(1)
Professor of Plastic Surgery, Director of Diabetic Wound Center, Director of Cell Therapy Laboratory, Korea University College of Medicine and Korea University Guro Hospital, Seoul, Republic of Korea (South Korea)
Abstract
For wound coverage, proper selection of the reconstruction method is a key to achieving successful results. A variety of artificial dermal substitutes have been produced and used in clinical practice to accelerate wound healing and reduce wound contraction. However, some authors reported that artificial dermis delays wound healing and procedures using artificial dermis often heal with an unsightly scar. The resultant wound contraction, which is an important clinical component, is not an uncommon problem. Because skin and soft tissue defects are usually created by trauma or surgical excision of tumors, the most common sites are exposed areas such as the face and upper extremities. For this reason, in many cases, cosmetic outcome should also be considered when selecting a treatment method. To minimize the limitation of using artificial dermis, tissue-engineered dermis comprising of cultured fibroblasts seeded on artificial dermis has been developed; some benefits include better graft take, enhanced epithelialization and vascularization, and reduced wound contraction. In this chapter, the author’s experiences with tissue-engineered dermis grafts based on cultured dermal fibroblasts or adipose-derived stromal vascular fraction cells are presented.
Keywords
Tissue-engineered dermisFibroblastSVF cellIn covering wounds, efforts should include utilization of the safest and least invasive methods with a goal of achieving optimal functional and cosmetic outcome. Furthermore, the procedure should be easy to perform and require a short operation time. Proper selection of the reconstruction method is a key to achieving successful results. Because skin and soft tissue defects are usually created by trauma or surgical excision of tumors, the most common sites are exposed areas such as the face and upper extremities. For this reason, in many cases, cosmetic outcome should also be considered when selecting a treatment method.
Recently, the development of advanced technology in the field of wound healing has triggered the use of cells and/or biological dermis to improve wound healing conditions. This technique is simple, is less time consuming, and reduces the surgical burden for patients. The application of artificial dermis and cultured fibroblasts or adipose-derived stromal vascular fraction (SVF) cells is one of the representative treatments.
Two main mechanisms, which mediate wound healing in skin and soft tissue defects, are wound contraction and tissue regeneration including epithelialization and granulation formation. Tissue-engineered dermis grafting cannot only accelerate wound healing but decrease wound contraction, thus ensuring satisfactory aesthetic outcome. Although wound contraction has a positive role in terms of wound healing rate, it can lead to formation of deforming scars and contractures. Therefore, reducing wound contraction without delaying wound healing can improve the overall wound healing outcome. Another advantage of using tissue-engineered dermis is, like other dermis grafts, to provide a graft that is similar to the surrounding skin, thus leaving minimal color mismatch. Because the epidermal portion can be restored by epithelialization, which is induced by migration and proliferation of adjacent epidermal cells including melanocytes, the density and activity of melanocytes and precursor melanocytes in the epidermis of the graft appear similar to those observed in the adjacent skin.
Artificial Dermis vs. Tissue-Engineered Dermis
A variety of artificial dermal substitutes have been produced and used in clinical practice to accelerate wound healing and reduce wound contraction.
When artificial dermis is implanted into full-thickness skin and soft tissue defects, fibroblasts of the wound bed infiltrate the artificial dermis. They accelerate the healing process by regulating matrix deposition, epidermal differentiation, and synthesis of various growth factors and cytokines that stimulate wound healing. However, some authors reported that artificial dermis delays wound healing. After grafting, the formation of new capillaries and deposition of extracellular matrices (ECMs) are observed in the artificial dermis within 2 or 3 weeks. To shorten this period, cultured fibroblasts can be added in the artificial dermis. Fibroblasts are the most important mesenchymal cells involved in wound healing. Many researchers have reported that fibroblasts accelerate the healing process by reducing the time needed for fibroblasts to invade the wound tissue and by early synthesis of new skin. Fibroblasts seeded in the artificial dermis secrete various human ECMs including collagen and glycosaminoglycan, which remain in the artificial dermis, and therefore promote wound healing. Tissue-engineered dermis grafts have been used clinically to treat chronic ulcers and demonstrated promising results. However, unfavorable results have also been reported. Mauch et al. reported that fibroblasts showed increased proteolytic activity once seeded in dermal tissue.
