Criteria
Reduces anesthetic requirements for procedure
Reduces operating time for procedure
Reduces complications or morbidity for procedure
Reduces recovery time for procedure
Facilitates new or improved technical approaches lacking in conventional or existing techniques
More than one novel application is possible with new technology
8.2 History of Subcutaneous Fiber Laser Techniques for Facial and Neck Rejuvenation
The history of laser lipolysis and energy-based soft tissue coagulation/contraction is a relatively brief one and has been summarized well by DiBernardo et al1 who note Apfelberg2 is credited for describing the laser–fat interaction in 1992. Publications by Blugerman, Schavelzon, and Goldman3 followed where each demonstrated personal experience with lasers on adipose tissue. Badin et al4 also highlighted the important tissue retraction observed with a technique of laser lipolysis. Ichikawa et al5 published on the histological evaluation of tissue treated with laser lipolysis, showing the destructive changes of heat-coagulated collagen fibers and degenerated fat cell membranes with dispersion of lipids after laser irradiation of human specimens. These histological changes correlate with clinical changes seen by both physician and patient. Further, the hemostatic properties of the 1,064-nm wavelength have been well documented. The thermal effect produced by the neodymium:yttrium aluminum garnet (Nd:YAG) laser (1,064 nm) in the adipose tissue promotes better hemostasis resulting in less surgical trauma and wound healing with fewer adverse sequelae. In addition to the histological evidence, the clinical evaluation shows improved postoperative recovery, resulting in a more rapid return to daily activities with an excellent aesthetic result. The application of laser lipolysis to facial and neck rejuvenation in conjunction with the advanced facial rejuvenation techniques of SmartLifting were first studied by Gentile in 2007 and reported in 2008,6 2009,7 2010,8 and 2011.9,10 The initial procedures were performed with the SmartLipo (▶ Fig. 8.1) (Cynosure, Waltham, Massachusetts) 1,064-nm laser but on introduction of the SmartLipo MPX (Cynosure) (▶ Fig. 8.2) the 1,064/1,320-nm multiplexed laser was used. The SmartLipo Triplex (▶ Fig. 8.3) and, beginning in 2012, the Precision TX handpiece (▶ Fig. 8.4)―operating at 1,440 nm and delivered through an 800-µm fiber―have been utilized. The Cynosure SmartLipo laser was the first laser to be approved by the Food and Drug Administration (FDA) for laser lipolysis. In addition to the laser lipolysis indication the laser is approved for the surgical incision, excision, vaporization, ablation, and coagulation of soft tissue. All soft tissue is included―skin, cutaneous tissue, subcutaneous tissue, striated and smooth tissue, muscle, cartilage meniscus, mucous membrane, lymph vessels and nodes, organs, and glands. Since the 2006 advent of subcutaneous laser-based lipolysis techniques, many other laser companies have developed similar products and many have introduced different wavelengths for the specific indication of laser lipolysis. Other wavelengths for laser lipolysis include 980 and 1,444 nm. Since the introduction of these fiber laser techniques in 2007 many other authors including DiBernardo,11 Sasaki,12 Holcomb et al,13 Goldman et al,14 and Sarnoff15 have described their experiences with subcutaneous fiber lasers in facial and neck rejuvenation procedures.
Fig. 8.1 Small Footprint SmartLipo 1,064-nm neodymium:yttrium aluminum garnet (Nd:YAG) laser. The SmartLipo laser was initially approved at 6 watts (W) and underwent power upgrades to 18 W prior to advancing to the MPX and now Triplex versions. (Reproduced with permission from Gentile RD, ed. Neck Rejuvenation. New York, NY: Thieme Medical Publishers; 2011:147.)
Fig. 8.2 SmartLipo MPX. The MPX or MultiPlex permits laser use in either the 1,064 or 1,320-nm mode or MultiPlex, which is sequential emission of 1,064 and 1,320 nm in three blends. (Reproduced with permission from Gentile RD, ed. Neck Rejuvenation. New York, NY: Thieme Medical Publishers; 2011:149.)
