Skin as an Organ of Protection: Introduction
|
The skin’s most important function is to form an effective barrier between the “inside” and the “outside” of the organism. Life on dry land requires the presence of a barrier to regulate water loss and prevent desiccation, commonly referred to as the “inside–outside” barrier. Additionally, skin provides an “outside–inside” barrier to protect against mechanical, chemical, and microbial assaults from the external environment (Fig. 47-1).1 To perform these functions the epidermis undergoes keratinization, a process in which epidermal cells progressively mature from basal cells with proliferative potential to the lifeless, flattened squames of the stratum corneum (SC) (Fig. 47-2). Both the SC and the deeper skin layers protect the skin from mechanical forces, ultraviolet (UV) radiation, cold and hot temperatures, and invasion of chemicals and microbes. To effectively perform this multiplicity of functions, the skin contains different types of barriers. The physical barrier consists mainly of the SC, but the nucleated epidermis, in particular the tight junctions, provides another important barrier component. The chemical/biochemical (antimicrobial) barrier consists of lipids, acids, lysozymes, and antimicrobial peptides (discussed in Chapter 10). The humoral and cellular immune system provides a barrier to infectious disease, but immune hyperactivity may lead to allergy (Table 47-1).
Physical barrier | Stratum corneum (corneocytes, lipid lamellae), nucleated epidermis (desmosomes, tight junctions) |
Chemical/biochemical (antimicrobial) barrier (innate immunity) | Lipids, organic acids, lysozymes, antimicrobial peptides |
Immune barrier humoral and cellular immune systems | Lymphocytes, neutrophils, monocytes, Langerhans cells |
Although the skin is of central importance for preventing water loss in a dry environment, aquatic animals also require a skin barrier to protect them from the high salinity of their surrounding environment. Terrestrial mammals with dense fur have much thinner skin than animals without this protective coat, demonstrating that fur itself is a considerable barrier. The relatively hairless skin of pigs shows much similarity to human skin and is therefore a good model for skin research.
In addition to the SC the entire skin, as a whole, serves a protective function. The innermost region of human skin, the subcutaneous fat layer, offers mechanical shock protection, insulates the body against external heat and cold, and is active in general energy metabolism and storage. The dermis is composed of collagen bundles and elastic fibers and is very important for the mechanical strength of the skin. The epidermis, the skin’s outer layer, consisting primarily of stratified nucleated keratinocytes and the SC, is most important for skin protection—the focus of this chapter. Sweat glands and blood vessels regulate body temperature. Sebaceous glands secrete specialized lipids to protect the hair from the environmental stress (see Chapter 79). In animals, these lipids serve as a water repellent for the fur, aiding in buoyancy and temperature regulation, and also preventing desiccation of the body and UV damage. The role of sebaceous lipids for SC barrier function and for dry skin is under active investigation.2,3 Sebaceous glands also transport glycerol to the skin surface, which is important for SC hydration.4 Nerve fibers are chemosensitive and act as a warning system against external trauma (see Chapter 102).
Structure of the Stratum Corneum: The Physical Permeability Barrier
Functions | Structural Bases | Biochemical Mechanisms |
---|---|---|
Permeability barrier | Lamellar bilayers | Hydrophobic lipids |
Mechanical integrity/resilience | Cornified envelope, cytosolic filaments | Cross-linked peptides; e.g., involucrin, loricrin. Keratin filaments |
Hydration | Lamellar bilayers; corneocyte cytosolic matrix | Sebaceous gland-derived glycerol; filaggrin breakdown amino acids; natural moisturizing factors (NMFs), hornerin |
Cohesion/desquamation | Corneodesmosomes | Serine proteases |
Antimicrobial defense | Lamellar bilayers; extracellular matrix | Free fatty acids; antimicrobial peptides |
UV protection | Corneocyte cytosol | Structural proteins; trans-urocanic acid (tUCA) |
Antioxidant defense | Corneocytes and extracellular matrix | Keratins; sebaceous gland-derived vitamin E and other antioxidants |
Waterproofing/repellence | Lamellar bilayers | Keratinocyte and sebum-derived lipids |
Cytokine signaling | Corneocyte cytosol | Storage and release of pro-IL-α; serine proteases |
Xenobiotic defense | Lamellar bilayers | Lipid solubility; cytochrome P450 system (outer epidermis) |
Research from the 1940s and 1950s established that the SC is the specific location of the physical barrier.5,6 The SC barrier structure visualized by electron microscopy and ruthenium tetroxide fixation (Fig. 47-3) is a better indicator of its barrier function than the typical “basket-weave” appearance of the SC in routine formalin-embedded tissue sections. Regulation of water permeation is not absolute. The normal movement of water from the SC into the atmosphere is known as transepidermal water loss (TEWL), previously called insensible water loss. The SC serves as the principal barrier against the percutaneous penetration of chemicals and microbes1 as well.
