23 Vascular territories
Synopsis
This chapter provides an overview of the angiosome concept and reviews the vascular anatomy of the body. The historical perspective summarizes the major progress in understanding the vascular basis and clinical applications of flap in reconstructive surgery
The anatomical basis of angiosomes, choke anastomotic vessels, arterial territories, and venous drainage of the human body are summarized. The neurovascular territories of skin and muscle are described. Comparisons with other species highlight the consistent features of vascular anatomy of the human body and illustrate the need to be aware of the vascular anatomy of animal flap models
The vascular anatomy of skin, muscle, and bone of each region of the body is discussed with an emphasis on flap design, avoiding surgical complications and providing an overview of angiosomes of the body
The general concepts of the vascular supply to tissues of the body are reviewed. The importance of these concepts to flap design is highlighted with clinical examples. These concepts also provide the basis for interpreting physiologic and pathologic events in skin flaps
The overall architecture of the vasculature of the human body is consistent but there is significant variability which requires a versatile operative plan for successful flap design. Methods of preoperative assessment of vascular anatomy and types of flaps, including skin, fasciocutaneous, musculocutaneous and perforator flaps, are reviewed. The basis of the delay phenomenon and procedure is explored.
Introduction
In 1977, Converse1 stated that “there is no simple and all-encompassing system which is suitable for classifying skin flaps.” It is now generally agreed that the anatomical vascular basis of the flap provides the most accurate approach for classification. Specific, anatomically based nomenclature simplifies communication between surgeons and allows for the advancement of the field of plastic and reconstructive surgery. The main named source vessels throughout the body provide a useful road map for the description of flaps.
The vascular architecture of the body is arranged anatomically as a continuous series of vascular loops, like the tiers of a Roman aqueduct, that increase in number while their size and caliber decrease as they approach the capillary bed (Fig. 23.1). The reverse situation occurs on the venous side. This anatomic arrangement of the vascular “skeleton” is shown beautifully in the corrosion cast studies of newborn babies performed by Tompsett2 that reside in the Hunterian Museum at the Royal College of Surgeons in London (Fig. 23.2). Note how the main arterial loops hug the bony framework and the secondary arcades follow the intermuscular and intramuscular connective tissue framework. The “keystones” of these arcades are represented usually by reduced-caliber (choke anastomotic) arteries and arterioles, matched on the venous side by avalvular (oscillating) veins that permit bidirectional flow. Choke arteries and avalvular veins have an essential role in controlling this pressure gradient across the capillary bed (Fig. 23.1).
Historical perspective
Plastic surgery evolved as a specialty in Europe and North America to restore the mutilated victims of the two World Wars. With artistic flair and geometric precision, tissues were advanced and rotated. These random flaps were transposed locally and dispatched to distant sites on limb carriers, only to be rebuffed on occasion by necrosis. Gillies often lamented that “plastic surgery is a constant battle between blood supply and beauty.”3 Gradually, rigid length-to-breadth flap ratios were calculated for different regions of the body because most of the flaps were designed without a precise knowledge or appreciation of the vascular supply of flaps. The flaps designed were “random” since it was not appreciated that the vascular supply was crucial to flap survival.
Unfortunately, this anatomic information was available but overlooked. In 1889, Manchot4 performed the first examination of the vascular supply of the human integument. His treatise, Die Hautarterien des menschlichen Körpers [The Cutaneous Arteries of the Human Body], was initially published in German and later translated to English.5 Manchot identified the cutaneous perforators, assigned them to their underlying source vessels, and charted the cutaneous vascular territories of the body (Fig. 23.3). He did not have the advantage of radiography since Röntgen was not to make his discovery until several years later. Nevertheless, the accuracy of Manchot’s work has mostly stood the test of time.
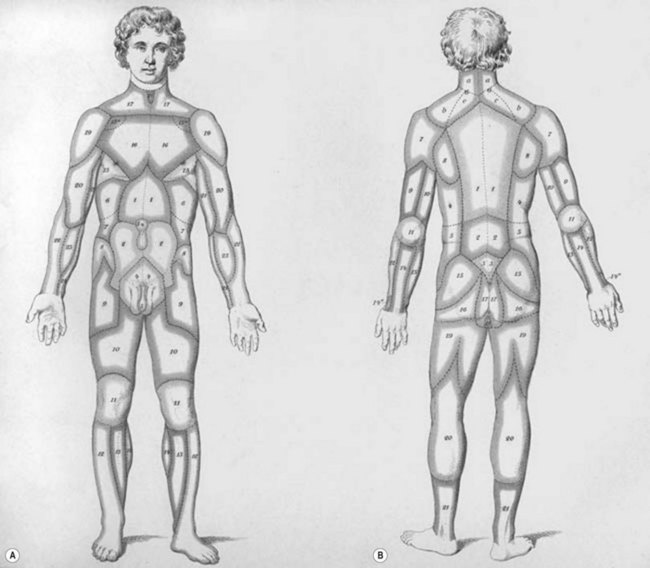
Fig. 23.3 Carl Mancot’s vascular territories of the human integument.
(From Manchot C. Die Hautarterien des menschlichen Körpers. Leipzig: FCW Vogel; 1889.)
In 1893, Spalteholz6 published an important paper on the origin, course, and distribution of the cutaneous perforators in adult and neonatal cadavers. He performed arterial injections of gelatin and various pigments. The soft tissues were fixed in alcohol and subtracted in xylol, and the resulting vascular network was embedded in Canada Balsam. Spalteholz’s main study concentrated on the detailed circulation of the skin. He made an important distinction between direct cutaneous vessels, which supply the skin, and indirect cutaneous vessels, which are terminal branches of vessels supplying the deeper organs, especially the muscles. A detailed account of this work was published by Timmons7 in a review of the landmarks in the anatomic study of the skin’s blood supply.