Fig. 8.3 Introduced in 2011, the advancement of the SmartLipo Triplex allows the use of three laser wavelengths (1,064, 1,320, and 1,440 nm) to be utilized independently or uniquely blending the MultiPlex technology. The 1,440-nm wavelength alone has a 40 × greater absorption rate in fatty tissue than that of other diode lasers (924 and 980 nm) available. The Triplex also offers the Cellulaze platform for correction of cellulite. (Courtesy of Cynosure, Westford, Massachusetts.)
Fig. 8.4 The Precision TX fiber (SideLaze 800 3D) uses the same laser wavelength as Cellulaze but the fiber is smaller and is able to deliver less energy more precisely. The laser beam is split, just as is in Cellulaze, so that the energy can be directed toward the undersurface of the skin to achieve enhanced skin tightening. The Precision TX device was designed to treat small areas in the face such as the neck and jowls.
Courtesy of Cynosure, Westford, Massachusetts.
8.3 The Technology of Subcutaneous Fiber Laser Techniques for Facial and Neck Rejuvenation
The original SmartLipo laser (▶ Fig. 8.1) delivered 1,064 nm of optical energy through a 300-µm fiber at 6 W (▶ Fig. 8.5). Subsequently, next-generation Cynosure SmartLipo and SmartLipo MPX lasers utilized a 600-µm fiber (▶ Fig. 8.6) as well as a 1,000-µm fiber (▶ Fig. 8.7) for high-power laser lipolysis. The unit developed for dedicated facial and neck contouring known as the Precision TX handpiece utilizes an 800-µm fiber. First-generation lasers operating at 6, 10, 12, and 18 W utilized a 600-µm optical fiber introduced through a 1-mm diameter stainless steel microcannula of variable length for facial laser-assisted procedures. The laser is fired through the distal end of the fiber that protrudes 2 mm beyond the tip of the cannula. The distal end of the fiber interacts with the facial and neck soft tissue. For visualization purposes, an aiming laser source is provided in the beam path providing the precise location of the fiber tip, indicating where the laser is working. For most facial and neck anatomical regions, a 6- to 12-W setting, 100-µs pulsed laser at 40 Hz and 150 mJ was used. The SmartLipo MPX laser, which is capable of blending both the 1,064- and 1,320-nm wavelengths, was used in subsequent years and in 2012 was replaced by the 1,440-nm laser available in the SmartLipo Triplex and is also used in the Cellulaze (Cynosure) system. The SmartLipo systems all utilize an Nd:YAG laser tuned to the desired wavelength that produces photomechanical and thermal effects. This facilitates dissection of tissues quickly and easily. In addition, the Nd:YAG laser’s hemostatic properties allowed for the coagulation of small blood vessels in the subcutaneous plane with preservation of the dermal plexus of vessels. Multiplexing the 1,064- and 1,320-nm wavelengths provides some unique advantages. SmartLipo with multiplex (SmartLipo MPX) (▶ Fig. 8.2) allows individual as well as sequential emission of 1,064- and 1,320-nm wavelengths. The sequential firing of these two wavelengths in combination maximizes the positive properties of both. The combination of these wavelengths increases the efficiency of fat lipolysis and offers a more evenly distributed laser energy profile that benefits superficial and deep treatment. These two wavelengths emitted sequentially offer a more efficient vascular coagulation through the conversion of hemoglobin to methemoglobin.8 The 1,320-nm wavelength heats the blood, converting hemoglobin to methemoglobin. The 1,064-nm wavelength has a 3 to 5 times greater affinity for methemoglobin than for hemoglobin, thereby increasing absorption resulting in more efficient coagulation leading to enhanced skin tightening. SmartLifting permitted flap separation in typically difficult to reach areas such as the nasal labial folds (NLF) and the corner of the mouth and infracommissural folds, also known as marionette lines, when completing “full rhytidectomy.” The wavelength characteristics and thermodynamic photo spectrum of the 1,064-nm laser are shown in ▶ Fig. 8.8; and the properties of the blended 1,064/1,320-nm multiplexed laser are displayed in ▶ Fig. 8.9. With the advent of the SmartLipo MPX laser, several innovations were introduced to enhance patient safety in reducing the risk of thermal injury. One feature is the SmartSense motion control sensor