Figure 47-3
Electron microscopy reveals that in the stratum granulosum (SG)–stratum corneum (SC) interface the lamellar bodies (LB) content is extruded to the intercellular space (A), thus forming continuous bilayers. (B then C). Desmosomes are becoming corneosomes in the process of cornification (D).
The 10–20 μm thick SC forms a continuous sheet of protein-enriched cells, embedded in an intercellular matrix, enriched in nonpolar lipids, and organized as lamellar lipid layers. The viable epidermis is a stratified squamous epithelium, consisting of basal, spinous, and granular cell layers. Upon leaving the basal layer, keratinocytes begin to differentiate and undergo a number of changes in both structure and composition during the apical migration into the stratum spinosum and stratum granulosum (see Chapter 46). Keratinocytes synthesize and express numerous structural proteins and lipids during their maturation. The final steps in keratinocyte differentiation are associated with profound changes in their structure, resulting in their transformation into flat and anucleated squamous cells of the SC, consisting mainly of keratin filaments and surrounded by a cell envelope composed of cross-linked proteins (cornified envelope proteins) as well as a covalently bound lipid envelope (Fig. 47-2). Extracellular nonpolar lipids surround the corneocytes to form a hydrophobic matrix. The cornified envelope proteins as well as the covalently bound lipid envelope are thought to be important for the chemical resistance of the corneocytes (Fig. 47-4). Desmosomes, which interconnect adjacent keratinocytes, are important for SC cohesion and are shed during the desquamation process in the SC. In the upper spinous and granular layers characteristic lamellar vesicles appear, which are called epidermal lamellar bodies (Figs. 47-3 and 47-5). These are enriched in polar lipids, glycosphingolipids, free sterols, phospholipids, and hydrolytic enzymes that deliver the lipids required for the SC’s extracellular layers. The lamellar bodies may also contain proteins such as human β-defensin 2.7 In response to certain signals, for example, an increase in calcium concentration during the transition from the granular layers to the SC, the lamellar bodies move to the apex of the uppermost granular cells, fuse with the plasma membrane, and secrete their content into the intercellular spaces through exocytosis. The lipids derived from the lamellar bodies are subsequently modified and rearranged into intercellular lamellae positioned approximately parallel to the cell surface. The covalently bound lipid envelope acts as a scaffold for this process. After the extrusion of the lamellar bodies into the stratum granulosum–SC interface, the polar lipids are enzymatically converted into nonpolar products. Hydrolysis of glycosphingolipids generates ceramides while phospholipids are converted into free fatty acids. These changes in lipid composition and cell structure result in the formation of a very dense structure packed into the interstices of the SC (Table 47-2).8
Figure 47-5
During differentiation, the upper stratum spinosum (SS) and the stratum granulosum (SG) cells generate lamellar bodies containing preformed lipid structures and hydrolytic enzymes. Their content is extruded into the SG–stratum corneum (SC) interface and undergoes profound remodeling. SB = stratum basale.
