Cross-face nerve grafting enables the reanimation of the contralateral hemiface in unilateral facial palsy and may recover a spontaneous smile. This chapter discusses various clinically applicable strategies to increase the chances for good functional outcomes by maintaining the viability of the neural pathway and target muscle, increasing the number of reinnervating nerve fibers and selecting functionally compatible donor nerve branches. Adopting those strategies may help to further improve patient outcomes in facial reanimation surgery.
Key points
- •
Facial reanimation aims to improve static and dynamic facial symmetry by improving the resting muscle tone and dynamic muscle excursion in voluntary and spontaneous smiling.
- •
A challenge that often remains in facial reanimation surgery is combining sufficient muscle excursion and resting tone with spontaneous emotional expression.
- •
The viability of the target muscle and the neuronal pathway are essential for functional recovery.
- •
Providing a sufficient number of nerve fibers for muscle reinnervation may improve functional outcomes.
- •
Combining multiple axon sources for facial reanimation may capitalize the individual advantages of different donor nerves.
Introduction and background
Principles of Cross-Face Nerve Grafting
Cross-face nerve grafting describes a surgical nerve transfer technique that uses one or multiple nerve grafts to reroute donor nerve fibers across the median line of the face in order to reanimate contralateral target muscles in unilateral facial palsy. This facial reanimation technique is preferably used when suitable ipsilateral axon sources are unavailable.
If the proximal facial nerve stump on the paralyzed side cannot be used as an axon source, alternative axon sources may be transferred to the dysfunctional facial nerve in order to achieve reinnervation of the facial musculature. Such alternative axon sources can be nearby located cranial nerves including the hypoglossal nerve, the motor nerve to masseter. and even the accessory nerve. , Alternatively, because of the symmetric innervation pattern of the mimic musculature by the left and right facial nerve, cross-face nerve grafting allows the surgeon to use the contralateral facial nerve as an axon source in unilateral facial palsy. A cross-face nerve graft can be used to reinnervate the facial musculature either as the main axon source or augmentative as a “supercharging” nerve transfer (distal graft end to the side of the recipient facial nerve) for partially recovered facial nerves. Both procedures, however, require viable target muscles to restore motor function. If viable facial musculature is not available for reinnervation, such as following tumor resection, congenital/developmental syndromes, or long denervation times (>18 months), a free functional muscle transfer may be used to provide viable musculature into the paralyzed hemiface. A free muscle flap may be powered by ipsilateral axon sources or by a cross-face nerve graft in a 2-stage procedure. During the first stage, one or more “blind-ending” cross-face nerve grafts are tunneled and banked subcutaneously in the paralyzed hemiface, and the proximal graft end is connected to the contralateral facial donor nerve. Then, the donor nerve fibers are allowed to grow through the nerve graft for 6 to 12 months, depending on the chosen graft length, before a free functional muscle transfer is performed in stage II. In the second stage, the banked distal end of the cross-face nerve graft is connected to the motor nerve of the muscle graft or, if a neural pedicle is unavailable, directly sutured to the muscle to induce its reinnervation.
Therapeutic Goals of Cross-Face Nerve Grafting
Loss of facial nerve function affects the patient’s daily life in multiple ways. , The vulnerable ocular surface loses protection due to incomplete eyelid closure as a result of dysfunctional temporal and/or zygomatic facial nerve branches to the orbicularis oculi muscle. In addition, proximal facial nerve lesions located within the temporal bone may affect the parasympathetic facial nerve fiber population that supplies lacrimal and salivary glands as well as the taste receptors in the anterior two-thirds of the tongue. Parasympathetic facial nerve fiber dysfunction thus may result in insufficient lacrimation and salivation, “crocodile tears,” gustatory sweating, and impaired taste. However, arguably the most debilitating dysfunction for an affected patient’s daily life and their social interactions is the loss of facial expression. , Facial palsy not only results in impaired dynamic expression but also disables emotional expression, the ability to spontaneously react to emotional stimuli and to formulate clear speech in daily social interactions. There is also a decrease in static muscle tone, resulting in stigmatizing disfigurement. Facial nerve surgery therefore aims to improve static and dynamic facial symmetry by improving both the resting muscle tone and dynamic muscle excursion in voluntary and spontaneous smiling.