There is controversy in effect of adding fibroblasts on scar contracture as well. Procedures using artificial dermis often heal with an unsightly scar. The resultant wound contraction, which is an important clinical component, is not an uncommon problem. To minimize the limitation of using artificial dermis, tissue-engineered dermis comprising of cultured fibroblasts seeded on artificial dermis has been developed; some benefits include better graft take, enhanced epithelialization and vascularization, and reduced wound contraction.
De vries et al. reported that artificial dermis shows a tendency to reduce wound contraction. The reason can be explained by the role of myofibroblasts which play a major role in wound contraction. Myofibroblasts disappear when epithelialization is well established, meaning that the faster the regeneration of the epidermis is, the greater the suppression of wound contraction. The presence of fibroblasts in artificial dermis leads to increased depositions of basement membrane proteins at the dermoepidermal junction. This promotes dermoepidermal regeneration and keratinocyte attachment, proliferation, and stratification. Another explanation may be that the existing cultured fibroblasts in the artificial dermis are already differentiated and prevent fibroblasts from migrating to the wound site. These migrating fibroblasts have the capability to differentiate into myofibroblasts that cause contraction. Prevention of migration of wound bed fibroblasts with myofibroblast-forming capacity may decrease contraction. Besides the influence of myofibroblasts, rapid dermal regeneration in the fibroblast-contained artificial dermis may also play an important role in reduction of wound contraction. It may be assumed that various ECMs are secreted from the added fibroblasts and locate themselves in the fibers of the artificial dermis. The space between the fibers becomes filled with the newly secreted dermal ECMs which appear to serve as the early reconstruction of a stable matrix and regeneration of a more normal dermal structure. All of the aforementioned factors may support the important role of seeded fibroblasts in improving graft performance and wound healing. However, there have been publications reporting conflicting results. Grinnell et al. and Spector et al. showed that fibroblasts can differentiate into myofibroblasts and lead to the development of contraction.
Likewise, the effects of fibroblast-seeded artificial dermis on wound healing have not been clearly determined. Furthermore, there have been no reports comparing the effects of using artificial dermis, autologous fibroblasts, a combination of the two, and control treatments all together at a time to objectively verify the advantage of using these novel technologies.
Animal Study
The author has performed an animal study to evaluate the effects of autologous fibroblast-seeded artificial dermis on wound healing rate and wound contraction, which are the two main factors of wound healing efficacy, by comparing those of artificial dermis and fibroblasts.
Methods
The artificial dermis used in the study was a commercial dermal substitute consisting of a native (non-cross-linked) bovine type I collagen matrix supplemented by an elastin hydrolysate (MatriDerm®; Skin & Health Care AG, Billerbeck, Germany). The artificial dermis was cut using a 6-mm punch into 6-mm-diameter disks. One hundred microliters of Dulbecco’s phosphate-buffered saline without Mg2+ and Ca2+ (DPBS) solution containing 1 × 105 cultured human fibroblasts was applied to each artificial dermis disk using a micropipet.
Full-thickness 6-mm punch wounds were created through the skin and the panniculus carnosus on the dorsal surface (two wounds each on the left and right sides) of each genetically created 5-week-old nude mouse.
Four types of grafts were applied on the four wounds of each mouse; 100 μl of DPBS solution without cells (control group), 100 μl of DPBS solution containing 1 × 105 cultured fibroblasts (FB group), an artificial dermis disk containing 100 μl of DPBS solution without cells (AD group), and the previously mentioned fibroblast-seeded artificial dermis disk (AD with FB group).
On the 14th day after treatment, wound healing rate and wound contraction were evaluated using a stereoimage optical topometer (SOT) system, histomorphological analysis, and immunohistochemistry (Figs. 4.1, 4.2, and 4.3).