that will stop laser emission when the laser is not being moved. The second device is the ThermaGuide, a deep temperature sensor that gives the operator instant feedback on the temperature of the laser internally. The final technology innovation by Cynosure in the SmartLipo platform and a by-product of the Cellulaze research is the Precision TX (▶ Fig. 8.4 and ▶ Fig. 8.10) fiber utilized with the 1,440-nm laser also known as SideLaze 3D. Due to its unique structure and design it is able to fire in specific directions. This differs from the unidirectional firing of the earlier SmartLipo fibers. The operator changes hand position in order to change laser firing position from down to sideways to up. The laser also fires ahead as well as the other selected direction. A chevron marks the spot of directional firing for the Precision TX (▶ Fig. 8.11). The incorporation of the 1,440-nm wavelength offers increased benefits for laser lipolysis and tissue lifting for facial rejuvenation. The 1,440-nm wavelength achieves high thermal absorption within fat and collagen (water), leading to fat reduction and collagen denaturation, and progressive tissue lifting. The longer wavelength provides increased localized photothermal and vaporizing effects in front of the fiber on fatty tissue and collagen fibers (water), achieving 20 times more absorption in adipose tissue than the 1,064/1,320-nm (▶ Fig. 8.12) and 40 times more absorption than the 924/980-nm wavelengths. The combination of the 1,440-nm wavelength, side-firing fiber, and the thermal-control systems provided a safe and effective means for facial rejuvenation.
Fig. 8.5 The original 300-µm fiber used for lipolysis and tissue coagulation. (Reproduced with permission from Gentile RD, ed. Neck Rejuvenation. New York, NY: Thieme Medical Publishers; 2011:148.)
Fig. 8.6 The 600-µm fiber. (Reproduced with permission from Gentile RD, ed. Neck Rejuvenation. New York, NY: Thieme Medical Publishers; 2011:148.)
Fig. 8.7 The 1,000-µm fiber. (Reproduced with permission from Gentile RD, ed. Neck Rejuvenation. New York, NY: Thieme Medical Publishers; 2011:148.)
Fig. 8.8 Wavelength characteristics in laser-assisted facial rejuvenation. Characteristics and photothermal footprint of 1,064-nm wavelength. (Reproduced with permission from Gentile RD. Neck Rejuvenation. New York, NY: Thieme Medical Publishers; 2011:150.)
Fig. 8.9 Wavelength characteristics in laser-assisted facial rejuvenation. Characteristics and photothermal footprint of blended 1,064- and 1,320-nm wavelengths. (Reproduced with permission from Gentile RD. Neck Rejuvenation. New York, NY: Thieme Medical Publishers; 2011:152.)
Fig. 8.10 The SideLaze 3D fiber permits the surgeon to aim the beam down, sideways, or up, which facilitates lipolysis, fibroseptal network (FSN) tightening, and dermal skin tightening.
Fig. 8.11 The Precision TX handpiece and SideLaze 3D fiber are aimed by having the chevron on the handpiece positioned down, sideways, or up.
Fig. 8.12 The water and fat absorption graph shows peak absorption for fat at 1,210, 1,740, and 2,300 nm. The 1,440-nm wavelength alone has a 40 × greater absorption rate in fatty tissue than that of other diode lasers (924 and 980 nm) available.
8.4 Classification of Energy-Based Fiber Laser Lifts for Facial and Neck Rejuvenation Techniques
As the technology powering the lipolysis lasers advanced over the past decade, the types of procedures being done in conjunction with interstitial laser lipolysis did so as well. As with other types of techniques, not every surgeon performs each procedure in quite the same way. For example, in performing “laser facelifting” or fiber laser lifting (FLL): is the surgeon only laser treating the face and neck subcutaneously? Or is the surgeon laser treating the face and neck, aspirating and placing sutures, or removing skin in conjunction with the interstitial laser lipolysis? As the number of reports of these procedures grew, the author wanted to develop a classification system of energy-based facial and neck rejuvenation procedures that would permit students and colleagues to understand exactly what was being done in conjunction with the interstitial laser lipolysis. It was decided to broaden the categorization to include as well energy-based neck treatments that do not puncture the skin and are delivered transcutaneously.