Challenges in Cross-Face Nerve Grafting
A challenge in facial reanimation surgery is combining sufficient muscle excursion and resting tone with the ability to spontaneously elicit an emotional facial response. Although ipsilateral non-VII cranial nerve transfers may restore powerful, voluntary muscle contractions, spontaneous and emotional facial expressions are often lacking. A major advantage of cross-face nerve grafting is the possibility of reanimating the paralyzed face via the contralateral facial nucleus, thereby enabling spontaneous and emotional smiling. However, cross-face nerve grafts can fail to provide meaningful levels of contractility and force in target muscles, and thus static and dynamic asymmetries may remain , ; this may in part be explained by the long regeneration distances and smaller donor motor neuron pools provided by cross-face nerve grafts compared with other sources. As a consequence, a reduced number of donor nerve fibers may reach the target muscles after a prolonged period of denervation compared with nerve transfers that use ipsilateral axonal sources.
Discussion—contributing factors to functional recovery and strategies to improve cross-face nerve grafting
Sufficient recovery of muscle function following denervation depends on a variety of factors. Key aspects that, at least to some extent, can be influenced by surgical decision-making are the muscles’ viability and receptivity to reinnervation, the number of motoneurons that successfully reconnect to the muscle fibers, and the source of motoneurons used for reinnervation.
Key Changes in Denervated Muscle and Nerve and Surgical Strategies to Maintain Their Viability
The viability of denervated musculature, as well as the motor nerves serving those muscles, is heavily time dependent. Although precise time windows are unknown, clinical guidelines and expert consensus presently suggest a maximum denervation time of 12 to 24 months. At this time, the chances for successful restoration of muscle function decline to a level where most surgeons favor alternative reanimation approaches, as reinnervation is more likely to fail; this is because denervated muscle fibers undergo profound cellular changes in response to denervation, including ionic imbalance, , decreased resting membrane potential, accelerated protein catabolism, permeabilization of the sarcolemma, and activation of the intracellular inflammatome. With increasing denervation time, the contractile apparatus progressively disintegrates , and the number of intramuscular mitochondria diminishes, with substantial decreases in contractile force. Simultaneously, the intramuscular capillary bed progressively degenerates in response to denervation, contributing to a hypoxic intramuscular environment and fibrotic remodeling of the denervated muscle. As a consequence, chronic denervation of muscle appears histologically as atrophic muscle fibers with a disorganized contractile apparatus, embedded in a dense meshwork of collagenous fibers and adipocytes. ,
Although some aspects of those degenerative changes, such as muscle mass, seem to be partly reversible by long-running external electrical muscle stimulation programs, attempts to reinnervate the long-term denervated muscle are usually less successful, most likely because the intramuscular neural pathways that are left behind the degenerated native nerve fibers become progressively less permissive for regenerating (donor) nerve fibers. The endoneurial tubes inside denervated nerve segments initially serve as guiding structures for regenerating axons and accommodate bands of proliferating, supportive Schwann cells providing a permissive regeneration environment for regrowing axons. In response to prolonged periods of axonal deprivation (“chronic denervation”), the Schwann cells atrophy and the endoneurial tubes are progressively occluded by collagen filaments. , This adverse intraneural regeneration environment, in combination with the disintegrated contractile apparatus, may explain the low success rate of delayed reanimation approaches.
To ensure target muscle viability, the denervation time should be reduced to a minimum, which generally implies early diagnosis and timely surgical intervention for procedures that aim at reanimating the paralyzed facial musculature. For long-term denervated muscles, repetitive electrical muscle stimulation may be used to externally induce contractions and thereby, at least in part, maintain the contractile apparatus until donor nerve fibers reach the muscle fibers. However, intramuscular and extramuscular neural pathway degeneration may still be present in electrically stimulated muscles, potentially limiting the merit of this procedure for facial palsy. Alternatively, one well-described strategy is to minimize the denervation time of the dysfunctional facial nerve and muscle by combining a cross-face nerve graft with a “babysitting nerve transfer.” An ipsilateral motor nerve, such as a portion of the hypoglossal nerve or the motor nerve to masseter, can be coapted end-to-end or end-to-side to the dysfunctional facial nerve to provide reinnervation and maintain a viable musculature. In the same procedure, a cross-face nerve graft is placed to reroute healthy contralateral facial nerve fibers to the dysfunctional facial nerve as a reverse end-to-side nerve transfer (distal graft end to the side of the dysfunctional facial nerve). By combining cross-face nerve grafts with ipsilateral axon sources, the time of axonal deprivation can be reduced, and patients may benefit from early restoration of muscle function.