Fig. 4.1
(A) The artificial dermis composed of the collagen and elastin complex (MatriDerm®) used for the experiment. (B) Magnification (×100) of the dry MatriDerm®. (C) The MatriDerm® soaked with DPBS. (D) Magnification (×100) of the wet MatriDerm®. (E) Magnification (×400) of the MatriDerm® seeded with the human fibroblasts (arrows)

Fig. 4.2
Photographs obtained by an ordinary digital camera. (A) View of the four dorsal full-thickness wounds created by a 6-mm punch. (B) Immediate after grafting. In this mouse, DPBS (control group), fibroblasts suspended in DPBS (FB group), artificial dermis (AD group), and fibroblast-seeded artificial dermis (AD with FB group) were applied on the left upper, right upper, left lower, and right lower side wounds, respectively. (C) Fourteen days after the treatments

Fig. 4.3
Photographs of a wound obtained by the SOT system. (A) A full-thickness round wound before treatment. (B) The wound 14 days after treatment. (C) ① Initial wound area. ② Total wound area. ③ Unhealed wound area. (D) ⓐ Contracted area (① – ②). ⓑ Epithelialized area (② – ③)
Results
Photometric Study for Wound Healing Rate
The AD group showed the largest nonhealed wound area, followed by the AD with FB group, the control group, and the FB group. There was a statistically significant difference between the AD group and the control group. However, the AD with FB group and the FB group did not demonstrate statistically significant differences with the control group.
These results imply that the artificial dermis treatment delayed wound healing, but the fibroblast and the fibroblast-seeded artificial dermis treatments did not have significant influence on wound healing rate (Fig. 4.4).


Fig. 4.4
Photographs of healed wounds of a mouse obtained by the SOT system. (A) The control group. (B) The FB group. (C) The AD group. (D) The AD with FB group. (E–H) Measurement of the total and nonhealed wound areas. AD with FB treatment showed the most excellent result considering the wound healing rate and the degree of wound contraction
Photometric Study for Wound Contraction
The control group showed the largest contracted area, followed by the FB group, the AD group, and the AD with FB group. The difference between the control group and the FB group was not significant. However, the AD group and the AD with FB group showed statistically significant differences with the control group.
These results imply that the artificial dermis and the fibroblast-seeded artificial dermis treatments helped to reduce wound contraction, but the fibroblast treatment did not (Fig. 4.4).
Histological Analysis
H&E and Masson’s trichrome staining demonstrated that collagen fibers in the control group were irregularly arranged, but collagen structures in the FB group, the AD group, and the AD with FB group showed a relatively regular pattern. A noteworthy finding was that regenerated dermoepidermal junction in the AD with FB group exhibited a more prominent rete ridgelike structure compared with the other three groups (Fig. 4.5).


Fig. 4.5
Histology (Masson’s trichrome stain) of healed wounds of a mouse (×100). (A) The control group. (B) The FB group. (C) The AD group. (D) The AD with FB group. Collagen fibers in the control group were irregularly arranged, but collagen structures in the FB, the AD, and the AD with FB groups showed a relatively regular pattern. AD with FB group demonstrated a more normal skin appearance having a more prominent rete ridgelike structure compared with the other three groups
This finding may imply that more normal skin structure was regenerated by treatment with fibroblast-seeded artificial dermis.
Immunohistochemistry of α-SMA
The control group exhibited the greatest amount of the α-SMA, followed by the FB group, the AD group, and the AD with FB group. The difference between the control and the FB groups did not reach statistical significance. However, the AD group and the AD with FB group showed statistically significant differences with the control group (Fig. 4.6).


Fig. 4.6
Immunochemistry of the α-SMA (×400). White areas exhibit the presence of the α-SMA. (A) The control group. (B) The FB group. (C) The AD group. (D) The AD with FB group. The AD with FB group demonstrated the least amount of the α-SMA
These results show that the findings of the α-SMA were consistent with those of the wound contraction study using the SOT system.