8.4.1 Type I: Superficial Noninvasive Transcutaneous Laser or Energy-Based Face/Neck/Skin Rejuvenation
There has been a very rapid progression of technologies that have facilitated our ability to provide moderate, minimally invasive, nonexcisional skin tightening with transcutaneous laser and energy-based devices. Many of these procedures have become important and sought after by our patient populations and are a centerpiece of consumer interest. Because patients desire effective procedures with decreased downtime, it behooves aesthetic physicians and surgeons to seek a nonsurgical approach to neck rejuvenation that centers on skin tightening. There has been an evolution of devices starting with near-infrared lasers operating in the 1,320- to 1,440-nm wavelengths, long-pulsed Nd:YAG lasers, and multiplexed lasers operating in the same infrared spectrum. These lasers were then replaced by various RF externally applied instruments that acted as efficient “bulk heaters” of the dermis. Monopolar RF and combined optical–bipolar RF mechanisms emerged and used procedures with multiple-pass, multiple-session protocols. These tools are static in nature and provide inadequate dermal stimulation, exposing dermal tissues to relatively low thermal stimulation due to short pulse duration. More recent developments in technology have produced devices that are not short pulse duration in design. These are continuous wavelength (CW) RF systems that are constantly moved along the surface of the skin. These dynamic systems have the ability to heat tissues to therapeutic temperatures relatively quickly, and to maintain these therapeutic temperatures for longer periods of time without significant patient discomfort or thermal-related skin complications. These transcutaneous, deep, dermal ablative devices include high frequency ultrasound (US) that acts by creating US-induced fractional thermal ablative zones in the deep dermis and in some cases the superficial aponeurotic system. These devices and their trade names are reviewed by Mulholland.16
8.4.2 Type II: Subcutaneous Laser or Energy-Based Interstitial Lipolysis/Liposculpture and the Fibroseptal Network
Type II procedures are focused on the subcutaneous and interstitial application of energy (laser or RF) via small portals on the face and neck. As this chapter goes to press several new devices have been introduced that utilize RF microneedling techniques such as the Syneron Profound and the Lutronic Infini. These devices will be classified as Type II A (▶ Fig. 8.42) as they perforate the skin aiming energy at the reticular dermis (the microincision laser or catheter-based devices are classified as Type II B). The interaction of the laser with the tissue is achieved by the absorption of the laser energy by the receptive chromophores, thus producing sufficient heat to cause the desired thermal damage. The heat acts on the fatty cell and the extracellular matrix including the fibrous septa (fibroseptal network [FSN]) to produce both reversible and irreversible cellular damage. Several studies have demonstrated that for low energy settings at different wavelengths a tumefaction of the adipocytes is observed leading to an increase of their diameter up to 100 µm.4,5 The heat generated by the laser would alter the balance of sodium and potassium of the cellular membrane, allowing the free transport of extracellular liquid to the intracellular atmosphere. For higher energy settings, rupture of adipocytes and coagulation of collagen fiber and small vessels are observed.3,5 Due to the rupture of the membrane, lipases liberated by the adipocyte are responsible for the liquefaction of the tissue, which further facilitates the subsequent aspiration. Through the liquefactive effect of the laser, the back and forth movement of the cannula is performed much more easily than when performing the conventional liposuction technique. The fact that heat also induces coagulation of small vessels in the fat tissue is very important; this phenomenon facilitates the liposuction through lesser trauma and bleeding. Liposculpture removes significant amounts of fat, serum, and blood. In cases where large amounts of fatty tissue are to be removed, a physiologically significant loss of blood can provoke metabolic alterations. In this way, laser-assisted liposculpture offers the advantage of removing larger volumes of fat without hemodynamic repercussions.4 As with type I procedures, the thermic and athermic events induce deep reticular dermal collagen denaturization and a cascade to neocollagenesis of the deep reticular layer and remodeling of the FSN (▶ Fig. 8.13, ▶ Fig. 8.14, ▶ Fig. 8.15), which serve to help firm, tighten, tone, or lift the skin. In FLL type II the procedure description should include referencing whether or not aspiration was performed.