Confocal laser scanning microscopy and X-ray microanalysis studies have shown that the major route of penetration results in a tortuous pathway between the corneocytes, confirming that intercellular lipids play an irreplaceable role in regulating skin permeability barrier function.8 The major lipid classes in the SC are cholesterol, free fatty acids, and ceramides (see eFig. 47-5.1).9–11
Cholesterol is probably the most abundant lipid in the entire body and part of the plasma membrane, but also part of the intercellular lipid lamellae in the SC. Although basal cells are capable of resorbing cholesterol from circulation, most cholesterol in the epidermis is synthesized in situ from acetate.12 The epidermal keratinocyte, the main cell type in the epidermis, is highly active in the synthesis of several lipids, including cholesterol and free fatty acids. The rate-limiting step in cholesterol biosynthesis is catalyzed by hydroxymethylglutaryl CoA (HMG CoA) reductase (Fig. 47-6). Epidermal cholesterol synthesis is regulated by these enzymes and increases during permeability barrier repair.13
The skin contains free fatty acids as well as fatty acids bound in triglycerides, phospholipids, glycosylceramides, and ceramides. The chain length of free fatty acids in the epidermis ranges from C12 to C24. The rate-limiting enzymes acetyl-CoA carboxylase and fatty acid synthase in the epidermis are largely autonomous (Figs. 47-6 and 47-7).14 Saturated and monounsaturated fatty acids are synthesized in the epidermis, in contrast to di- and polyunsaturated acids. The nomenclature of the fatty acids is determined from the position of the first double bond in the molecule, starting from the terminal methyl group. In particular, the essential ω-6-unsaturated acids are obtained from food and reach the epidermis by the circulation but can also be obtained by topical treatment. The nonessential monounsaturated fatty acid, the oleic acid, is an ω-9-fatty acid. The most important double unsaturated fatty acid, linoleic acid, is an ω-6 fatty acid. Also of importance is α-linoleic acid (ω-3). No skin changes due to ω-3-fatty acid deficiency are currently known; however, it has been proposed that these fatty acids are important for the resolution of inflammation. ω-3-fatty acids are obtained from fish, whereas ω-6-fatty acids are obtained from plant oils.15,16 Essential fatty acid deficiency (EFAD) caused by unusual diets or malabsorption in humans or experimentally induced in rats and mice leads to the EFAD syndrome, characterized by profound changes in epithelia including the epidermis.17 In this condition, the epidermis is rough, scaly, and red and shows a severely disturbed permeability barrier function. In addition, severe bacterial infection, impaired wound healing, and alopecia may occur. Linoleic acid is part of phospholipids, glucosylceramides, ceramide 1, ceramide 4, and ceramide 9.18 It has been proposed that the linoleic acid metabolite γ-linoleic acid is of special importance for atopic eczema.
Ceramide is an amide-linked fatty acid containing a long-chain amino alcohol called sphingoid base. The carbon chain lengths of amide-linked fatty acids and sphingoid bases in most mammalian tissues are 16 to 26 and 18 to 20, respectively (see eFig. 47-5.1). Although sphingolipids, including glycosphingolipids and phosphosphingolipids, are ubiquitously distributed in mammalian tissues, tissue-specific molecular distribution has been described. Glucosylceramide is enriched in the epidermis and spleen while galactosylceramide is enriched in the brain, but is not detected in keratinocytes. Whereas ceramide is a minor lipid component, comprising less than 10% of cholesterol or phospholipids in other mammalian tissues, ceramide is a major lipid component in the SC, accounting for 30%–40% of lipids by weight. Moreover, such a high content of ceramides in the SC is not observed in the epidermal stratum granulosum, stratum spinosum, or stratum basale. This also suggests that terminal differentiation is a key factor in accumulating ceramide. The SC contains at least nine different ceramides.18 In addition, there are two protein-bound ceramides: (1) ceramide A and (2) ceramide B (see eFig. 47-5.1).8 These ceramides are covalently bound to cornified envelope proteins, most importantly to involucrin.