Strategies to Increase the Number of Reinnervating Motoneurons
In facial reanimation surgery, the excursion, symmetry, and spontaneity of muscle contractions are key metrics for satisfactory results. The muscle excursion and thereby the dynamic symmetry depend on the number of motoneurons available for muscle reinnervation. If the muscle is reinnervated by a low number of neurons, muscle function partly recovers but force deficits often remain, presumably due to remaining denervated muscle fibers. Accordingly, muscles with moderate functional recovery tend to show a higher number of atrophic muscle fibers, and conversely, a higher number of transferred facial donor nerve fibers is associated with improved muscle function with regard to symmetry and excursion.
Reverse the nerve graft
A simple measure to increase the chance for donor nerve fibers to reach their target is using the nerve graft in a reversed orientation. This ensures that all endoneurial tubes guide the donor nerve fibers to the opposite end of the graft and thereby the target muscle. If instead the graft is used in its physiologic orientation, some endoneurial tubes may lead to former nerve branches. Such branches could have been cut during graft harvest and thus may not be immediately recognizable to the surgeon. Although it is presently unclear whether the concept of reversed graft orientation actually translates into functional improvements and whether those effect sizes would be detectable with currently available diagnostic tools in a reasonably sized clinical sample, we prefer to use grafts in a reversed orientation based on the before-mentioned considerations.
Select suitable donor nerve branches
In addition, in order to increase the number of neurons that eventually reinnervate the target muscle, the surgeon may use a donor nerve branch with a higher axonal load. Depending on the selected branch of the facial nerve and the proximodistal level of transection, the number of transferred donor nerve fibers can vary by a factor of 20, ranging from approximately 150 to 3000 myelinated axons. Following 10 to 14 months of nerve regeneration after stage I, approximately 20% of the transferred donor nerve fibers are detectable as myelinated axons at the distal end of the nerve graft. However, in the absence of a target muscle, a large proportion of axons that regenerated through the nerve graft seem to remain in a thinly or unmyelinated stage and mature after the nerve fibers are allowed to reinnervate the transferred muscle graft after stage II.
The relevance of rerouting a sufficient number of axons for muscle reanimation becomes evident when comparing the proportion of afferent and efferent axons in peripheral and cranial nerves. Even in nerves often referred to as “pure motor nerves” (eg, accessory or the phrenic nerve) the proportion of efferent (ie, motor) nerve fibers remains less than 30%. Although this highlights the relevance of afferent input for motor control, it also suggests that only the minority of the transferred facial nerve fibers are motor nerve fibers and thus capable of establishing a functional reconnection to the target muscle. Selecting donor nerve branches of appropriate diameter and axonal load may increase the number of provided motor nerve fibers and thus improve recovery of muscle function. Terzis and colleagues proposed approximately 900 myelinated axons as a reasonable cutoff above which they observed a higher rate of sufficient functional recovery after free functional gracilis transfer. Although greater than 90% of the intraparotid zygomatic nerve branches reliably provide more than 900 axons, the proportion of extraparotid zygomatic nerve branches with comparable axon counts rapidly declines in distal direction. Conversely, larger, proximal nerve branches may bundle axons that innervate different targets and thus lose functional specificity. To intraoperatively define the sweet spot that is neither too proximal, thereby losing specificity, nor too distal, thereby losing axonal load, remains challenging and may be subject to considerable uncertainty. Alternatively, surgeons may combine multiple axon sources for facial reanimation.