The next major study was performed by Salmon,8,9 a French anatomist and surgeon in the 1930s. Manchot had defined approximately 40 cutaneous territories that excluded the head, neck, hands, and feet. Salmon knew of Manchot’s studies and set out to reappraise his work. Aided by radiography, he was able to delineate the smaller vessels of the cutaneous circulation and charted more than 80 territories encompassing the entire body (Fig. 23.4). Salmon noted the interconnections that exist between perforators, and his observation of the density and size of the vessels in different regions of the body led him to define what he called the hypervascular and hypovascular zones. His work has become available in English.10 Ironically, in the preface to Michel Salmon’s book on the cutaneous vascular anatomy published originally in 1936,8 Raymond Grégoire stated: “This new work by Michel Salmon is a painstaking study that no surgeon from now on can ignore and few anatomists would have had the courage to undertake.” Indeed, if we had heeded this advice, plastic surgery would have evolved much earlier!
In 1975, Schafer,11 published an important study on the arterial and venous anatomy of the lower extremity. Scribtol and an ink–serum mixture were injected into the lower limbs of adults and into the entire circulation of fetal and neonatal cadavers. Schafer concluded that most cutaneous arteries emerge in rows from the intermuscular septa or occasionally from the intramuscular septa. In addition, he highlighted the two systems of perforating veins: the venae communicantes, large veins that pierce the deep fascia and connect the superficial venous plexus to the deep venous system; and the venae comitantes, small, usually paired veins that accompany the cutaneous arterial perforators.
Early last century, advances on the clinical front gave significance to the work of these great anatomists. In 1906, Tansini12 reported a latissimus dorsi flap supplied by the thoracodorsal artery. In 1919, Davis13 published Plastic Surgery and introduced many of the chapters with illustrations from Manchot’s book. In 1921, Blair14 described a forehead flap based on the superficial temporal vessels, and in 1929, Esser published Artery Flaps.15 In 1937, Webster16 again cited the work of Manchot when he described a long, bipedicled thoracoepigastric flap based on named arteries that extended from the groin to the axilla. Shaw and Payne17 used the clinical information available in wartime to provide one-stage direct flaps for hand reconstruction. In 1965, Bakamjian18 drew attention to the long paramedian perforators of the internal thoracic system.
The 1970s witnessed the beginning of the “anatomic revolution.” McGregor and Morgan19 differentiated between large flaps based on a known axial blood supply and those based on random vessels. Daniel and Williams20 reappraised the work of Manchot and others and classified the cutaneous arteries into direct cutaneous and musculocutaneous vessels.
Studies on the free flap by Taylor and Daniel21,22 were published in 1973, and a few years later the musculocutaneous flap was revived by McCraw23–25 and Mathes and Nahai.26 Both procedures demanded a precise knowledge of the vascular supply of the tissue transfers. In the search for new donor sites for tissue transfer, surgeons returned to the dissection room. In the 1980s the pedicled musculocutaneous flap and the free microvascular transfer gained popularity and their use became common. However, on occasion there was a tendency for the techniques to neglect the aesthetic side of plastic surgery. The results could sometimes be what McDowell27 described as “globs and blobs.”
To escape from the hamburger of muscle and skin, surgeons soon rediscovered that blood vessels follow fascial planes and, starting in the 1980s, there was a much greater awareness of the fasciocutaneous flap.28 With this development, there has been an explosion of new terms and new classifications of the cutaneous circulation. The thesaurus of flaps now includes a bewildering array of terms including axial, random, direct cutaneous, musculocutaneous, fasciocutaneous, supercutaneous, septocutaneous, chimeric, retrograde, antegrade, and perforator. Indeed, there has been an attempt to classify flaps into no less than 10 types and subtypes on the basis of the origin of the cutaneous perforators.29 In many ways, these flap classification terms are simply different expressions of the basic cutaneous architecture that Manchot published in 18894 and Salmon reported in 1936.8 In an effort to standardize the literature and add clarity to descriptions of flaps, we have previously suggested using the name of the main source vessel to define the flap.30,31 Cormack and Lamberty32 published a book, The Arterial Anatomy of Skin Flaps, that contains a concise appraisal of the history, anatomy, and clinical aspects of skin flap surgery. The relationship of vessel size to vascular territory and axiality of vessels in the fasciocutaneous system has also been described by Cormack and Lamberty.33
The vertical rectus abdominis musculocutaneous flap was described by Michael Drever in 1977.34 The transverse rectus abdominis muscle (TRAM) flap reported initially by Hartrampf et al.35 demonstrated that the skin paddle survives based on the musculocutaneous perforators of the superior epigastric vessels. The pedicled and, later, the free TRAM flap became very popular for postmastectomy breast reconstruction. As the anatomy of the musculocutaneous perforators became better understood,36,37 surgeons harvested less of the rectus abdominis muscle in order to preserve function of the muscle. Investigators pushed the boundaries once again with the rediscovery of the perforator flap, whereby a large abdominal skin flap can be raised with preservation of the underlying rectus muscle and hence reduce the potential problems of an abdominal incisional hernia and abdominal wall weakness. This gradual change in flap design gave rise to the deep inferior epigastric artery perforator (DIEAP) flap based on perforators of the deep inferior epigastric vessels.38 This evolution of autogenous reconstruction of the breast – from pedicled TRAM flap, to free TRAM flap, to muscle-sparing free TRAM flaps, to DIEAP flaps – reflects a greater awareness of the precise anatomy of the underlying musculocutaneous perforators and an attempt to reduce donor site morbidity and improve results. As knowledge of individual perforators to the skin improved, the spectrum of flap options increased dramatically.39 There has been a virtual explosion in published surgical work regarding perforator flaps.