Conclusions
The results of the animal study showed that treating wounds by using only artificial dermis may be beneficial in reducing wound contraction, but may delay wound healing. Fibroblast-seeded artificial dermis graft may minimize wound contraction without significantly delaying wound healing for the treatment of skin and soft tissue defects.
Fibroblast-Seeded Artificial Dermis
The use of cultured fibroblasts is representative of the tissue engineering of soft tissue, and numerous previous studies have shown that fibroblasts are suitable for the treatment of soft tissue defects. The author has used tissue-engineered dermis graft comprising of autologous cultured dermal fibroblasts seeded on a nonwoven hyaluronic acid sheet after removal of basal cell carcinomas (BCCs) on the face. BCC is the most common human malignancy. BCC is usually slow-growing tumor arising on sun-exposed areas of the body, with the face being the most common site. Different treatment modalities have been used to treat BCCs. However, surgery is the most frequently used treatment for BCCs because it can completely define and confirm tumor boundaries by histopathologic margin evaluation. Surgical excision of a BCC leaves a skin and soft tissue defect which requires reconstruction.
Application Methods
A skin biopsy (1-2 cm2) is taken from a patient. Autologous dermal fibroblasts are isolated and cultured. Once the fibroblast number reaches 5 × 106, the cells are then seeded on a distinct biodegradable scaffold composed entirely of a benzylic ester of hyaluronic acid, which is a three-dimensional nonwoven scaffold. Starting on the eighth day from seeding, the fibroblast-hyaluronic acid complex is ready for transplantation (Fig. 4.7). Approximately 4 weeks are required after the skin biopsy to prepare the tissue-engineered dermis.


Fig. 4.7
Preparation of the tissue-engineered dermis. (A) Skin harvest. (B) Microscopic view of cultured dermal fibroblasts. (C) Fibroblast pellet and nonwoven hyaluronic acid sheet. (D) A hyaluronic acid sheet seeded with cultured fibroblasts. (E) Magnification (×100) of the tissue-engineered dermis. (F) Magnification (×400) of the tissue-engineered dermis. Arrows indicate dermal fibroblasts attached to hyaluronic acid fibers
After local anesthesia with an epinephrine-containing lidocaine solution, a BCC is excised at an angle of 45° in the subcutaneous tissue, including a 5-mm clinically tumor-free resection margin, to obtain a bowl-shaped excision specimen. Intraoperative frozen section analysis is used to assure negative surgical margins. If residual tumor is noted, the procedure is repeated until the area was tumor-free.
The tissue-engineered dermis is tailored to the size and shape of the defect and is transferred to the recipient site in two to three layers. The grafts are fixed to the defect using fibrin glue and covered with a polyurethane foam dressing. Wounds are kept moist until complete reepithelialization is achieved. Sunshine is avoided for the first 3–6 months using sunblocking agents (Figs. 4.8 and 4.9).



Fig. 4.8
Application technique. (A) A skin defect. (B) Tailing of the tissue-engineered dermis. (C) Immediate after the application of the tissue-engineered dermis. (D) Sealing with fibrin glue to maintain moisture. (E) Polymerization of the glue. (F) A secondary dressing with a polyurethane foam

Fig. 4.9
A basal cell carcinoma treated by the tissue-engineered dermis. (A) Preoperative view. (B) After wide excision of the tumor. (C) Immediate after the graft of the tissue-engineered dermis. (D) Four weeks after the graft. (E) Three months after the graft. (F) One year after the graft
Clinical Study
The author has performed a clinical study to report the efficacy of the cultured autologous fibroblast-seeded artificial dermis in the treatment of skin and soft tissue defects created by removal of BCCs on the face. This study used existing data by reviewing the medical records of 29 patients who were treated for facial defects created by resections of BCCs using artificial dermis (Hyalomatrix®; Chabio & Diostech, Seoul, Korea) or fibroblast-seeded artificial dermis. A long-term follow-up of more than 1 year was possible in all patients.
