15 Capsular Contracture and Biofilms—Etiology, Prevention, and Treatment
Summary
The development of clinically apparent peri-implant capsular contracture can be a challenging problem, both for the patient as well as for the surgeon. In this chapter, the classification, pathogenesis, prevention, and treatment options will be discussed.
15.1 Introduction
Capsular contracture (CC) is a common but unpredictable complication following aesthetic and reconstructive breast prosthesis implantation. The reported rates of CC vary widely, ranging from 0.5% to 59%. 1 , 2 The heterogeneity of reported studies (implant type, surgical technique, and follow up period) precludes an accurate estimate of overall incidence. Nonetheless, CC is one of the commonest indications for reoperation in aesthetic breast surgery. 3 Moreover, revisional surgery is technically challenging, with poorer patient satisfaction. 4 This chapter aims to review the pathogenesis of CC formation with a focus on the subclinical infection theory, biofilm formation, and the implications of implant texturing and planes. The evidence for current nonoperative and operative strategies for CC will also be evaluated.
15.2 Classification
Although various classification tools have been proposed to score the severity of CCs, the Baker and Spear’s modified Baker grading systems are the most widely used scales. 5 , 6 These classifications are simple grading systems that recognize clinical features such as implant visibility and firmness.
15.3 Pathogenesis
Several hypotheses have been proposed to account for the pathogenesis of capsular formation in prosthetic breast surgery (Fig. 15‑1).

15.3.1 Biofilm and Subclinical Infection Theory
Most bacterial species in nature exist in two different forms: free-floating planktonic form or as a sessile biofilm. 7 The preliminary stage of biofilm formation begins with the reversible attachment of planktonic cells to a surface, mediated by pili, flagellae, and van der Waals forces. 7 , 8 The bacterial secretion of extracellular polysaccharide (EPS) matrix, the hallmark of biofilm formation, contributes to irreversible adhesion of the microbes to the underlying implant surface. Following attachment, bacteria proliferate and continue to synthesize EPS matrix, resulting in the formation of highly structured microcolonies. 7 , 9 These microcolonies vary in pH, oxygen concentration, nutrient availability, and cell density. Sustained growth and differentiation of the microcolonies culminate in the formation of a mature biofilm community. Dispersion of biofilm cells through active or passive detachment may contribute to local extension over the implant surface. 7 , 8
The biofilm architecture contributes to host resistance and survival advantages. 7 , 8 The EPS matrix serves as a diffusion block and mechanical barrier to antimicrobials and immune cells; the reduced metabolic activity of sessile bacteria increases antimicrobial tolerance; and the microarchitecture allows transfer of antimicrobial resistance genes carried on mobile genetic elements (plasmids). 7 , 8 The host immune response to the presence of bacterial biofilms and their associated antigens induces a prolonged and sustained inflammatory response. Although the recruitment of proinflammatory cells (macrophages and lymphocytes) and proteases aids in dislodging biofilms, they also damage normal tissue. The recruitment of macrophages and myofibroblasts within the chronic inflammatory milieu contributes to capsule formation. 8 Studies suggest that myofibroblast-driven centrifugal forces directly contribute to contracture formation around a prosthesis. 10 , 11
Although biofilms are typically polymicrobial, a few species are overrepresented. Staphylococcus epidermidis, a normal constituent of skin and endogenous breast microflora, is the most frequently identified organism on breast implants removed because of CC. 12 , 13 , 14 , 15 , 16 Other bacterial species implicated in the formation of breast implant biofilms include Propionibacterium acnes, Staphylococcus aureus, Bacillus, Escherichia coli, Mycobacterium species, Corynebacterium, and Lactobacillus. 7 , 12 , 13 , 14 , 15 Potential microbial sources include contaminated implants, contaminated saline, poor surgical technique, and mammary duct disturbance, particularly with periareolar surgical approaches. The mammary ducts host bacterial colonies in more than 90% of cases. 17 Predisposing factors for late colonization include secondary seeding from bacteremia (e.g., dental source). 14 Rieger et al recently demonstrated a clear correlation between the degree of CC and the presence of bacterial biofilms on implant surfaces. 13 Interestingly, a recent study has confirmed high levels of bacteria in breast implant–associated anaplastic large cell lymphoma (ALCL) specimens, with a gram-negative shift in the microbiome as compared to the more traditional biofilm species detected in CC cases. 18 . The gram-negative profile has been implicated in the malignant transformation, 18 a distinction from the inflammation and fibrosis pattern observed in CC alone.