The recent enthusiasm for perforator flaps has heightened awareness of the vascular supply to tissues of the body.40 Instead of 10–20 named arterial flaps in the body we now have a vast array of flap options based on any one of the over 400 arterial perforators of the body. This heightened awareness has led to greater understanding of the seemingly infinite possibilities for customized flap options.
Vascular anatomical research
Angiosome
The angiographic studies produced by Salmon using lead oxide, gelatin, and water were exceptional, however, modifications of the technique have further improved results.41,42 In particular, reducing the concentration of lead oxide has improved computed tomographic (CT) angiographic anatomic studies.43 A review of vascular injection techniques reveals the wide array of techniques available for investigation.44
Initially, cadaver injection studies to study the vascular anatomy of the human integument and other structures utilized intra-arterial injections of radiopaque substances such as barium sulfate or lead oxide or visible substances such as latex and ink. Depending on the specific study, the area of tissue of interest was then dissected and radiographed. As the radiographic film quality improved, the quality of the image of the small blood vessels improved. However, studies using simple radiographs have largely been replaced by CT techniques.43,45,46 These investigations were conducted in fresh cadavers. In the majority of studies, the anatomical question was problem-oriented in a desire to provide a surgical solution to the individual patient’s needs. We have performed a large number of fresh cadaver studies, investigating various regions, tissues, and combinations of tissues. This has included an investigation of the entire integument and underlying deep structures in a series of total body studies of the arteries,47 which led to the angiosome concept, discussed in detail in a later section. This was followed by studies of the veins48 and the neurovascular territories of the body49 and detailed studies of the angiosomes of the forearm,50 the leg,51 and the head and neck,52 as well as a comparative study of a series of mammals.53 As well, we have performed detailed analyses of numerous muscle flaps including sartorius,54 rectus femoris,55 gracilis,56 pectoralis major,57 and skin flaps, including the reversed sural artery flap,58 thoracodorsal artery perforator flap,59 profunda femoris artery perforator flap,60 and the superior and inferior gluteal artery perforator flaps.61
The investigations initially involved an analysis of various regions of the body to define possible donor sites for free skin flap transfer.62 The studies subsequently focused on other tissues and included the anatomic basis for the transfer of bone,63 nerve,64 and certain muscles.21,65 Encouraged by the success of some of the resulting clinical procedures, the authors expanded the research to investigate composite units of tissue, supplied by a single vascular system. Units of skin and tendon,66 muscle with nerve,67 and skin, muscle, and bone68–70 were analyzed. It was from this work that the angiosome concept germinated. Various regions, including the anterior abdominal wall,21,36,65,71 the anterior thorax,72,73 the lower limb, and the upper limb, were studied. The results added strength to the angiosome concept of the blood supply and revealed the interconnections that exist at all levels between adjacent vascular territories, a relationship that is evident throughout the body.74
In cadaver vascular research, various techniques can be used to identify and study the area of interest. In the past, the integument (skin and subcutaneous tissue) was removed, and the sites of emergence of the dominant cutaneous perforators (0.5 mm or more) were identified on the surface of the deep fascia with lead beads. Currently, individual perforators are easily identified on CT angiography (CTA). Approximately 400 cutaneous perforators on average were identified per body.40,47 The three-dimensional branching pattern of main source vessels can be identified. Previous workers, including Salmon, had made topographic boundary incisions to remove areas of skin, particularly in the lines of the groins, axillae, neck, and limb joints (Fig. 23.5). These junctional regions are of great clinical importance, and for this reason the incisions were designed to retain their continuity wherever possible. In our current techniques, using CTA, the incisions utilized for dissection are not as crucial since the pathway and branches of individual vessels are clearly documented prior to dissection.
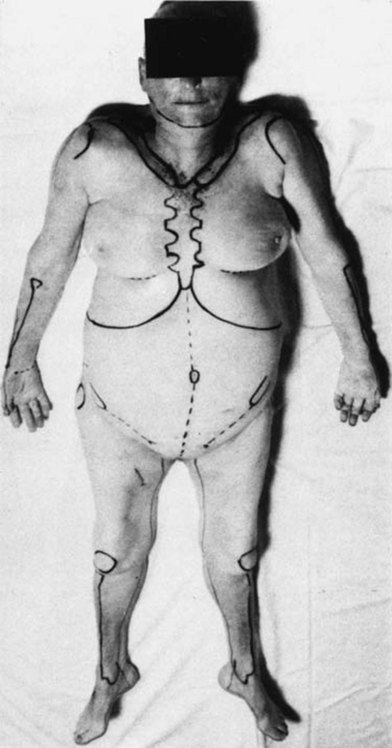
Fig. 23.5 Cadaver with body landmarks and incision lines marked.
(From Taylor GI, Palmer JH. The vascular territories [angiosomes] of the body: experimental study and clinical applications. Br J Plast Surg. 1987;40:113.)
In the original studies of the vascular supply of tissues of the body, the integument was radiographed, and a montage of the entire cutaneous circulation was constructed in “plan view” (Figs 23.6, 23.7).47 Although Manchot and Salmon described the origin and course of the cutaneous arteries, and Salmon9 made a separate study of the individual muscles, neither worker illustrated the course of the arteries between the deep tissues and the skin. Therefore, the skin and subcutaneous tissues were cut into parallel strips and placed on their side, and radiographs were taken to provide “elevation views” of the vessels in different regions of the body (Fig. 23.8). Current CTA techniques allow a far more detailed three-dimensional appraisal of the vascular anatomy of tissues (Fig. 23.9).
