene gun,” etc.) and viral vectors (e.g., adenoviruses, AAVs, herpes simplex viruses, retroviruses, lentiviruses, etc.).
Biological Methods to Enhance Tendon Healing
One possible way to augment tendon healing is to provide the tendons with exogenous cells, to ameliorate sparse cellularity. A few such attempts have been made. Direct delivery of bone marrow stem cells to healing rabbit Achilles tendons produced some evidence of increases in tendon strength.40 Investigators coated surgical sutures with bone marrow stem cells and delivered those cells to the tendon in vitro,6 but the in vivo efficacy of this method has not been reported.
Growth factors, such as insulin-like growth factor-1 (IGF-1),9,10 platelet-derived growth factor-B (PDGF-B),20 and basic fibroblast factor (bFGF),20,23,25 have been reported to promote tenocyte proliferation or collagen synthesis. Direct supplementation of growth factors to the healing tendons has failed to reliably improve healing strength. Chan and colleagues41 injected bFGF to rat cut patellar tendon and detected no effects on ultimate strength of the tendon 7 and 14 days after surgery despite increases in cell proliferation and type III collagen production. Surgical sutures can be coated with growth factors such as bFGF or growth differentiation factor-5 (GDF-5).4,42 Surgical repairs using such coated sutures improved the strength of the rabbit flexor tendon only at a single time point (postoperative week 3) but failed to improve strength at other time points.4,42
Controlled-release drug delivery systems is another way to provide the tendon with a prolonged supply of growth factors.7,21 Growth factors can be incorporated into polymers in vitro, and the impregnated polymers are then implanted into the tissues, which may deliver the growth factors continuously for a prolonged period in a controlled fashion. This system holds promise for use in tendon repairs, but research in this area is just beginning. Growth factors (bFGF and PDGF-BB) have been incorporated into fibrin matrices and the release kinetics has been tested over a 10-day-period in vitro21; bFGF and PDGF-BB were found to release gradually from the fibrin matrices and to modulate cell proliferation and extracellular matrix synthesis in vitro. However, this system loaded with PDGF-BB was not found to improve the strength of the healing canine flexor tendons at days 7, 14, and 42 after surgery.7 In a canine model, bFGF delivered through fibrin heparin–based controlled-release system stimulated cellular proliferation, promoted neovascularization and inflammation of the flexor digitorum profundus (FDP) tendon in the earliest stages after surgical repair, but it failed to improve mechanical strength of the repair.43 This system remains to be fully tested with respect to its effectiveness to increase tendon strength in vivo.
Gene Therapy Approaches—the First Step: Choosing Genes and Delivery Methods
Over the past 10 years, we have directed our efforts toward improving tendon healing through augmentation of growth factor activity (Figure 6-2). To test the efficacy of this approach, we faced two initial questions: (1) what genes should be delivered, and (2) which are safe and efficient gene-carrying vehicles for our purposes?
To answer the first question, we examined levels of growth factors in the healing digital flexor tendons. Growth factor genes with low levels of expression in the healing process are considered targets of gene therapy. In a chicken tendon laceration model, we examined the levels of growth factors from day 3 to week 12 after surgery. bFGF and PDGF-BB were found to have the lowest expression in the early period28; VEGF and IGF-1 were expressed in modest amounts.28 Consequently, we decided to target the bFGF gene initially, and the VEGF gene secondarily.
Our answer to the second question—how best to deliver the genes—was based on an essential consideration: the intended therapy is aimed at the healing of normal tissues, requiring only short transgene expression, rather than treating cancers or curing inherited diseases. In this regard, safety issues of the vectors should be the primary concern. With these considerations in mind, we first eliminated retroviral vectors. Lentiviral vectors and herpes simplex viral vectors were next excluded, because of concerns of biosafety of lentiviral vectors (e.g., potentials of generation of replication-competent lentivirus and oncogenesis) and the narrow applications of herpes simplex viral vectors (i.e., in the nervous system).44 Adenoviral, AAVs, and liposome-mediated gene transfer thus became viable candidates.