15.3.2 Implant Texturing
Two systematic reviews 19 , 20 have confirmed the protective effect of surface implant texturing on the rate of CC. These studies collectively reported a greater incidence of CC in smooth implants (5–7 times) over a three-year follow-up period. 19 , 20 Brandt and colleagues 21 , 22 have theorized that smooth-surfaced implants experience increased contracture rates because they predispose to a planar arrangement of fibroblasts with organized collagen deposition and bridging synovial epithelium around implants, favoring CC formation. By contrast, as textured implants typically have grooves larger than the diameter of a fibroblast, the surface texturing causes the cells to anchor in deep and random patterns, disrupting any planar arrangement or centrifugal moment. 7 , 23
The relationship between implant texturing and biofilm development is complex. Several in vitro studies have reported higher rates of biofilm formation in textured implants compared to smooth implants, given their greater surface area and enhanced bacterial adhesion on rough surfaces. 7 , 24 , 25 , 26 Contrastingly, Jacombs et al reported no significant difference in the rate of biofilm formation between smooth surface implants and textured surface implants following deliberate inoculation with human S. epidermidis in a porcine model. 26 The authors suggested that a threshold of biofilm load exists, which, once crossed, leads to significant potentiation of capsule formation, regardless of implant texturing. Despite this, the recent implication of textured implants and biofilms in the causation of ALCL warrants discussion. Loch-Wilkinson et al 27 proposed that the contamination of textured implants with biofilm leads to chronic antigen stimulation in genetically susceptible hosts, prompting the proliferation of mutant T cells into malignant clones.
15.3.3 Implant Position and Incision Choice
A recent meta-analysis demonstrated significantly higher rates of CC with periareolar (7.2%) incisions as compared to combined nonperiareolar approaches (inframammary and transaxillary; 3.1%). 28 Periareolar incisions increase the risk of transection of large end ducts, leading to implant contamination with intraductal material and subsequent biofilm formation. Arguably, the release of bacterially colonized material may continue until the transected ducts have healed and scarred. The impact of transaxillary approaches on CC formation has been variably reported. Previous criticisms of hematoma formation from blind transaxillary pocket dissection have been largely addressed through endoscopic assistance. Tebbetts reported a decrease in CC from 4.2% to 1.3% after adopting an endoscopic transaxillary technique. 29
Moreover, implant pocket location also appears to have an impact on development of CC. Recent meta-analyses have reported that subpectoral implant placement is associated with a lower incidence of CC, as compared to subglandular implants. 19 , 20 It is hypothesised that this results from reduced exposure to endogenous glandular bacteria.
15.4 Prevention
On the basis of increasing evidence supporting the subclinical infection theory and biofilm formation as a significant cause of CC, Deva et al 34 proposed a “14-point plan” to reduce the risk of prosthetic contamination at the time of implantation. These steps represent a codification of evidence to support bacterial mitigation strategies at the time of implant deployment. Since publication of the plan, an increasing number of surgeons and breast registries have supported the integration of these steps into their clinical practice. Many of the principles of the 14-point plan, 34 shown in Box 15.1, rely simply upon meticulous operative planning and aseptic surgical technique. However, the use of drainage tubes and pocket irrigation warrants a closer evaluation of existing scientific evidence.
Box 15.1 The 14-Point Checklist
Use intravenous antibiotic prophylaxis at the time of anesthetic induction.
Avoid periareolar incisions.
Use nipple shields to prevent spillage of bacteria into the pocket.
Perform careful atraumatic dissection to minimize devascularized tissue.
Perform careful hemostasis.
Avoid dissection into the breast parenchyma. The use of a dual-plane, subfascial pocket has anatomic advantages.
Perform pocket irrigation with triple antibiotic solution or povidone-iodine.
Use an introduction sleeve (Keller funnel, cut-off glove, povidone-iodine-impregnated drapes) to minimize skin contact with implant.
Use new instruments and drapes, and change surgical gloves prior to handling the implant.
Minimize the time of implant opening.
Minimize repositioning and replacement of the implant.
Use a layered closure.
Avoid using a drainage tube, which can be a potential site of entry for bacteria.
Use antibiotic prophylaxis to cover subsequent procedures that breach skin or mucosa.