Ceramides are synthesized by serine-palmitoyltransferase as rate-limiting enzyme and by hydrolysis of both glucosylceramide (by β-glucocerebrosidase)19 and sphingomyelin (by acid sphingomyelinase) (Figs. 47-7 and 47-8).20 Whereas all kinds of ceramides are derived by synthesis from serine-palmitoyltransferase and from β-glucocerebrosidase, only ceramide 2 and ceramide 5 are obtained from sphingomyelinase because sphingomyelinase contains nonhydroxy acids.21
Keratinocytes require abundant cholesterol for cutaneous permeability barrier function. ABCA1 is a membrane transporter responsible for cholesterol efflux and plays a pivotal role in regulating cellular cholesterol levels. It was demonstrated that ABCA1 is expressed in cultured human keratinocytes and murine epidermis. Liver X receptor (LXR) activation and activation of peroxisome proliferator-activated receptor (PPAR)-α, PPAR-ss/δ, and retinoid X receptor (RXR) increased ABCA1 expression in keratinocyte cultures. Thus, cholesterol levels for permeability barrier function are regulated by ABCA1, LXR, and PPARs.22 The cellular fatty acid transport and metabolism is regulated by fatty acid-binding proteins (FABPs).23,24
Additional protective functions of the epidermis are not discussed in this chapter, but are listed in Table 47-3.
Functions | Biochemical Correlates |
---|---|
Antimicrobial systems | Antimicrobial peptides and lipids, iron-binding proteins, complement |
Antioxidants | Glutathione, oxidases, catalase, cytochrome P450 system, vitamins C and E |
Inflammatory mediators | Prostaglandins, eicosanoids, leukotrienes, histamine, cytokines |
UV-absorbing molecules | Melanin, trans-urocanic acid, vitamin D, vitamin C metabolites, filaggrin metabolites |
Xenobiotic-metabolizing enzymes | Glucoronidation, sulfation, hydroxylation mechanisms |
Epidermal Proliferation and Differentiation in Skin Barrier Function
To provide the physical barrier of the SC, not only intercellular lipids, but also corneocytes are of crucial importance.25,26 The epidermis undergoes keratinization in which epidermal cells progressively mature from basal cells with proliferative potential to lifeless flattened squames of the SC (Fig. 47-2). Keratinocytes arise from stem cells in the basal layers and transient amplification cells and move to a series of differentiation events until they are finally brought to desquamation.27 Thus, in the normal epidermis, there is a balance between the processes of proliferation and desquamation that results in a complete renewal approximately every 28 days. In some forms of ichthyosis, the rate of desquamation may be decreased, leading to epidermal cell retention (retention hyperkeratosis).28 (See Chapter 49.) In inflammatory skin diseases like psoriasis there is an increase in proliferation resulting in a disturbance in differentiation and parakeratotic squames (hyperproliferative hyperkeratosis).29