All cutaneous perforators of diameter greater than 0.5 mm were traced to their underlying source arteries. The results were averaged from each cadaveric study and plotted on a diagram of the body (Fig. 23.10). Subsequently, investigations were expanded to map out the venous territories (venosomes) of the body along with the neurovascular territories of the skin and muscle.48 These results have led to an overall picture of the vascular territories of the entire body. The remainder of this section gives a brief overview of the arterial, venous, and nervous territories of the body.
Arterial territories
The arterial network of the body forms a continuous interlocking arcade of vessels throughout each tissue and throughout the body, linked together as loops of vessels, often of reduced caliber. The course of the cutaneous perforators depends on the proximity of the source artery to the undersurface of the deep fascia. As Michel Salmon noted in 1936,8 arteries supply branches to each tissue that they pass, including the intermuscular and intramuscular septa, fascia, nerves, and tendons. Arteries generally fall into two groups, direct and indirect (Fig. 23.11). In our anatomical dissections, it is clear that there is great variability in the exact course and size of individual vessels. The direct cutaneous vessels pass between the deep tissues before piercing the outer layer of the deep fascia. They are usually the primary cutaneous vessels, their main destination being the skin. They tend to supply the skin with larger-diameter vessels which have a large vascular territory (e.g., circumflex scapular artery). The direct branches include direct cutaneous vessels (sometimes called axial vessels) and septocutaneous vessels. The indirect vessels can be considered the secondary cutaneous supply. They emerge from the deep fascia as terminal branches of arteries which supply the muscles and other deep tissues. The majority of indirect branches are musculocutaneous perforating branches which emerge to supply the skin. In fact, there is usually significant variability in the distribution of direct and indirect vessels and their vascular territory from individual to individual. There is a vast interconnected network of direct and indirect arteries which supply the skin. The vascular territories of individual perforators vary and tend to be reciprocal with adjacent arterial vascular territories according to the so-called law of equilibrium, described by Salmon and supported by our work.
The direct cutaneous perforators pierce the deep fascia near where it is anchored to bone or the intermuscular and intramuscular septa (Fig. 23.10). These lines and zones of fixation also correspond to the fixed skin areas of the body. From these points, the vessels flow toward the convexities of the body surface, branching within the integument. The wider the distance between the cavities and the higher the summit, the longer the vessel (Fig. 23.8). The size and density of the direct perforators also vary in different regions. For example, in the head, neck, torso, arm, and thigh, the vessels are larger, longer, and less numerous. In the forearm, leg, and dorsum of the hands and feet, the vessels tend to be smaller, shorter, and more numerous. In the palms of the hands and the soles of the feet, where the skin is fixed, there is a high density of smaller perforators. Hence, the primary supply of each cutaneous territory varies between source arteries. Each of these territories also has indirect perforators.
In the scalp and limbs, where the skin is relatively fixed to the deep fascia, the larger vessels hug that surface. They course on the deep fascia for a considerable distance in the loose areolar layer that separates them from the subcutaneous fat (Fig. 23.8). This is especially true when a perforator accompanies a cutaneous nerve.
In the loose skin areas of the body, the direct cutaneous vessels course for a variable distance parallel to the deep fascia. They are more intimately related to the undersurface of the subcutaneous fat, however, being plastered to it by a thin fascial sheet that separates them on their deep surface from a plexus of smaller vessels. This plexus lies in loose areolar tissue on the surface of the deep fascia. It is formed by branches that arise from the direct perforators as they pierce the deep fascia and the connections these branches make with smaller indirect perforators. The large direct perforators then pierce the subcutaneous layer. They ascend within the superficial fascia (subcutaneous fat) to reach the rich subdermal plexus, where they travel for considerable distances (Fig. 23.8).
Venous drainage
The cutaneous veins also form a three-dimensional plexus of interconnecting channels throughout the body (Fig. 23.12). There are valved segments in which valves direct flow in a particular direction, and there are avalvular segments where no valves are present. The avalvular or oscillating veins allow bidirectional flow between adjacent venous territories. They connect veins whose valves may be oriented in opposite directions, thus providing for the equilibration of flow and pressure. Indeed, there are many veins whose valves direct flow initially in a distal direction, away from the heart, before joining veins whose flow is proximal. An example of this is the superficial inferior epigastric vein that drains the lower abdominal wall integument toward the groin. In some regions, valved channels direct flow radially away from a plexus of avalvular veins, for example, in the venous drainage of the nipple–areola complex. In other areas, valved channels direct flow toward a central focus, as seen in the stellate branches of the cutaneous perforating veins of the limbs.
In general, venous anatomy parallels arterial anatomy (Fig. 23.13). From dermal and subdermal venous plexuses, the veins collect either into horizontal large-caliber veins, where they often relate to cutaneous nerves and a longitudinal system of chain-linked arteries, or alternatively in centrifugal or stellate fashion into a common channel that passes vertically down in company with the cutaneous arteries to pierce the deep fascia. Thereafter, the veins travel with the direct and indirect cutaneous arteries, draining ultimately into the venae comitantes of the source arteries in the deep tissue.
In the torso, the pattern of arcades is conspicuous (Fig. 23.13); the parent veins are oriented as longitudinal and transverse arcades that match the pattern of the source artery. Distinct territories are evident. Where choke arteries define the arterial territories, they are matched by oscillating veins in the venous network. The existence of venae comitantes is variable.