Sixteen of the twenty-nine patients were treated with the graft of tissue-engineered dermis. The mean patient age was 70.6 ± 8.3 years, and the defect sizes ranged between 2.0 and 9.1 cm2 (mean area, 4.6 ± 1.9 cm2). Recipient sites were located as follows: seven on the orbital area, five on the nasal area, and four on the cheek. Control treatment (graft of artificial dermis without cells) was performed on 13 of the 29 patients. The mean age of the control group was 73.2 ± 7.7 years, and wound sizes ranged from 2.0 to 8.4 cm2 (mean, 4.2 ± 1.6 cm2). Recipient sites were located as follows: five on the orbital area, six on the nasal area, and two on the cheek.
All tissue-engineered and artificial dermis grafts were well taken to the wound beds without graft rejection or graft failure in both groups. Reepithelialization occurred progressively from the periphery to the center of the newly formed granulation tissue. The whole wound of the tissue-engineered dermis graft reepithelialized after grafting within 21–42 days (average 31.4 ± 5.3 days). Artificial dermis-grafted wounds were healed by 28–42 days (average 34.2 ± 4.9 days). However, no statistically significant differences were observed in healing time. Most patients treated with the tissue-engineered dermis had satisfactory results with high-quality skin characteristics. With respect to scar height, nine (56 %) scars