Keratins are major structural proteins synthesized in keratinocytes (see Chapter 46). They assemble into a web-like pattern of intermediate filaments that emanate from a perinuclear ring, extend throughout the cytoplasm, and terminate at junctional desmosomes and hemidesmosomes. During the final stages of normal differentiation, keratins are aligned into highly ordered and condensed arrays through interactions with filaggrin, a matrix protein. In keratin disorders, the filament networks collapse around the nucleus, preventing attachment with the filament-matrix complex and the inner surface of squames, and alter interaction between neighboring cells, thereby affecting desquamation. Filaggrin aggregates the keratin filaments into tight bundles. This promotes the collapse of the cell into a flattened shape, which is characteristic of corneocytes in the cornified layer. Together, keratins and filaggrin constitute 80%–90% of the protein mass of mammalian epidermis.25,26
The structural proteins involucrin, loricrin, trichohyalin, and the class of small proline-rich proteins (SPRRs) are synthesized and subsequently cross-linked by transglutaminases to reinforce the cornified envelope just beneath the plasma membrane. The proteins of the cornified envelope constitute about 7%–10% of the mass of the epidermis. These corneocytes provide the bulwark of mechanical and chemical protection, and together with their intercellular lipid surroundings, confer water-impermeability. The cornified cell envelope is a tough protein/lipid polymer structure formed just below the cytoplasmic membrane and subsequently resides on the exterior of the corneocytes (Fig. 47-4). It is resistant to 10% KOH and is the rigid structure seen on KOH skin scrapings. It consists of two parts: (1) a protein envelope and (2) a lipid envelope. The protein envelope contributes to the biomechanical properties of the CE as a result of cross-linking of specialized cornified envelope structural proteins by both disulfide bonds and N(ϵ)-(γ-glutamyl)lysine isopeptide bonds formed by transglutaminases.26,30 The isopeptide bonds are resistant to most common proteolytic enzymes. The corneocyte-bound lipid envelope is plasma membrane-like structure, which replaces the plasma membrane on the external aspect of mammalian corneocytes.31 Involucrin, envoplakin, and periplakin serve as substrates for the covalent attachment of ω-hydroxyceramides with very long-chained N-acyl fatty acids by ester linkage.32 These not only provide a coating to the cell, but also interdigitate with the intercellular lipid lamellae (Table 47-2).26