Fig. 8.13 Effects of subcutaneous laser and energy-based devices in dermis and hypodermis. (a) Short-term soft tissue effects in dermis and hypodermis are depicted: (1) nonablative papillary changes, (2) reticular dermal coagulation, (3) adipose coagulation, vascular coagulation, and (4) contracted fibroseptal network (FSN) bands. (b) Also shown are long-term reorientation of intermediate hypodermis and FSN, and (1) dermal neocollagenesis with remodeling; dermal remodeling 30% contraction; and (2) remodeled FSN bands.
Fig. 8.14 The LaserFacialSculpting (LFS) treatment grids utilized for delineating the procedure areas in facial zones in midface, lower face, and lateral neck. (Reproduced with permission from Gentile RD, ed. Neck Rejuvenation. New York, NY: Thieme Medical Publishers; 2011:159.)
Fig. 8.15 The LaserFacialSculpting (LFS) central and lateral treatment zones for the middle neck. (Reproduced with permission from Gentile RD, ed. Neck Rejuvenation. New York, NY: Thieme Medical Publishers; 2011:159.)
8.4.3 Type III: Concurrent Nonexcisional Surgical Neck Techniques (Submentoplasty or SMAS Procedures)
In the remainder of the energy-based facial and neck rejuvenation techniques, all procedures are preceded by a subcutaneous interstitial laser lipolysis and then a subsequent rhytidectomy procedure of the neck (FLL type III), face (FLL type IV), or both face and neck (FLL type V) involving concurrent rhytidectomy techniques. In type III procedures, there are isolated nonexcisional platysmal or superficial musculoaponeurotic system (SMAS) procedures that may include surgical correction of platysmal banding that can be diminished by plication or division of the platysma muscle. Limited SMAS elevation can also be placed in this minimal incision non-excisional category. After identifying the medial platysmal edges, approximation is performed. An absorbable or nonabsorbable suture is used to create a muscle sling. The plication should extend from the submental incision to the thyroid cartilage. If a running suture is used, the plication should continue in a reverse direction, allowing for the plication of the lateral edges. A wedge excision of the medial border of the platysma at the cervicomental angle may also provide additional definition. Another technique for platysmaplasty is purse-string platysmaplasty.17 At times in the performance of a laser neck lift the author will also place lateral sutures or a running suture along the inferior border of the mandible in order to define the mandible. In type III procedures, any nonexcisional neck rejuvenation procedure performed and preferred by the surgeon in completing an isolated submentoplasty or isolated neck lift can be performed, and it differs only by the antecedent laser irradiation/elevation of the neck flaps with or without concurrent laser lipolysis and liposculpture.
8.4.4 Type IV: Concurrent Facial Rhytidectomy Techniques (Mini-Lifts)
In FLL type IV procedures a platysmaplasty or submentoplasty is not performed but a facial rhytidectomy is performed. This rhytidectomy may include a lateral plication of the superficial musculoaponeurotic system (SMAS), but does not utilize midline surgical techniques other than laser lipolysis with or without liposculpture. In the event subplatysmal fat is treated, it would be classified as a type IV procedure provided medial suture techniques are not used. As with the FLL type III procedures, any type of rhytidectomy procedure may be performed as long as midline platysmaplasty techniques are not used. Most commonly in the author’s practice a mixed plane rhytidectomy18 is performed via an intermediate length incision and a short or intermediate flap elevation. In adding rhytidectomy techniques it is useful to know what exactly is done during the rhytidectomy, and there exists considerable confusion as to what different nomenclature means. Is the lift called a mini-lift short scar, short flap, or both? In order to be able to compare facelift results based on what actually was done, the author has proposed a classification of cervicofacial rhytidectomy that simplifies the nomenclature. Up until now the definition and classification of mini-lifts and other more extensive forms of rhytidectomy have been lacking in the plastic surgery literature. A classification listing the extent of rhytidectomy performed is shown in ▶ Table 8.2, referencing flap and incision length as the parameters of assessment.
