12 Treatment of Vascular Lesions
Key Concepts
Appropriate treatment of vascular lesions requires an understanding of the pathophysiology of the lesion and knowledge of the indications for nonsurgical and surgical options.
Lasers are important tools in the armamentarium of facial plastic and reconstructive surgeons for the treatment of vascular lesions.
Lasers can allow for targeted treatment of vascular facial lesions.
Lasers are selected based on the type of lesion, chromophore content of the tissue, desired effect, and characteristics of the laser, including wavelength and energy density.
Continued advancements in laser technology have improved results of laser treatment of vascular lesions of the head and neck.
Introduction
Historical accounts of vascular lesions stem from ancient Greece, Rome, and Europe. The history of vascular lesions is steeped in negative perceptions. For example, the connotation of the term birthmark, which is often associated with vascular lesions, has historically implied a direct causal relationship between the emotions, thoughts, or behaviors of the mother and the anomaly of the offspring.1 The theory that the mother was in some way responsible for vascular lesions was prevalent until the 18th century and was termed maternal impression.1,2 William Hunter is largely credited with dispelling the unfounded belief in maternal impression.
Advances in medicine have provided understanding of the biological basis for many vascular anomalies. Treatment of vascular lesions has evolved at a rapid pace with increasing knowledge of pathophysiology and technical advancements. One of the greatest strides in the treatment of vascular lesions came with the development of the laser.
Einstein published his paper “On The Quantum Mechanics of Radiation” in 1917, which established the basis for laser theory.3 Subsequently, the first laser was built by Maiman in 1960 and patient treatment began shortly thereafter.4 Goldman performed the first cutaneous laser treatment with ruby and neodymium pulsed laser systems,5 and Patel developed the first CO2 laser in 1964.6 The argon laser became popularized in the 1970s for treatment of cutaneous vascular lesions, and by the early 1980s, the argon laser was the treatment of choice for port wine stains. Following these advancements, the flashlamp pumped pulsed dye laser was introduced in the mid-1980s, and the intense pulsed light laser was developed in 1993. Lasers have flourished since the advent of this technology with the development of new lasers with unique characteristics and expanding clinical applications.7
This chapter discusses vascular lesion classification, treatment options, laser biophysics, laser-tissue interaction, technical aspects of laser treatment, and complications associated with treatment of vascular lesions in facial plastic and reconstructive surgery. This chapter also provides a detailed understanding of common vascular lesions, laser technology, indications and reasons for employment of specific lasers, and practical knowledge related to the technical aspects of vascular lesion treatment.
Background: Basic Science of Procedure
Vascular Lesions
Mulliken and Glowacki are credited with developing the modern classification system for vascular lesions.8–10 Although the original classification schema has undergone necessary changes with advancements in the understanding of underlying pathophysiology, vascular lesions continue to be categorized based on structural components and biological behavior. The general term vascular lesion comprises vascular malformations and hemangiomas.1
Vascular malformations are differentiated from hemangiomas based upon their presence at birth, increasing size with patient growth, and normal rate of endothelial cell growth. Additionally, vascular malformations have a propensity to become darker in color and develop surface thickening with time. Vascular malformations are often subclassified based on their vessel components: capillary vascular malformations (port wine stains) and venous, arterial, lymphatic, and combination lesions.1 Vascular malformations characteristically have large ectatic vessels and lack endothelial hyperplasia.
In contrast, hemangiomas behave like true neoplasms. Hemangiomas have a proliferative phase with rapid growth and endothelial proliferation, followed by an involution phase. Involution of these lesions is a slow process. Hemangiomas are characteristically absent at birth and demonstrate endothelial hyperplasia in ectatic vessels.1
Treatment Options
Treatment of vascular lesions has been revolutionized with the development and advancements in laser technology. When considering appropriate management for each patient and individual lesions, one must consider all available options. Common nonlaser treatment options consist of observation, ligation, excision, cautery, sclerosant therapy, chemotherapy, radiation, steroid therapy, embolization, systemic medications, or a combination of treatments. However, laser technology remains a mainstay in the treatment of vascular lesions because laser selection often allows for targeted and selective photothermolysis. Throughout the following discussion regarding laser characteristics and technical aspects of laser treatment, treatment options will be further delineated in relation to specific lesions.
Laser Biophysics
The word laser was originally an acronym for light amplification by the stimulated emission of radiation. Lasers produce light that is monochromatic (one wavelength), collimated (little divergence), and coherent (identical temporal and spatial phases). Lasers utilize photon energy to achieve these characteristics.