Combine multiple axon sources
The concept of supercharging aims to combine functional specificity with sufficient axonal load by combining 2 or more axon sources for a single target. Smaller, contralateral facial nerve branches with high functional specificity but insufficient axonal load may be augmented with strong ipsilateral axon sources. The ipsilateral motor nerve to masseter is an anatomically consistent and reliable donor nerve, containing 2700 myelinated nerve fibers on average, short regeneration distances, and thus rapid restoration of function within 3 to 5 months. , As a result, the motor nerve to masseter is frequently used to restore mimetic muscle function. Physiologically, the masseter is a strong muscle of mastication. Accordingly, facial muscles that have been reinnervated by a transferred masseter nerve often show strong contractility and correspondingly high oral commissure excursion, close to the maximum excursion of the healthy side, but often with poor resting tone and less emotional spontaneity. , Similarly, muscle grafts that are powered by the motor nerve to masseter often maintain a larger volume compared with muscles supplied by a cross-face nerve graft, which may indicate a lower proportion of muscle fibers that remain denervated and thus atrophic in nerve to masseter-powered muscles. Thus, combining a cross-face nerve graft with a strong and reliable axon source can increase the number of reinnervating nerve fibers and thus may optimize functional outcomes in facial reanimation surgery.
Although supercharging strategies mainly aim at a quantitative augmentation of smaller donor nerves, the emerging concept of “dual nerve transfers” combines 2 axon sources to capitalize the qualitative advantages of both donor nerves. , Beyond cross-face nerve grafting, dual nerve transfers have also been proposed for nonfacial axon sources such as the hypoglossal nerve (good muscle resting tone but limited commissure excursion) with the motor nerve to masseter (good muscle excursion but limited resting tone) for facial reanimation. In conclusion, combining multiple axon sources represents a promising chapter in facial reanimation surgery, and future research may inspire even more nuanced surgical concepts to treat facial palsy.
Enhance axonal regeneration
In addition, strategies to enhance the regeneration of the transferred axons may accelerate the reinnervation process and increase the proportion of neurons that reach the target muscle. Brief electrical stimulation (30–60 minutes, 20 Hz) of the transferred donor nerve, proximal to the coaptation site and immediately after or during nerve repair promotes the initial phase of axonal outgrowth and increases the number of nerve fibers that cross the repair site. However, the currently available intraoperative techniques require additional operative time to complete the stimulation protocol and the functional benefit of electrical stimulation in cross-face nerve grafting remains to be determined in ongoing studies. Another promising experimental strategy for enhancing nerve regeneration may be the local microdosing of proregenerative drugs directly at the nerve repair site. A recently developed biodegradable drug delivery system for sustained release of Tacrolimus (FK506) was found to accelerate the rate of axonal regeneration in preclinical nerve repair models with minimal off-target drug accumulation in other organs when compared with systemic drug delivery. Future clinical studies will determine the applicability of local drug delivery systems in peripheral nerve repair and facial reanimation.
Protect the pathway
As the endogenous growth support for the regenerating axons decreases in the distal segments of the nerve graft in response to prolonged axonal deprivation, strategies to maintain Schwann cell viability and prevent endoneurial tube occlusion may enhance nerve fiber growth through cross-face nerve grafts. The concept of distal pathway protection resembles the previously mentioned “babysitting” approach for denervated muscle. By connecting the distal end of the cross-face nerve graft to a small sensory nerve branch (eg, a branch of the infraorbital nerve), cellular constituents of the sensory nerve may repopulate the distal graft and maintain a permissive regeneration environment. , Despite encouraging preclinical evidence , and although distal pathway protection of cross-face nerve grafts has been shown to be surgically feasible without any detectable donor side deficit, clinical outcome studies are not yet available.
Similarly, aiming at an enhanced growth support for the regenerating axons on their route to the target muscle, some investigators hypothesize that it may be of advantage to increase the length of the neural pedicle of the free muscle flap at stage II of the procedure and shorten the length of the cross-face nerve graft at stage I proportionately, thus providing the regenerating axons with a fresh regeneration environment and decreases the distance needed to traverse within the banked sensory nerve graft. Although compelling in theory, the ideal “nerve graft to pedicle length ratio” for maintaining an optimized growth environment for the regenerating axons and its clinical benefit remains yet to be determined.