Mini-lift (mini-lifts are designated by either being short flap or short scar including minimal-incision approaches.) | ML type I (short flap) | Flap length from tragus < 3–4 cm |
ML type II (short scar/minimal incision) | The incision(s) are limited, not extending beyond the retrotragal component. A series of small incisions are also classified as short scar. | |
ML type III (combined) | Incorporates both short flap and short scar/minimal-incision components | |
Intermediate lift | IL type I | Flap length > 4 cm from tragus |
IL type II | Incision extends > 1 cm into posterior hairline | |
IL type III | Incorporates both longer flap and longer incision | |
Full facelift | FFL type I | Incorporates IL with extended dissection past zygomatic or mandibular cutaneous ligaments or to the nasal labial fold (NLF) |
FFL type II | Incorporates IL with extended (side-to-side) neck dissection | |
FFL type III | Incorporates both extended facial and neck dissection | |
Note: The use of terms to describe more limited rhytidectomy techniques should take into consideration: anesthesia requirements, operative time, complication risk, and recovery time. |
8.4.5 Type V: Concurrent Surgery for both Face and Neck (Cervicofacial Rhytidectomy)
FLL type V patients are characterized by subcutaneous laser-assisted cervicofacial flap elevation with or without interstitial laser lipolysis and liposculpture, and the performance of rhytidectomy procedures of the face and neck including midline platysmal plication or platysmaplasty.
8.5 Subcutaneous Optical Energy and Laser–Tissue Interaction
8.5.1 Hemostasis
The clinical observation of hemostatic effect of the fiber laser treatment of the facial flap in an early patient in 2007 led to a small clinical study that compared the 980-nm CW diode laser to the pulsed 1,064 Nd:YAG SmartLipo and SmartLipo MPX that utilized both the 1,064 and 1,320 nm in single wavelength or multiplexed mode. Split-face studies were done utilizing the same 10-W setting with the following findings: The diode laser was much more effective in passing through the superficial soft tissues when compared to the SmartLipo laser, but the hemostatic effects observed with the SmartLipo were not present in the diode laser. This was true when comparing the 980-nm diode to the 1,064 and 1,320 nm, or blended multiplexed 1,064/1,320-nm wavelengths. A similar comparison with the 1,440-nm wavelengths likewise demonstrated less hemostatic ability than the 1,064 or 1,064/1,320 nm blended. A quantitative comparison showed that the SmartLipo had roughly 5 times the hemostatic capability when compared to the diode laser based on sponge counts. An explanation of this is shown in the following illustrations. First when comparing the pulse characteristics of the SmartLipo laser, it is evident that high-peak powers are generated with each laser pulse. This is known as a gradient pulse (▶ Fig. 8.16). The high-peak energies are responsible for sealing off the blood vessels before the fiber laser divides the blood vessels, and the probability of vessel coagulation is related to the high-peak power of the pulsed laser (▶ Fig. 8.17). The 980-nm CW laser does not generate the high-peak power seen in the SmartLipo laser―whether the 1,064-nm or the 1,064/1,320-nm blended MPX unit or the Triplex or the 1,440-nm laser alone. When the laser is passed subcutaneously, it simply divides the vessel without cauterizing it (▶ Fig. 8.18), which leads to no advantage for the laser use if hemostasis is the desired end point. At meetings over the past several years, the author has seen clinical photos of fairly large hematomas during use of the 980-nm CW diode laser subcutaneously in the face. This is not surprising because, as demonstrated in the author’s study, the fixed-pulse, 980-nm fiber laser operating at 10 W and used subcutaneously does not provide substantial hemostatic effects. The author would be concerned about using the 980-nm CW diode laser on lower orbital fat due to the fact that lack of effective hemostasis in orbital fat vessels could lead to catastrophic complications. Complications with skin burns and full-thickness necrosis have been reported with the 980-nm diode laser when used without temperature monitoring.19
Fig. 8.16 Gradient pulse characteristics of SmartLipo and SmartLipo MPX. Wave configuration has much to do with the hemostatic properties of the investigated fiber lasers. The pulsed lasers generate higher peak powers for cauterization of facial blood vessels. (Reproduced with permission from Gentile RD, ed. Neck Rejuvenation. New York, NY: Thieme Medical Publishers; 2011:157.)