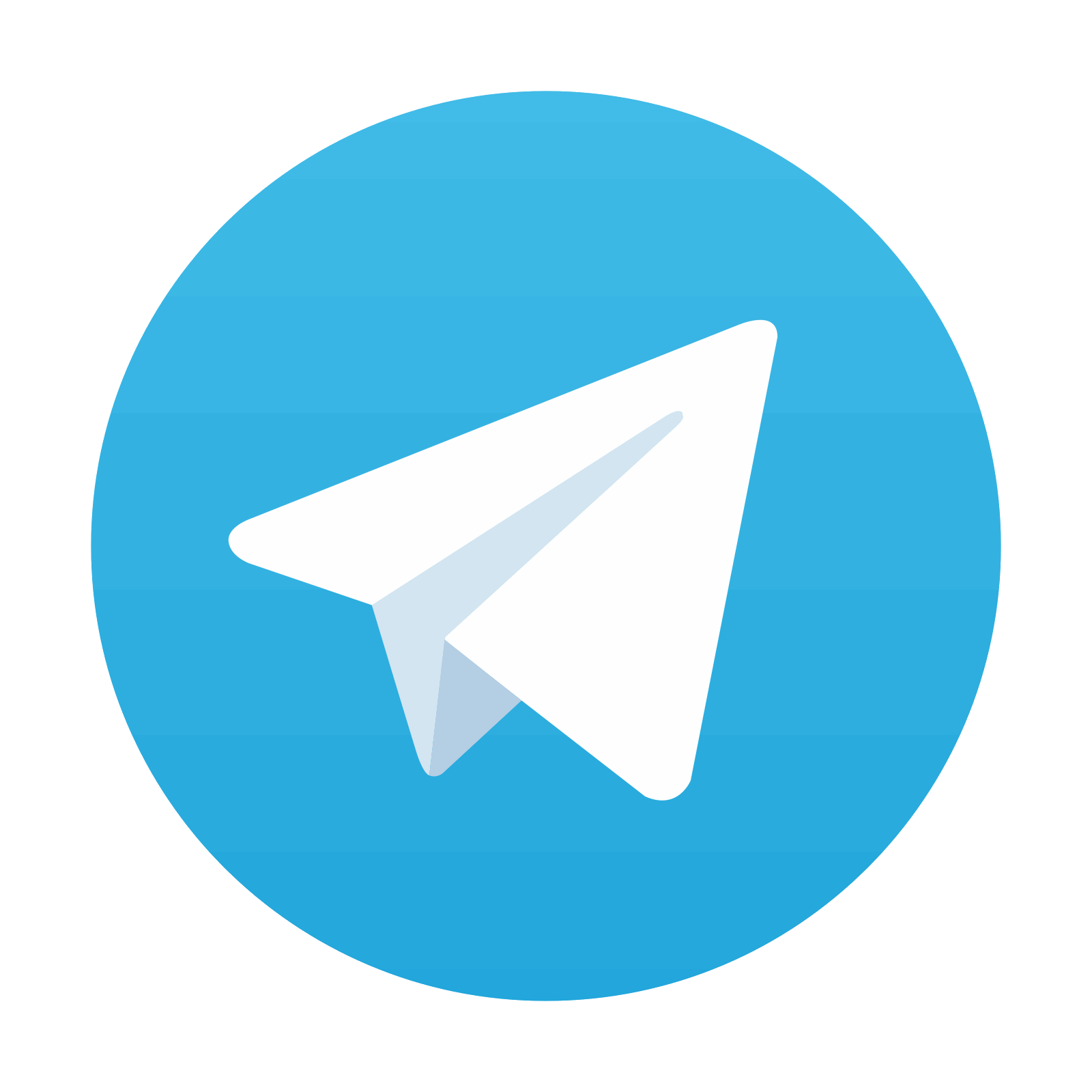
Stay updated, free articles. Join our Telegram channel

Full access? Get Clinical Tree
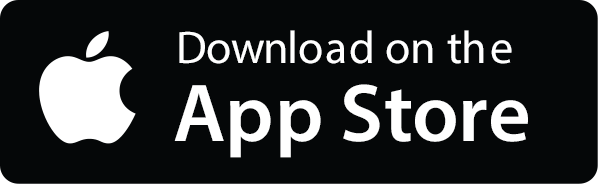
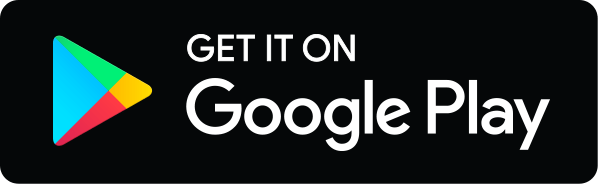