Selecting the Appropriate Donor Nerve for Facial Reanimation
Controllability, symmetry, and spontaneity of facial muscle function following reanimation surgery depend on the source of reinnervating neurons. Although the motor nerve to masseter is a reliable axon source, the jaw closing masseter muscle is usually not involved in emotional expression. This lack of integration into emotional neuronal circuits reflects in a low proportion of patients who regain the ability for spontaneous smile and emotional facial expression when the motor nerve to masseter is used for facial reanimation. To regain spontaneity with nonfacial donor nerves, central neuronal circuits need to undergo substantial reorganization. Individual subsets of a neuron population previously responsible for the motor control of a single muscle now need to be recruited independently and embedded into multiple, new, and functionally distinct neuronal circuits for mimetic motor control. The ability of the central nervous system to fundamentally reorganize its neuronal circuits is highly age dependent and declines rapidly in adults, potentially hampering the functional outcome of the facial reanimation procedure.
Cross-face nerve grafting may reduce the necessary extent of central reorganization because the “rewired” contralateral facial neurons belong to a population that is already integrated into motor control circuits for mimic and emotional facial expression. From a neurophysiological perspective, the cortical motor control of spontaneous facial expression is primarily organized along a horizontal axis. The facial expression of primary emotions, including happiness, interest, surprise, fear, anger, disgust, contempt, and sadness is controlled independently for the upper and lower part of the face, and this allows infants, and to some extent adults, to display a blend of 2 different emotions simultaneously on the upper and lower face. Commonly observed blends of facial expressions in infants are interest and surprise (raised eyebrows with eye opening) on the upper half of the face, coupled with joy, sadness, or anger on the lower face. In contrast, the expression along the vertical facial axis is symmetric, particularly when positive emotions are expressed. Accordingly, it seems to be challenging to display 2 different emotions in the left and right hemiface simultaneously. This lateral symmetry of emotional expression underpins the suitability of contralateral facial nerve fibers for the restoration of a naturally appearing smile and spontaneity in facial reanimation surgery.
To further increase functional specificity, the nerve surgeon can selectively target subsets of neurons with high functional compatibility with their contralateral target muscle by individually mapping the function of facial donor branches via intraoperative nerve stimulation. As such, muscle co-contractions often resemble familiar activation patterns that frequently occur simultaneously in normal facial expression.
Further, the contractile properties of muscle fibers are (re-)defined by the reinnervating neuron population. Fiber type compositions can differ substantially among different muscles, and thus, muscle fiber composition and consequently the contractile properties may change following nerve transfers. Although the clinical relevance of fiber type composition in facial reanimation surgery is incompletely understood, transferring contralateral facial nerve fibers may theoretically result in closer resemblance to the original fiber type composition and contractile properties compared with alternative donor nerves.
Summary
In conclusion, cross-face nerve grafting enables reanimation of the contralateral hemiface in unilateral facial palsy and may recover a spontaneous smile. Various strategies can be adopted to increase the chances for good functional outcomes by maintaining the viability of the neural pathway and target muscle, increasing the number of reinnervating nerve fibers and selecting functionally compatible donor nerve branches. Beyond that, preclinical research has produced a promising group of novel therapies to further enhance nerve regeneration. Future studies will determine their clinical applicability and therapeutic benefit aiming to further improve patient outcomes and push the boundaries in facial reanimation surgery.
Clinics care points
- •
A key advantage of cross-face nerve grafting is the ability to use the contralateral facial nerve fiber population, which may enable spontaneous and emotional smiling following facial reanimation surgery.
- •
Supercharging a dysfunctional but partially recovered facial nerve with a cross-face nerve graft can supplement functional gains and thus increase static and dynamic symmetry even years after injury.
- •
Oral commissure excursion when smiling often remains less than normal levels (approximately 60% of normal excursion) when a cross-face nerve graft is used, but can return to normal levels when the masseteric nerve is used as an additional input to power the muscle.
- •
Augmenting a cross-face nerve graft with a nonfacial donor nerve may combine spontaneity and sufficient muscle excursion.
- •
The number of donor nerve fibers available for muscle reinnervation is positively correlated with muscle force and excursion.