Within the muscle, the intramuscular venous network mirrors that of the arterial side. Where arterial territories are linked by choke arteries or true anastomotic arteries without changing caliber, the venous territories of the muscles, which drain in opposite directions, are linked by avalvular oscillating veins. Broadly, the muscles can be classified into three types on the basis of their venous architecture. Type I muscles have a single venous territory that drains in one direction. Type II muscles have two territories that drain from the oscillating vein in opposite directions. Type III muscles consist of three or more venous territories that drain in multiple directions (Fig. 23.14).
The extramuscular veins are of two types. The first group consists of the efferent veins. They contain valves and drain the muscles to their parent veins. The other group consists of the afferent veins. They are derived from the overlying integument as musculocutaneous perforators or from adjacent muscles (Fig. 23.14).
Neurovascular territories
In our anatomical studies of neurovascular territories, fresh human cadavers were injected with a radiopaque lead oxide mixture, and the nerves were dissected and labeled with fine computer wire.49 The nerves and vessels were then segregated by subtraction angiography.
Each cutaneous nerve is accompanied by an artery, but the relationship is variable. Some of the arrangements seen in the integument are shown in Figure 23.15. In each case, either a long artery or a chain-linked system of arteries “hitchhikes” with the nerve.
When the cutaneous nerve and artery appear at the deep fascia together, their relationship is often established early (e.g., the lateral intercostal neurovascular perforators on the torso or the saphenous system in the lower limb). However, the nerve sometimes pierces the deep fascia at a point remote from the emergence of its associated artery (e.g., the lateral cutaneous nerve of the thigh and the superficial circumflex iliac artery below the inguinal ligament; Fig. 23.16). Alternatively, the nerve leaves one vascular system with which it is traveling in parallel to cross the path of another (e.g., the lateral intercostal nerve, which courses initially with its artery and then leaves it to meet the superficial inferior epigastric vessel). In many of these cases, secondary or tertiary branches of the artery often peel off to accompany the nerve (Fig. 23.16). Sunderland noted that each peripheral nerve is abundantly vascularized by a “vascular net” of a series of nutrient arteries entering the nerve at different levels.75
1. The nerves follow the connective tissue framework. Dissection showed the motor nerves coursing in the connective tissue sheath from its origin at the nerve trunk to the neurovascular hilum of the muscle. Thereafter, the nerve and its branches follow the intramuscular connective tissue to reach the muscle bundles.
2. The nerves are economical. As in the integument, the direct course of the motor nerves is in stark contrast to the wandering pattern of the vessels. The nerves take the shortest extramuscular and intramuscular routes compatible with the function of each muscle.
3. Neurovascular relations vary with the muscle, the extramuscular course, and the intramuscular branching of the nerves and the vessels. Some muscles have a single nerve supply; others receive multiple motor branches. All receive multiple arterial pedicles. However, despite the variables, certain observations can be made:


Neurovascular anatomy of muscles of the body
Several methods have been used to classify muscles on the basis of morphology, function, blood supply, or nerve supply (Table 23.1). We have classified muscles of the body according to their most common pattern of innervation (Fig. 23.17). The pattern of neurovascular anatomy of the muscles influences the way a whole muscle or segment of muscle can be harvested as a functioning muscle microvascular transfer. It is possible to subdivide certain muscles, based on the neurovascular anatomy, into separate neurovascular units if each segment has an individual vascular pedicle. Clinically, serratus anterior, latissimus dorsi, gracilis, and rectus femoris are often used in this way, taking a portion of the muscle with their motor nerve and blood supply.55,56
• Type I. The muscle is supplied by a single motor nerve that divides usually after entering the muscle (Fig. 23.18). Multiple vascular pedicles supply each muscle and form a continuous network throughout the tissue. It is possible in each case to remove a vascularized segment of muscle with its nerve supply and yet leave viable muscle in situ.
• Type II. A single motor nerve supplies each of the muscles in this group, but this time the nerve divides before entering the muscle. Muscles in this group include the deltoid (Fig. 23.18), gluteus maximus, trapezius, vastus lateralis, serratus anterior, and flexor carpi ulnaris.
• Type III. Multiple motor nerve branches derive from the same nerve trunk (Fig. 23.18). Once again, it is possible to subdivide each muscle into separate functional units because of the multiple vascular pedicles as well as the several nerve branches. Gastrocnemius is often split in this way, taking one head for reconstruction, leaving behind the other functional unit with its neurovascular supply attached.
• Type IV. Multiple motor nerves are derived from different nerve trunks (Fig. 23.18). It is apparent that each muscle can be subdivided anatomically into several functional units because of the multiple, often segmental neurovascular pedicles. Indeed, several of these muscles are formed developmentally by the fusion of adjacent somites (e.g., rectus abdominis and internal oblique).
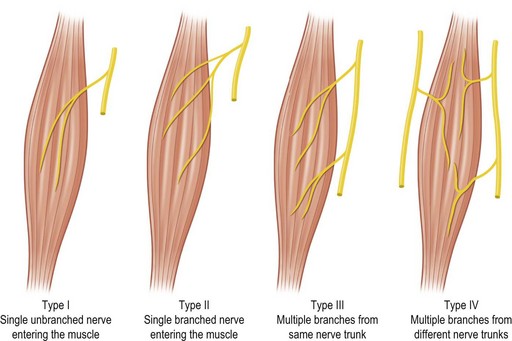
Fig. 23.17 Classification of muscle on the basis of nerve supply.