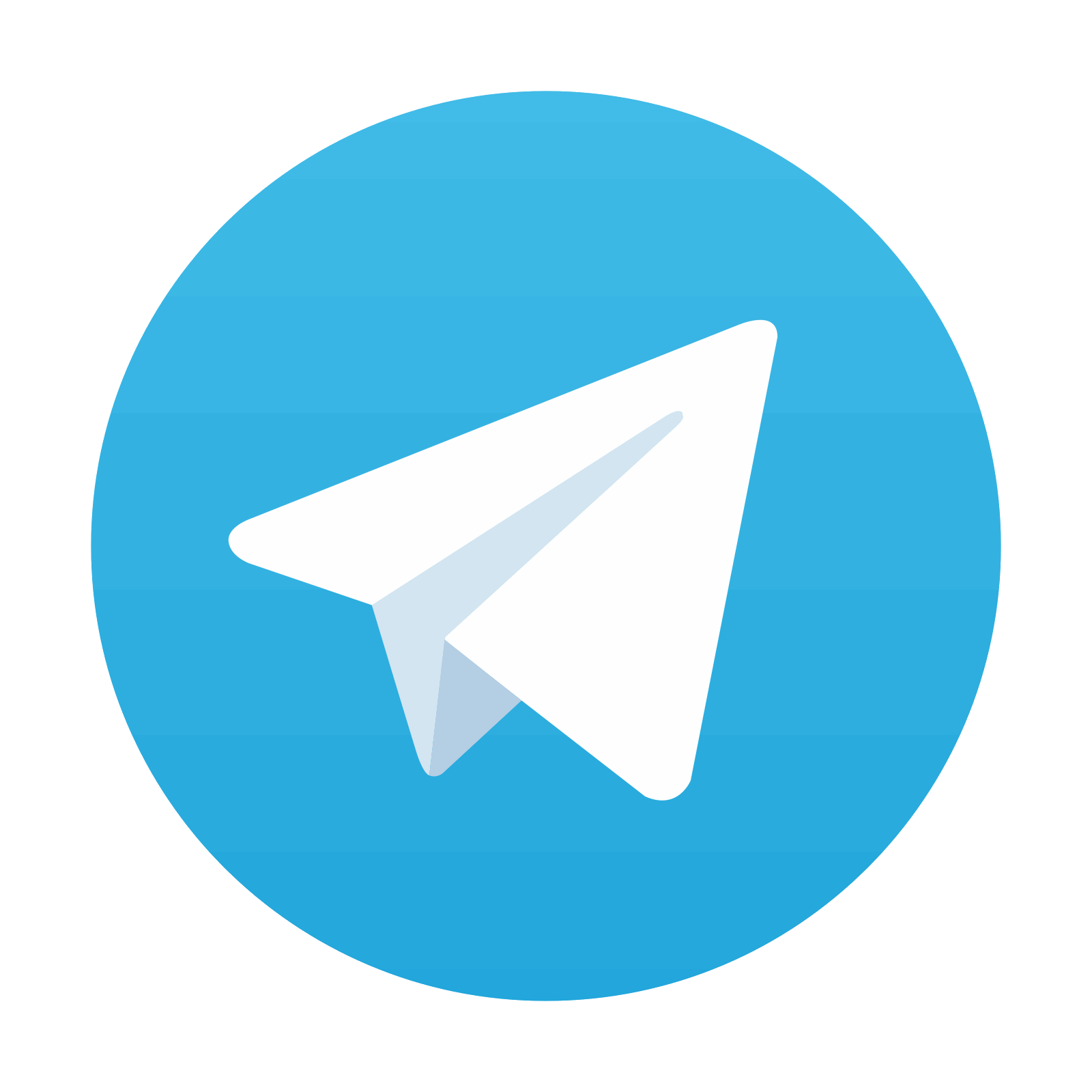