- •
Promising strategies to enhance axonal regeneration following nerve surgery are on the horizon. , , , ,
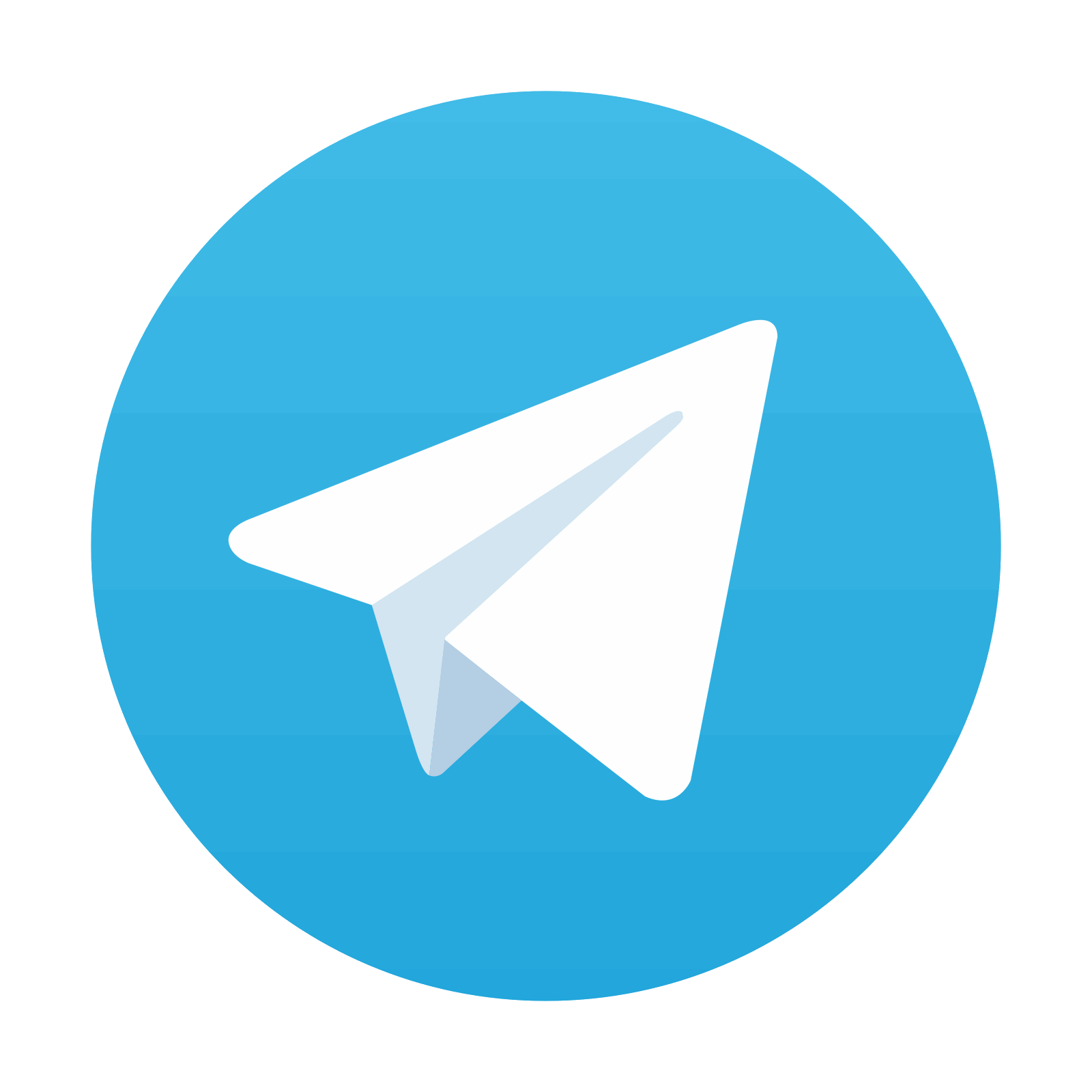
Stay updated, free articles. Join our Telegram channel

Full access? Get Clinical Tree
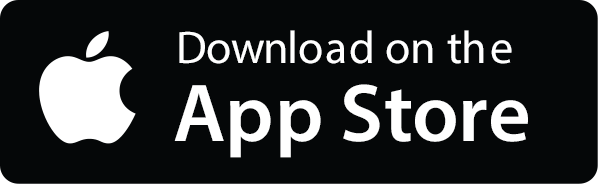
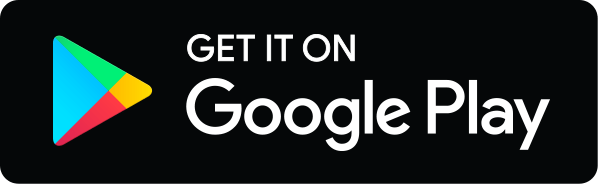