(From Taylor GI, Gianoutsos MP, Morris SF. The neurovascular territories of the skin and muscles: anatomic study and clinical implications. Plast Reconstr Surg. 1994;94:1.)
Comparative anatomy
In the course of parallel investigations to describe animal models for vascular studies, it was noted that there are close similarities in vascular anatomy between animal species. However, there are also important differences of which investigators should be aware. The purpose of this section is to provide a more complete picture of the vascular territories. As we shall see, the angiosome concept can be applied equally to other members of the animal kingdom as well as to humans.76
When the radiographs of four animals are reviewed (Fig. 23.19), it is obvious there is a marked dissimilarity in the vasculature of the integument between them and that of the human (Fig. 23.7). Surprisingly, however, this is in marked contrast to the dramatic similarity of the radiographs of deep tissue of the mammalian torsos (Fig. 23.20).
The similarity in vasculature of the deep tissue is not confined to the anterior torso. Certain muscles between species have a remarkably similar vascularity, as can be seen in Figure 23.21.
It appears that the vascular blueprint of the deep tissues of the torso of mammals remains relatively constant. It is simply enlarged from the fetus to the adult and from small to large mammals. The reason for this may be that the functional requirements of the torso are the same in each mammal, that is, respiration, protection of the viscera, and aid in removal of the contents. Beyond the deep tissues of the torso, it appears that the vasculature of the overlying integument, the head and the neck, and the limbs has been modified to meet the functional demands of each species, as Hunter77 predicted more than 200 years ago.
An underlying theme of the vascular architecture is that of vascular loops and arcades. Comparison of the vascular architecture in the human with that of other animals and other species reveals a similar arrangement. In loose-skinned animals, the arcades in the integument are stretched over long distances (Fig. 23.18). In the wings of insects and in the leaves of plants, the “veins” assume a pattern of interconnecting arcades similar to those of the intestinal mesentery.
The angiosome concept
Following a review of the works by Manchot and Salmon, along with the results of our total body studies of blood supply to the skin and the underlying deep tissues, it has been possible to segregate the body anatomically into three-dimensional vascular territories named angiosomes. These three-dimensional anatomic territories are supplied by a source (segmental or distributing) artery and its accompanying vein or veins that span between the skin and the bone (Figs 23.22–23.24). Each angiosome can be subdivided into matching arteriosomes (arterial territories) and venosomes (venous territories). Forty of these territories were initially described,73 but subsequent investigation has led to many of these territories being subdivided further into smaller composite units and revealed some that do not reach the skin surface. In a later study, 61 vascular territories were identified.40 Recent work has illustrated no fewer than 13 angiosomes of the head and neck, originally mapped as eight supplied by branches of the external carotid, internal carotid, and subclavian arteries.52 The angiosome concept indicates that the three-dimensional block of tissue is supplied by a major source artery and its accompanying vein(s), but it is important to note that the angiosome itself is divisible depending on the branching pattern of the source vessel.
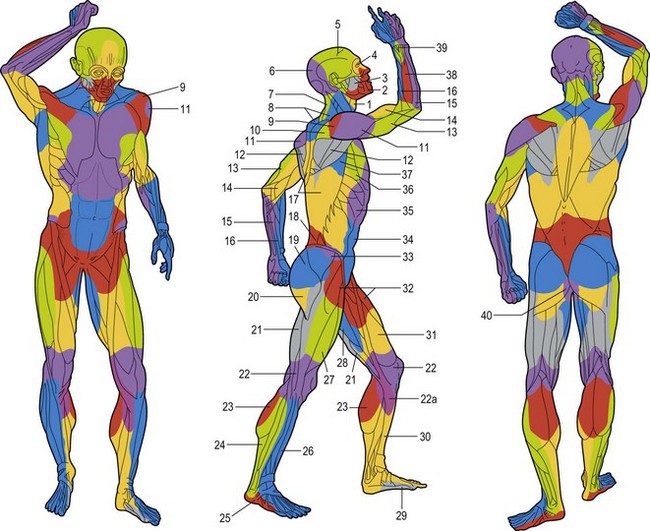
Fig. 23.23 The angiosomes of the source arteries of the body shaded to correspond to Figure 23.9. The angiosomes are: (1) thyroid; (2) facial; (3) buccal (internal maxillary); (4) ophthalmic; (5) superficial temporal; (6) occipital; (7) deep cervical; (8) transverse cervical; (9) acromiothoracic; (10) suprascapular; (11) posterior circumflex humeral; (12) circumflex scapular; (13) profunda brachii; (14) brachial; (15) ulnar; (16) radial; (17) posterior intercostals; (18) lumbar; (19) superior gluteal; (20) inferior gluteal; (21) profunda femoris; (22) popliteal; (22a) descending genicular (saphenous); (23) sural; (24) peroneal; (25) lateral plantar; (26) anterior tibial; (27) lateral femoral circumflex; (28) adductor (profunda); (29) medial plantar; (30) posterior tibial; (31) superficial femoral; (32) common femoral; (33) deep circumflex iliac; (34) deep inferior epigastric; (35) internal thoracic; (36) lateral thoracic; (37) thoracodorsal; (38) posterior interosseous; (39) anterior interosseous; and (40) internal pudendal.