Lasers start with a source of light energy. The photons present in the light strike atoms in the laser medium, which raises electrons to higher energy states. When an atom in the higher energy state is then struck by an additional photon, two photons are emitted. This process is termed stimulated emission. The photons produced will continue to propagate the energy transfer via mirrors within the system ( Fig. 12.1 ). The photons emitted characteristically have the same wavelength, progress in the same direction, and travel in phase.7
Laser–Tissue Interaction
Laser energy can have a variety of effects on tissue, ranging from activation of biochemical substances or stimulation of biological tissues at low energy to coagulation or vaporization of biological tissues at high energy.7 The effect of a laser on a given tissue is a function of the laser wavelength, energy density, and the amount of reflection, scatter, absorption, or transmission of energy that occurs ( Fig. 12.2 ).7

Chromophores are substances that absorb energy at specific wavelengths. The specific absorption patterns of tissues partly determine the most efficacious laser for a given lesion. The chromophore absorbs light energy, which is then converted to heat. Coagulation of tissues occurs at 60 to 70°C and tissue vaporization occurs at 100°C.11 Appropriate laser selection allows for targeted thermal damage in tissue components that absorb a specific wavelength of light. This process is termed selective photothermolysis.11 Another important laser property is wavelength, with deeper tissue penetration occurring with longer wavelengths of light.11 The peak absorption wavelength for oxyhemoglobin, which is found in high concentrations in vascular malformations and hemangiomas, is 577 to 585 nm. Some lasers are used at the wavelength that corresponds to a chromophore absorption peak, whereas others are designed for use at a wavelength that does not correspond to a chromophore absorption peak.

Two important laser parameters are energy density and power density. Energy density (fluence) is a function of the power (intensity) of the laser and the time of exposure. Power density is a function of the power divided by the cross-sectional area of the laser beam (spot size). Thus, by altering power density or time of exposure, the amount of energy delivered by a particular laser can be altered. Additionally, by decreasing the laser spot size, power density is increased.
Energy density (fluence) = Power density × Time
Power density = Power (watts)/Cross-sectional area of laser beam (spot size)
Pulsed energy can also be utilized. This mechanism incorporates regular periods of irradiation (on period) that are alternated with periods of no irradiation (off period). This alternating delivery of laser energy permits heat dissipation during the off period. Thus thermal heat damage to surrounding tissue is minimized. Duty cycle is defined as the ratio of the on interval to the on plus off intervals. Q-switched lasers release high-energy bursts of light for short time intervals using an electro-optical shutter.7 Ultimately, one must match the laser wavelength, spot size, energy density, power, and pulse duration to the tissue of interest and desired outcome.11
Technical Aspects of Procedure
The ideal laser for vascular lesions should have a wavelength that is selectively absorbed by a chromophore in the vascular tissue. The laser should have minimal epidermal absorption to reduce the incidence of scarring and the ability to vary the pulsed laser energy based on the tissue thermal relaxation time. Currently, there are several lasers available for the treatment of vascular lesions based on the key laser characteristics already discussed.
Laser Characteristics
Pulsed Dye Laser
The flashlamp-pumped pulsed dye laser (PDL) was developed in 1989 utilizing the principle of selective photothermolysis. The laser medium is a rhodamine dye, which is excited optically by a flashlamp. Ultimately, this laser produces visible, yellow light.12 PDLs are currently used to treat hemangiomas, port-wine stains, rosacea, and telangiectasias. These lasers have wavelengths of 585 to 600 nm.12,13 The more recent versions of the PDL also utilize a dynamic cooling device that reduces the risk of epidermal injury.14,15
The spot sizes of these lasers vary from 7 to 12 mm and have higher peak fluence potentials. These alterations have been developed for more effective treatment of the deeper vessels found in hemangiomas and port-wine stains.12,16 Additionally, longer pulse durations of 1.5 to 40 msec have allowed for telangiectasia treatment without the development of significant purpura.12,16 A new PDL (Perfecta, Candela, Wayland, MA) has incorporated a pulse structure where each macropulse is subdivided into eight micropulses. This technology allows for single-pass treatment with delivery of higher total fluencies with less risk of purpura ( Fig. 12.3a,b ).17,18
Most patients develop variable erythema and/or purpura following PDL treatments. This may last for 7 to 10 days. Furthermore, patients may develop hyperpigmentation, hypopigmentation, or scarring.12
Intense Pulsed Light Laser
Intense pulsed light (IPL) devices produce 500 to 1,200 nm noncoherent light. Cutoff filters are used to produce wavelengths that target vascular lesions. Likewise, IPL system filters can eliminate shorter wavelengths to allow for greater dermal penetration with light of longer wavelengths.12 IPL systems have been used to treat port-wine stains, hemangiomas, and telangiectasias.12,19,20 In comparison with the PDL, IPL treatments require more time and have greater associated risks because additive heating is incurred with successive passes and longer pulse durations.12
The primary usage for IPL systems is treatment of telangiectasias. Selected wavelengths between 470 and 1,400 nm corresponding to absorption peaks of oxyhemoglobin are used to specifically target vessels. The range of wavelengths allows for treatment of vessels at various depths and greater energy penetration into the tissue.12 Newer systems incorporate cooling with chilled sapphire windows.