were flat, three (19 %) were slightly depressed, and four (25 %) were slightly elevated. No hypertrophic or keloid scars were formed. A favorable smooth texture was achieved in ten (63 %) cases and an irregular texture was achieved in six (37 %) cases. The majority of the scars (13/16, 81 %) had favorable color matches to the surrounding skin; two (13 %) and one (6 %) scars were hypopigmented and erythematous, respectively. No hyperpigmentation was observed. Even though slight scar contractures were observed in three (19 %) patients, they did not show any functional or aesthetic problems. Comparing the results of the scars of the tissue-engineered dermis group to those of the artificial dermis group, there were significant differences in terms of height and contracture of scars. However, no statistically significant differences were detected in scar texture and color match. Patient satisfaction in the tissue-engineered dermis graft group was also better than that in the artificial dermis group.
No revision procedures were needed to improve scar quality in the tissue-engineered group, but two patients in the artificial dermis group underwent skin grafting due to severe scar contractures. Except for these cases, there were no significant complications and no tumor recurrences during the follow-up period in either group (Figs. 4.10, 4.11, and 4.12).



Fig. 4.10
Treatment of BCCs on the lower eyelids. The upper case was treated with an artificial dermis graft. (A) After wide excision of the tumor. (B) Immediate after grafting of the artificial dermis. (C) One-year postoperative view. This case showed a conspicuous scar with contracture. The lower case was treated with a tissue-engineered dermis graft. (D) After wide excision of the tumor. (E) Immediate after grafting of the tissue engineered dermis. (F) One-year postoperative view. The scar condition was satisfactory compared with the upper case

Fig. 4.11
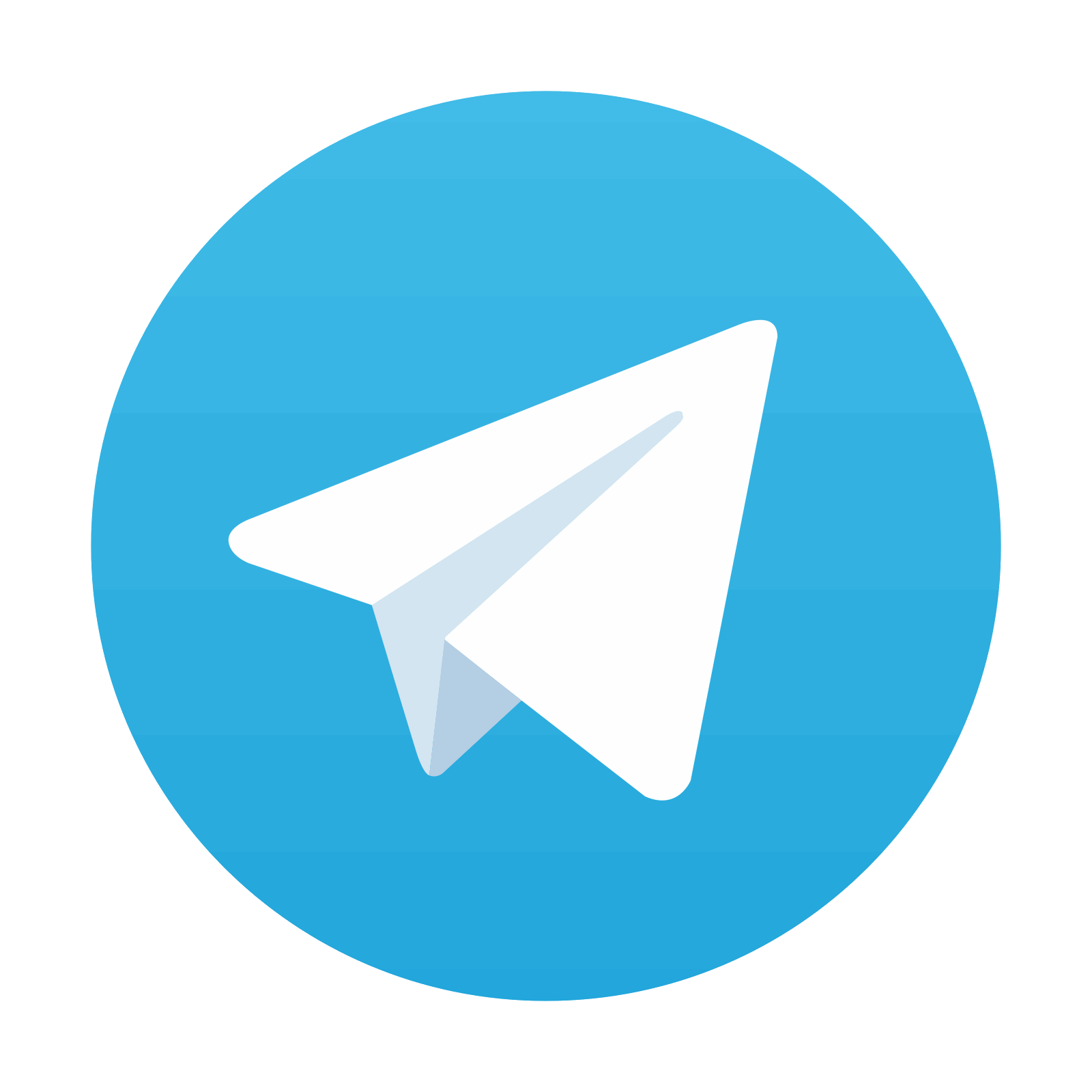
Reconstruction of defects on the alar grooves. The upper case was treated with an artificial dermis graft. (A) Preoperative view. (B) After wide excision of the tumor. (C) Immediate after grafting of the artificial dermis. (D) Two years after the graft. This artificial dermis graft resulted in an unsightly scar. The lower case was treated with a tissue-engineered dermis graft. (E) Preoperative view. (F) After wide excision of the tumor. (G) Immediate after grafting of the tissue-engineered dermis. (H) One-year postoperative view. The scar condition was acceptable compared with the upper case
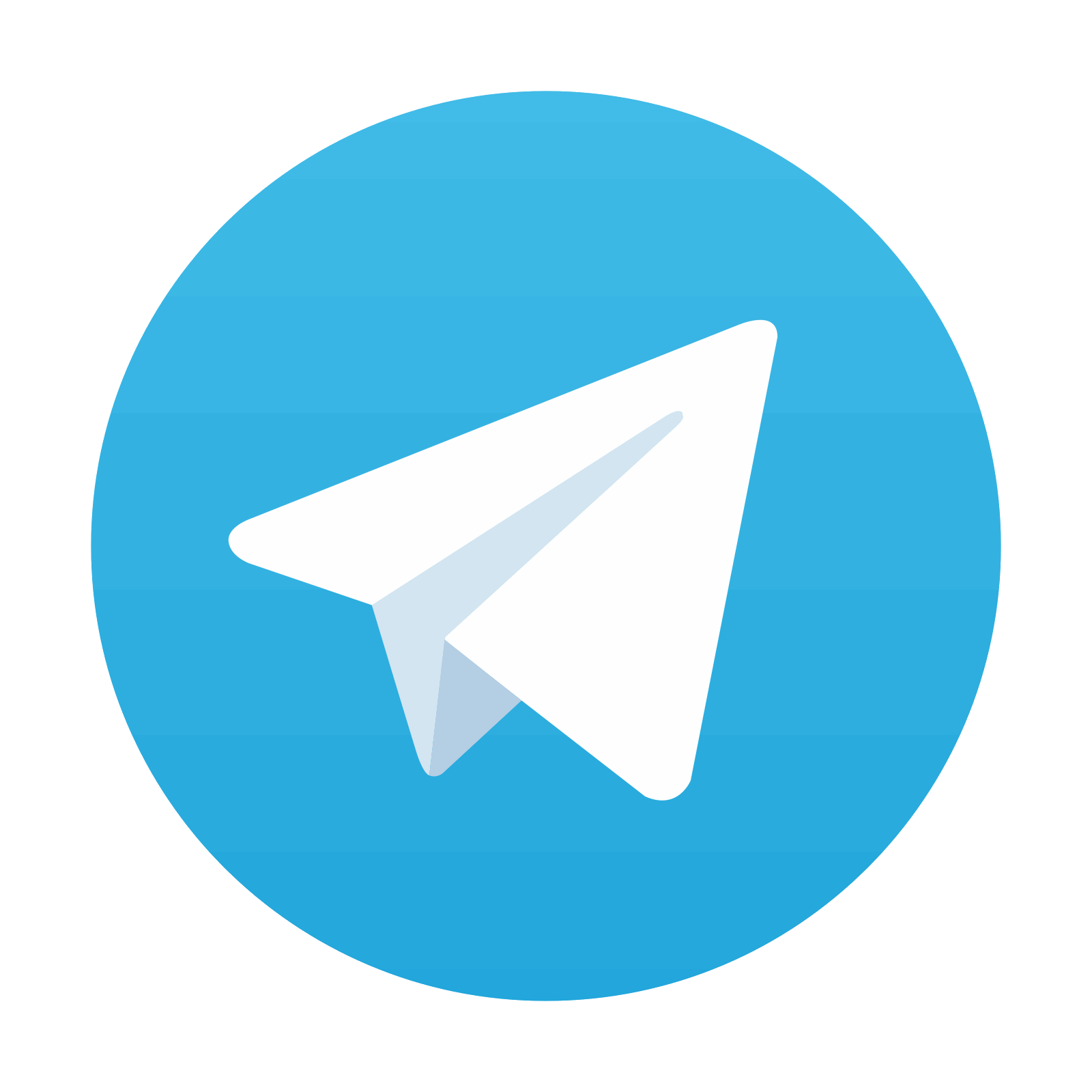
Stay updated, free articles. Join our Telegram channel

Full access? Get Clinical Tree
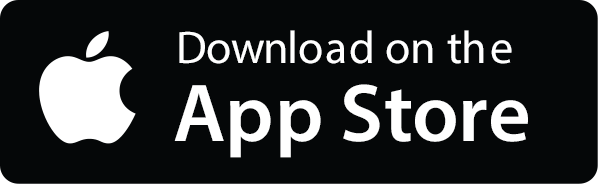
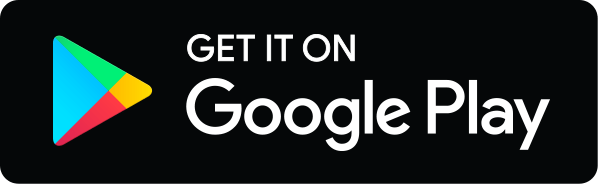