Experimental Barrier Disruption and Gene Modification in Epidermal Differentiation
Experimental barrier disruption leads to changes in epidermal differentiation, epidermal lipid keratin, and cornified envelope protein expression and, vice versa, overexpression and deficiency of these lipids and proteins in mice result in barrier defects. A number of diseases displaying defective epidermal barrier function are also the result of genetic defects in the synthesis and metabolism of either lipids, keratins, cornified envelope proteins, or the transglutaminase 1 cross-linking enzyme.
Inhibition of HMG CoA reductase by topical application of the lipid-lowering drug lovastatin in mice resulted in a disturbed barrier function and in epidermal hyperproliferation. Therefore, the specific relationship between barrier function and epidermal DNA synthesis was examined. After acute skin barrier disruption (local acetone treatment or by tape-stripping) (Fig. 47-9) and in a model of chronic barrier disruption (EFAD diet), an increase in DNA synthesis leading to epidermal hyperplasia was noticed.33 The increase in DNA, and in lipid synthesis, was partially prevented by occlusion.15,33,34
Figure 47-9
Three phases of barrier recovery with distinct metabolic activities occurring after acute barrier disruption. A = Secretion of preformed pool of lamellar bodies (0 to 30 minutes). B = Increased lipid synthesis (free fatty acids, ceramide, and cholesterol) (30 minutes to 6 hours), accelerated lamellar body formation and secretion (2 to 6 hours). C = Increased glucosylceramide processing (9 to 24 hours), increased keratinocyte proliferation and differentiation (16 to 24 hours).
Also, the described acute and chronic barrier disruption leads to specific changes in epidermal keratin and cornified envelope protein expression. Increased expression of the basal keratins K5 and K14 and a reduction of the differentiation-related keratins K1 and K10 were noted. In addition, there was expression of the proliferation-associated keratins K6 and K16 as well as the inflammation-associated keratin K17 (see eFig. 47-9.1).35 The importance of keratins for skin barrier function was supported by studies in K10-deficient mice. Heterozygotes and homozygotes showed a mild or severe permeability barrier disruption, respectively. Importantly, homozygous neonatal K10-deficient mice exhibited an extremely delicate epidermis and died a few hours after birth. Heterozygous littermates showed a normal skin at birth but developed increasing hyperkeratosis as they grew up.36
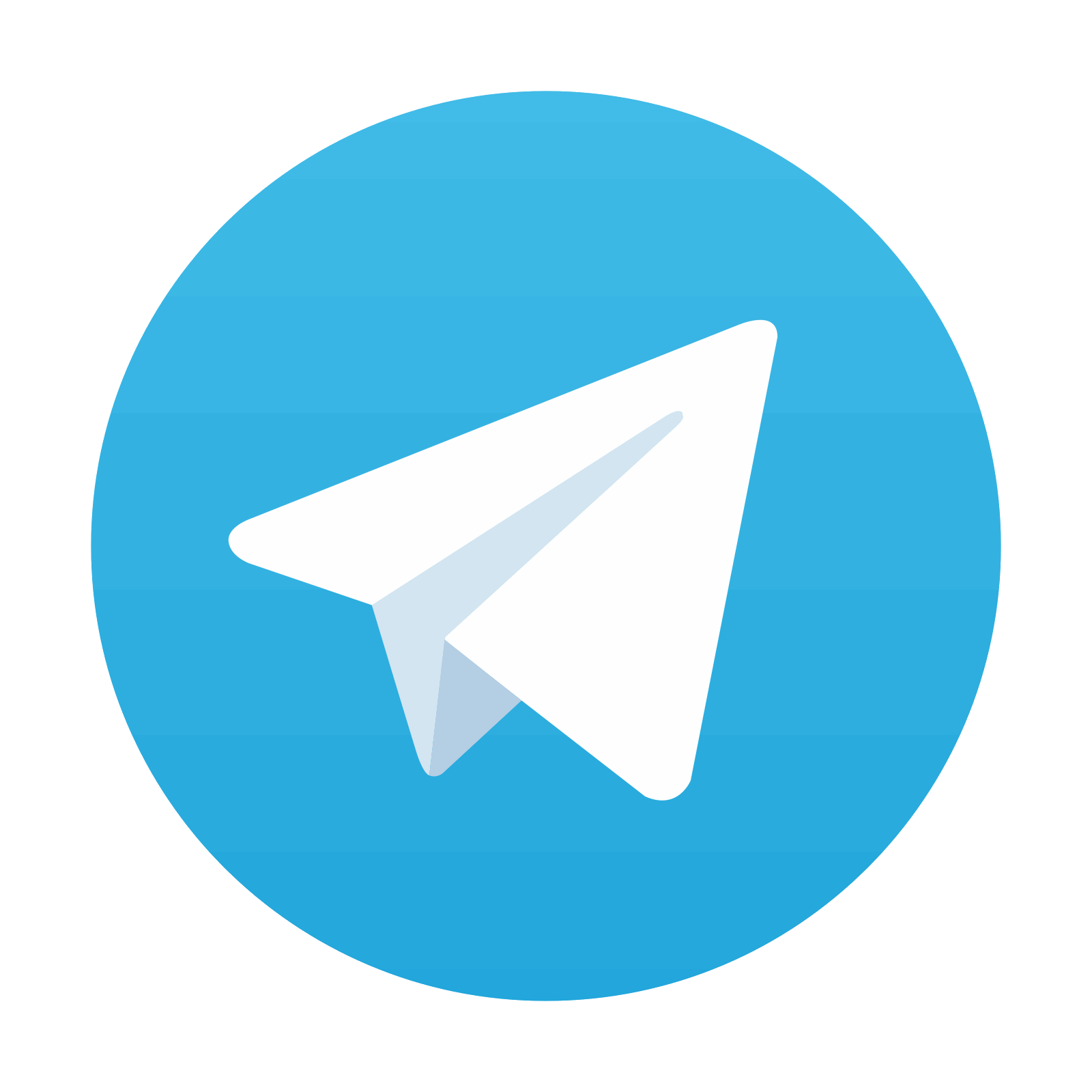
Stay updated, free articles. Join our Telegram channel

Full access? Get Clinical Tree
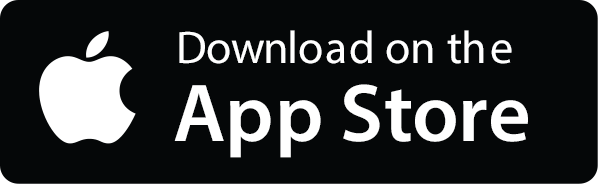
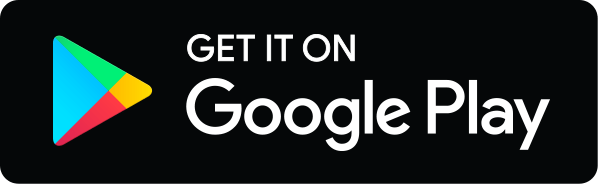