Potassium Titanyl Phosphate Laser
The potassium titanyl phosphate (KTP) laser is a frequency-doubled (wavelength halved) neodymium: yttrium-aluminum-garnet (Nd:YAG) laser. This is accomplished by passing the laser energy though a KTP crystal. The resultant light is green with a wavelength of 532 nm, corresponding to a hemoglobin absorption peak. The laser energy is delivered to tissue via a fiber. If the fiber is not in contact with the tissue, vaporization and coagulation occur. Lower settings are typically used for coagulation. If the fiber is in semi-contact with the tissue, cutting occurs. The KTP laser has a wide range of pulse durations, from 1 to 100 msec. The KTP laser has a shallow penetration depth. Accordingly, this laser is often utilized for vascular lesions given its absorption patterns (e.g., telangiectasias). Additionally, there is low risk of purpura, edema, or crusting.12,21 Atrophic scarring is a concern with the KTP laser. One of the common KTP lasers in use is the Varilite, a 532 nm KTP and 940 nm diode pumped laser (Iridex, Mountain View, CA).
Red and Infrared Lasers
The alexandrite (755 nm), diode (532 nm, 800 to 940 nm), and Nd:YAG (1,064 nm) lasers are in the red and near-infrared (IR) range. These lasers have been used to treat reticular veins, port-wine stains, and deep vessels in vascular malformations. Red and IR lasers are effective for these lesions because they have wavelengths that target peaks of deoxyhemoglobin in the near-IR range (700 to 1,200 nm), achieve deeper penetration, and allow for higher fluence.12
The alexandrite laser is a flashlamp-pumped solid-state laser in the red spectrum of light (755 nm). This laser is absorbed by melanin and achieves deep penetration. The alexandrite laser has been used with significant improvement for hypertrophic port-wine stains. Some authors have reported using settings of 3 mm spot size, fluence range from 30 to 85 J/cm2, and