15.4.1 Pocket Irrigation
Burkhardt and colleagues championed the use of povidone-iodine for breast pocket irrigation as a means of preventing subclinical pocket infection. 35 , 36 , 37 Following in vitro studies, Adams et al reported that pocket irrigation with triple antibiotic solution (cefazolin, gentamicin, bacitracin) with or without povidone-iodine was associated with low CC rates in primary aesthetic breast augmentation and implant-based breast reconstruction. 38 , 39 A systematic evaluation of routine antibiotic pocket irrigation is difficult, as most existing studies have a high risk of bias as well as significant clinical and methodological heterogeneity. Two recent studies, however, have shown a 10-fold reduction in capsular contracture following use of either antiseptic or antibiotic irrigation. 40 , 41
15.4.2 Drainage Tubes
The theoretical argument for use of drainage tubes is to reduce the risk of hematoma formation, which may stimulate local inflammatory reactions and serve as a culture medium for microorganisms. Despite this rationale, closed suction drains provide a direct portal of entry for bacteria into the surgical site, increasing the risk of infection, biofilm formation, and CC. 2 , 42 , 43 Dower demonstrated biofilm bacterial contamination of drains as early as 2 hours postoperatively. 43 Araco et al reported a fivefold increase in the risk of developing an acute infection when drains were used, in a retrospective review of breast augmentation cases. 2 Similarly, Henriksen et al reported that use of drains increased CC by at least twofold. 42
15.5 Management
15.5.1 Nonsurgical
No scientific consensus exists regarding the use of nonsurgical modalities in the management of established CC.
Leukotriene Receptor Antagonists
Leukotriene receptor antagonists (zafirlukast and montelukast) can reduce the severity of CC by modulating the inflammatory response to implant placement. Several clinical studies have demonstrated a reduction in Baker grading of CC with administration of zafirlukast (20 mg twice daily) for a period of 3–6 months. 44 , 45 , 46 Another study conducted by Mazzocchi et al demonstrated that mammary compliance declined following cessation of zafirlukast, suggesting that the long-term effect of the drug is contingent on persistent dosing. 47 Dosing must be counterbalanced against drug side effects (hepatic). 31 In regard to contracture prevention, a recent study conducted by Bresnick (n = 1,122 primary breast augmentation cases) reported that prophylactic use of zafirlukast (20 mg twice daily) for 3 months postoperatively was associated with significantly reduced CC rates compared with untreated patients (2.2% versus 5%) at 1 year of follow-up. 48 No statistical significant difference in CC rates was observed with prophylactic administration of montelukast. It is hypothesised that zafirlukast inhibits cysteinyl leukotrienes (LTC4 , LTD4, LTE4), suppressing their effects on macrophages and myofibroblasts. 48 Montelukast has a narrower spectrum of action (it does not inhibit LTC4 or LTE4), which may account for its reduced efficacy. 48
Corticosteroids
Many papers have reported the use of corticosteroid injections (triamcinolone acetonide) in the management of established CC. 49 , 50 , 51 , 52 A study conducted by Sconfienza et al demonstrated that ultrasound-guided injection of 40 mg of triamcinolone acetonide in the peri-implant area of breasts with Baker grade IV CC was effective in reducing capsular thickness and patient discomfort, even in patients who had undergone adjuvant chemoradiotherapy. 52 Anecdotally, the risk profile of corticosteroid injections, such as soft tissue atrophy, discoloration, implant perforation, and infection, means that they are not routinely utilized by most surgeons. 53
15.5.2 Surgical
Revision breast surgery in augmentation mammaplasty presents particular challenges. In addition to CC, the thinned soft tissue interface and scarred breast envelope may contribute to ptosis and implant malposition. For most surgeons, the management principles of established CC involve a combination of capsulectomy, site change, and implant exchange in either an immediate or a staged approach.
Capsulectomy versus Capsulotomy
A systematic review conducted by Wan and Rohrich 4 reported that capsulectomy was associated with variable contracture recurrence rates (0–53%), similar to those observed with open capsulotomy (0–54%). Additionally, conflicting scientific evidence exists regarding the benefits of total versus partial capsulectomy. 4 , 58 , 59 Proponents for total capsulectomy argue that contracted capsules are colonized with biofilm and should be excised as completely as possible. Critics, however, argue that biofilms are only one aspect in CC formation, with negative bacterial cultures seen in up to 33% of contracted capsules. 4 , 12 , 14 Consequently, they argue that open capsulotomy alone may be effective in disrupting myofibroblast tension around the prosthesis. Given the inadequate scientific evidence, Box 15.2 highlights key principles in the surgical decision making for capsule management. 4 , 58 , 59 , 60 , 61 , 62
Box 15.2 Management Principles for Capsulectomy (Partial versus Total) and Capsulotomy
Total capsulectomy may be attempted in the subglandular plane (provided adequate breast tissue exists). If the overlying gland is significantly atrophic, an anterior capsulectomy may compromise skin or cause distortion. In these cases, posterior capsulectomy alone may be performed.