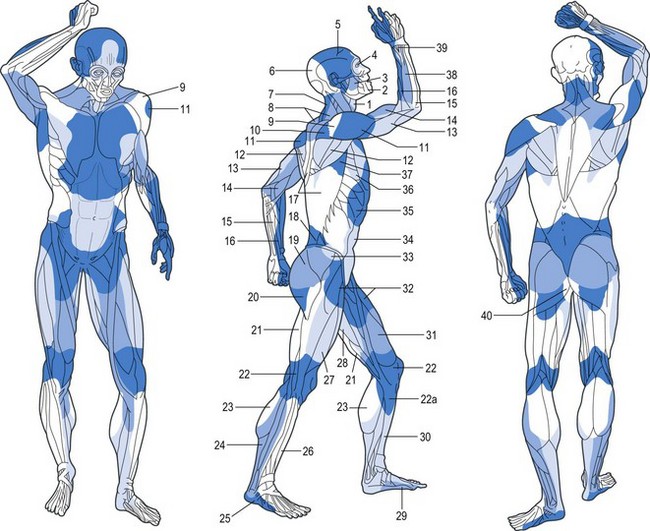
Fig. 23.24 The venosomes of the body. Compare with Figure 23.22.
(From Taylor GI, Caddy CM, Watterson PA, et al. The venous territories (venosomes) of the human body: experimental study and clinical implications. Plast Reconstr Surg. 1990;86:185.)
These composite blocks of skin, bone, muscle, and other soft tissues fit together like the pieces of a jigsaw puzzle, to make up the body. In some of the angiosomes, there is a large overlying cutaneous area and a relatively small deep tissue region; in others, the reverse pattern exists. Each angiosome is linked to its neighbor at every tissue level, either by a true (simple) anastomotic arterial connection without change in caliber of the vessel or by a reduced-caliber choke anastomosis. A similar pattern with avalvular (bidirectional or oscillating) veins on the venous side defines the boundaries of the venosome (Fig. 23.24).
The angiosome concept has several important clinical implications:
1. Each angiosome defines the safe anatomic boundary of tissue in each layer that can be transferred separately or combined on the underlying source vessels as a composite flap. Also, the anatomic territory of each tissue in the adjacent angiosome can usually be captured with safety when it is combined in the flap design.
2. Because the junctional zone between adjacent angiosomes usually occurs within muscles of the deep tissue, rather than between them, these muscles provide an important anastomotic detour (bypass shunt) if the main source artery or vein is obstructed.
3. Because most muscles span two or more angiosomes and are supplied from each territory, one is able to capture the skin island from one angiosome by muscle supplied in the adjacent territory. As we shall see later, this fact provides the basis for the design of many musculocutaneous flaps.
Vascular territories of the body
Vascular territories of the forearm
The forearm is an important flap donor site due to the high incidence of hand and upper extremity injuries around the world.50
Forearm skin
The cutaneous perforators arise directly from the source arteries or from their muscular branches, and then they follow the intermuscular septa distally. Proximally, perforators pierce the muscle bellies near where the muscles are fixed at their origins from bone or from the intermuscular septa. The perforators become more numerous but smaller distally, with the maximum number of small perforators being seen in the palm, where the skin is most rigidly fixed. The cutaneous perforators on both the anterior and posterior surfaces of the forearm emerge in rows along the course of the radial and ulnar arteries (Fig. 23.25).
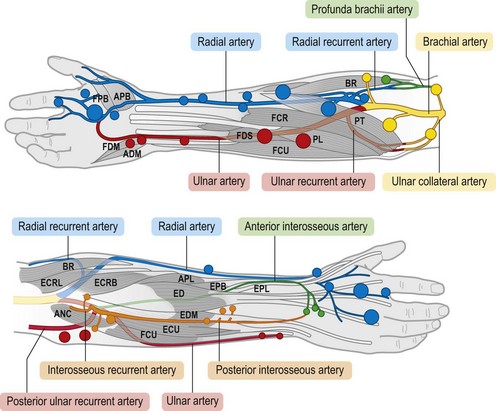
Fig. 23.25 The cutaneous perforators of the forearm, color-coded to match the angiosomes. Large and small skin perforators are indicated by size of the colored markers. Compare with Figure 23.22. FPB, flexor pollicis brevis; APB, abductor pollicis brevis; FDM, flexor digiti minimi; ADM, abductor digiti minimi; FDS, flexor digitorum sublimis; FCR, flexor carpi radialis; FCU, flexor carpi ulnaris; PL, palmaris longus; PT, pronator teres; BR, brachioradialis; ECRL, extensor carpi radialis longus; ANC, anconeus; ECRB, extensor carpi radialis brevis; ECU, extensor carpi ulnaris; EDM, extensor digiti minimi; ED, extensor digitorum; APL, abductor pollicis longus; EPB, extensor pollicis brevis; EPL, extensor pollicis longus.
(From Inoue Y, Taylor GI. The angiosomes of the forearm: anatomic study and clinical applications. Plast Reconstr Surg. 1996;98:195.)
Muscles
The anterior group of forearm muscles can be further subdivided into the superficial and deep muscles. The superficial muscles proximally receive branches from the brachial artery, the ulnar artery, or the ulnar recurrent artery. Distally, they receive branches from the radial artery or ulnar artery (Fig. 23.26).