dynamic cooling22; others have used settings of 8 mm spot size for the initial treatment of deep malformations.12 Li et al also demonstrated the effectiveness of the long-pulse pulsed alexandrite laser for hypertrophic, purple port-wine stains.23 The authors did note that this laser can cause dyspigmentation, but no scarring was noted.23

The diode laser uses superconducting materials that are directly coupled to fiberoptic delivery devices. These lasers result in varying wavelengths depending on the material used. Diode lasers are efficient, converting electrical power to light at efficiencies of 50%, which allows for less heat production and power input.21
The Nd:YAG emits a wavelength of 1,064 nm, in the near-IR spectrum. The laser medium is neodymium:yttrium-aluminum-garnet. Pigmented tissue is selectively targeted with this laser because it penetrates up to 4 to 6 mm for treatment of deeper vessels ( Fig. 12.4a,b ).24 The depth of penetration of the Nd:YAG is related to its scatter, which is much greater than that with the CO2 laser. Yang et al found the long-pulsed Nd:YAG laser to be equally effective as PDL for the treatment of port-wine stains in a series of 17 patients.25 The authors found that purpura lasted longer following PDL treatments, but the Nd:YAG caused greater perivascular and epidermal injury with scarring noted in one patient.25
Depressed scarring and hyperpigmentation are potential complications associated with the Nd:YAG laser.12 Cooling devices assist in minimizing these risks. In comparison with the near-IR diode laser, the Nd:YAG laser has deeper penetration, incurs less surrounding epidermal injury, and may be used more safely in darker pigmented individuals.12
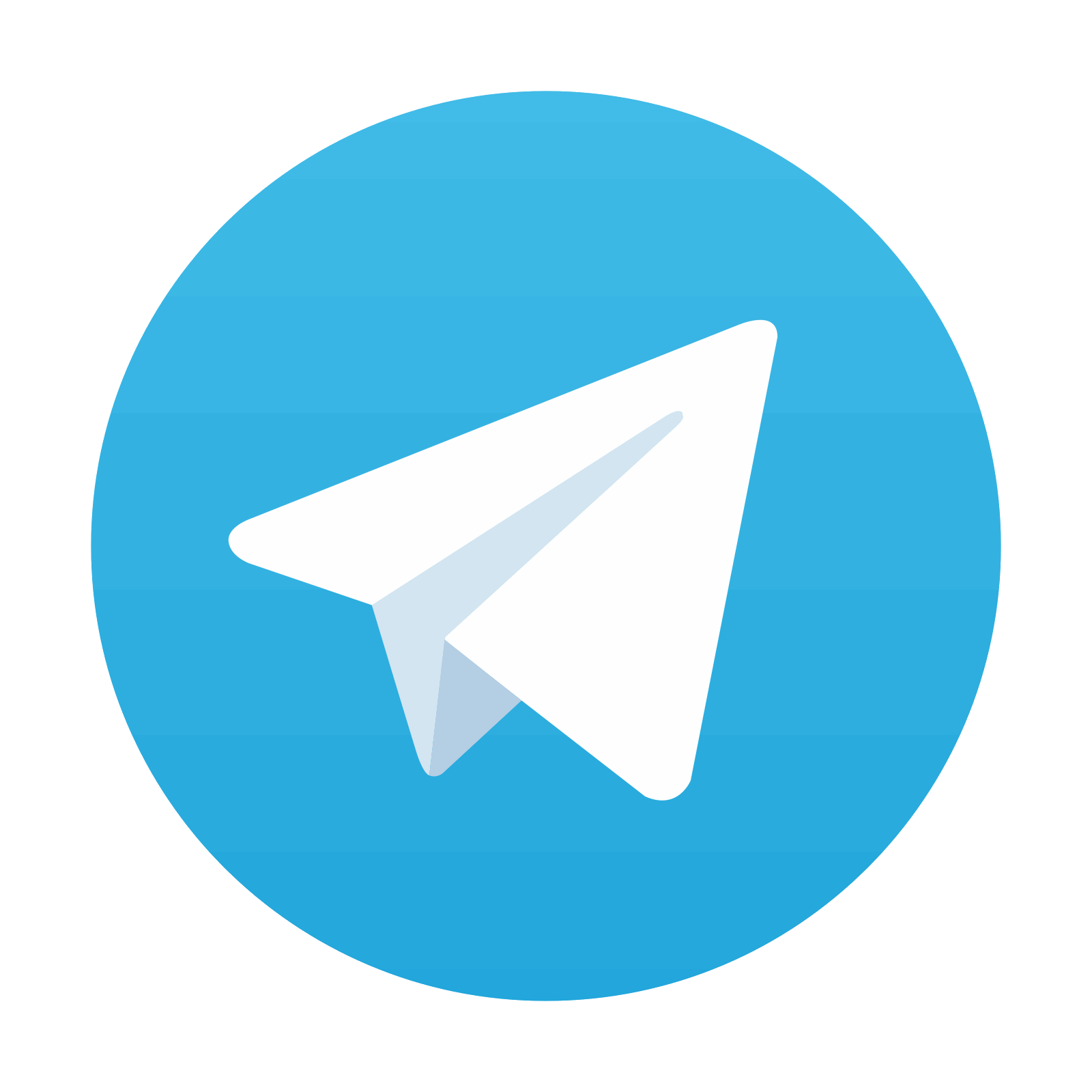
Stay updated, free articles. Join our Telegram channel

Full access? Get Clinical Tree
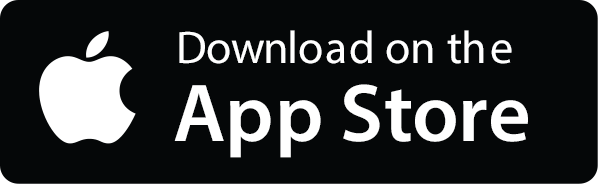
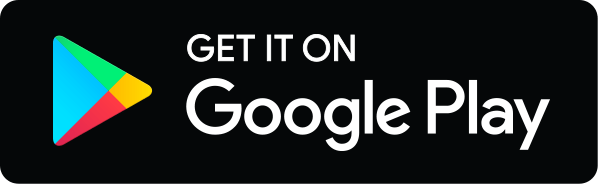