Anterior capsulectomy is recommended in the subpectoral plane to avoid chest wall injury.
Thick, calcific, or granulomatous capsules should be excised to minimize breast distortion and prevent interference with future mammography.
In cases where capsulectomy (total/ partial) would excessively damage surrounding tissue (particularly with atrophic gland or pectoralis major), open capsulotomy (with or without radial scoring) is appropriate.
Change of Implant Site or Plane
Most surgeons strongly advocate change of implant site or plane at the time of revisional surgery to limit new periprosthetic scar formation and inflammation. Wan and Rohrich 4 reported that site change procedures (with limited or no capsulectomy) were associated with lower CC recurrence rates (0–12%) compared with no site change (0–54%). The vast majority of the studies included in the review used a neo-pocket as their method of pocket conversion, which is not representative of plane change in the traditional sense. Nonetheless, most surgeons agree that subglandular contractures are best treated with conversion to the subpectoral pocket or dual-plane technique. 4 Equally, most advocate staying in the submuscular plane to manage subpectoral contractures as well, with neo-pocket formation or limited capsulectomy. 4 , 63 , 64 Conversion to a subglandular plane is rarely preferred, as the overlying tissue is often inadequate and the plane naturally subjects the implant to a higher endogenous bacterial load. 4 , 19 , 20
Implant Exchange
Most surgeons strongly advocate for implant exchange in revisional surgery. Wan and Rohrich 4 reported that implant exchange (with or without pocket change) was associated with lower CC recurrence rates (0–26%) compared to studies in which no implant exchange was performed (0–54%).
Acellular Dermal Matrices
Current literature suggests a potential role for acellular dermal matrices (ADMs) as a reinforcement in regions of atrophic breast tissue or at the site of the capsulectomy, where it acts an antigen-free barrier between the implant and breast tissue. 4 , 65 , 66 , 67 , 68 , 69 . The barrier is believed to dampen the host–foreign body immune response and reduce the risk of capsule formation. Basu et al 69 demonstrated significantly reduced chronic inflammatory changes, giant cell infiltrate, and capsule fibrosis in integrated ADM compared to implant capsules in a histopathologic study. Moreover, in their systematic review, Wan and Rohrich 4 reported that ADM was associated with consistently lower CC recurrence rates (0–7%), with a mean follow-up of 3 years. However, the short follow-up period and the confounding bias of concomitant site change or capsulectomy procedures in these studies mean that the true benefits of ADM placement are difficult to define exclusively. Consequently, indications for ADM use in revisional contracture management are evolving.
15.6 Conclusion
The role of biofilm formation and the subclinical infection theory in the pathogenesis of CC is well established. In genetically susceptible individuals who are preconditioned to a gram-negative microbiome, the chronic inflammation associated with biofilms may promote clonal proliferation of mutated T cells, implicated in the pathobiology of ALCL. Nonetheless, the sine qua non of CC prevention is meticulous aseptic technique and surgical planning (incision, implant type, and plane) as espoused by the 14 point plan. 34 Although nonoperative strategies such as leukotriene receptor antagonists (specifically zafirlukast) have being studied, their use is adjunctive in established CC at best. Revisional surgery in the management of CC is challenging. Surgical approaches have traditionally involved a combination of capsulectomy (partial/ total) and change of implant plane, pocket, or both as well as implant exchange. This recognizes the importance of biofilm eradication and the site change principle to limit periprosthetic scar tissue and inflammation. Although ADM use has potentially demonstrated some reduction in short-term CC rates, its current indications are evolving.