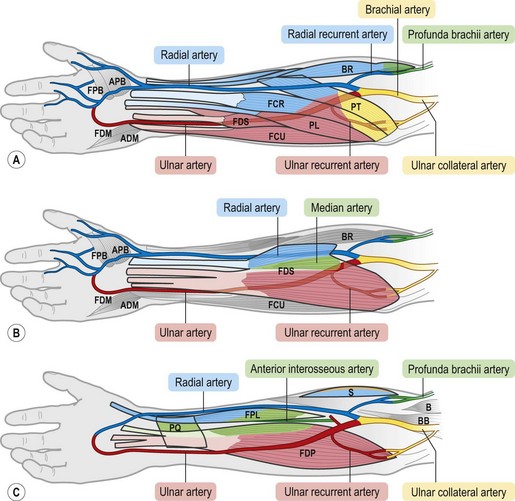
Fig. 23.26 The vascular territories of the (A) superficial, (B) middle, and (C) deep forearm flexor muscles. Note that the junctional zone between angiosomes occurs primarily within the muscles and that most muscles cross at least two angiosomes. Compare with Figures 23.2 and 23.24. FPB, flexor pollicis brevis; APB, abductor pollicis brevis; FDM, flexor digiti minimi; ADM, abductor digiti minimi; FDS, flexor digitorum sublimis; FCR, flexor carpi radialis; FCU, flexor carpi ulnaris; PL, palmaris longus; PT, pronator teres; BR, brachioradialis; PQ, pronator quadratus; FPL, flexor pollicis longus; FDP, flexor digitorum profundus; S, supinator; BB, biceps brachii; B, brachialis.
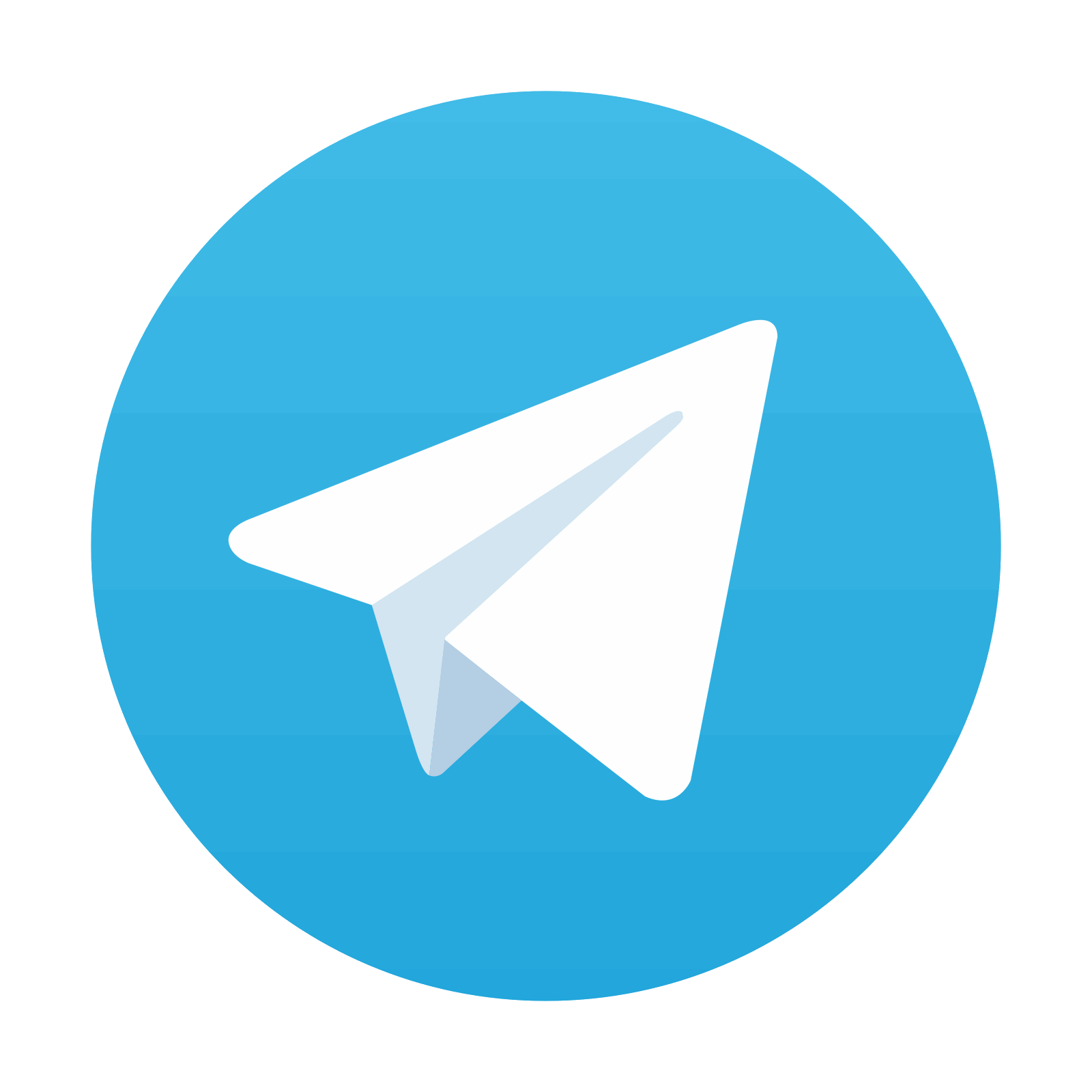
Stay updated, free articles. Join our Telegram channel

Full access? Get Clinical Tree
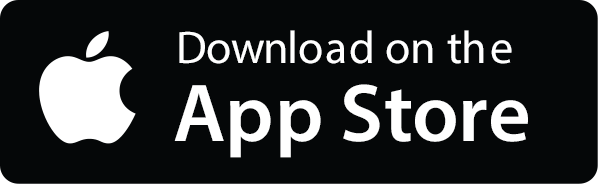
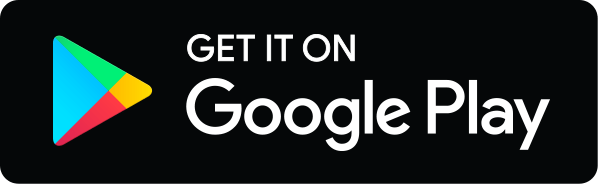