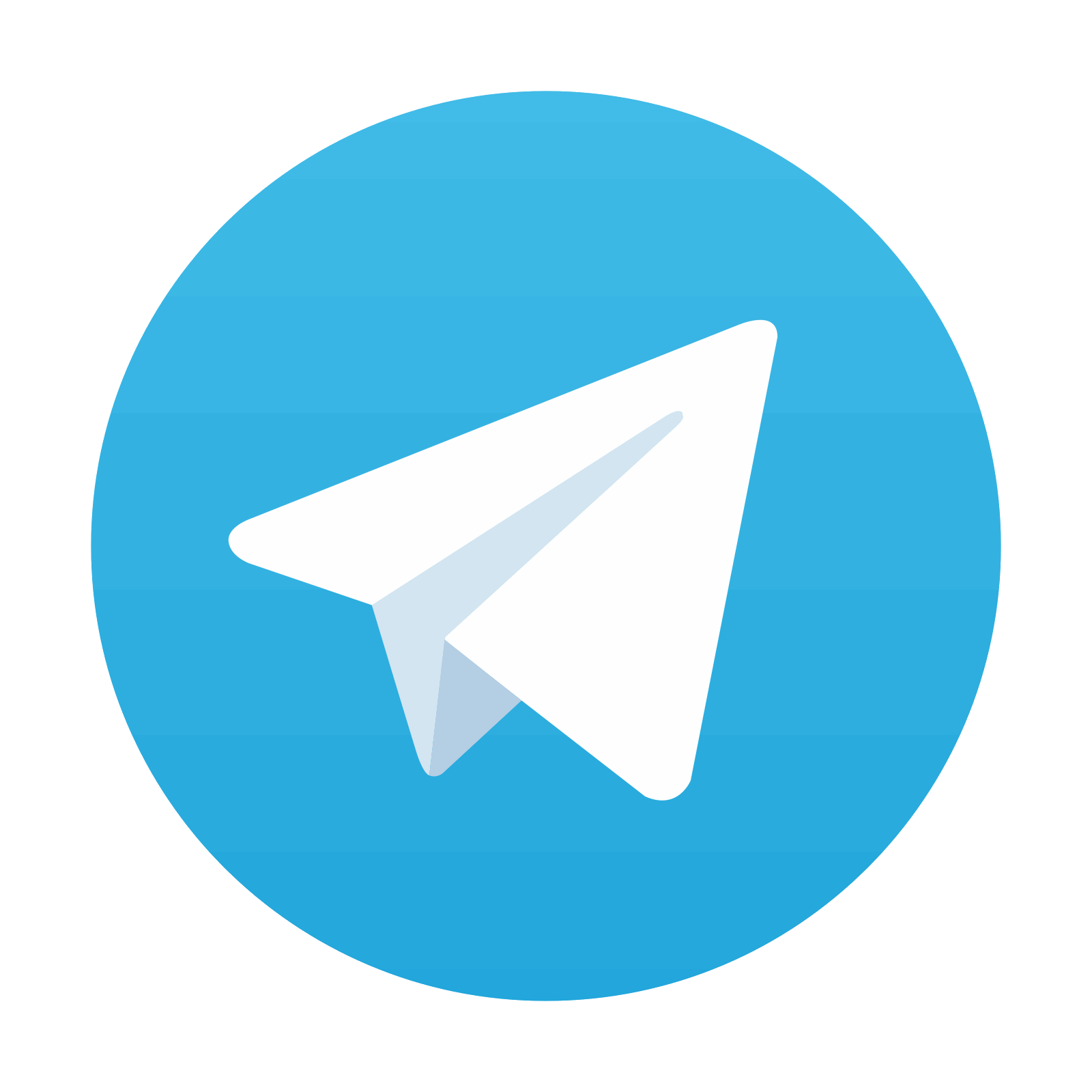
Stay updated, free articles. Join our Telegram channel

Full access? Get Clinical Tree
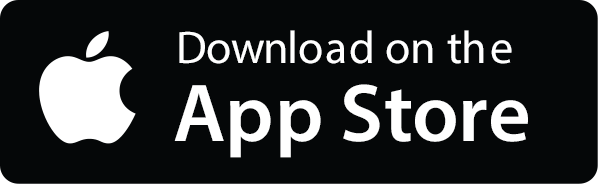
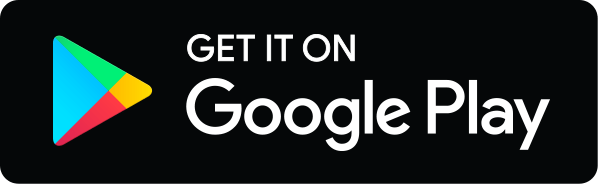