History of Tattooing
The history of tattooing dates back to a time as ancient as civilization itself, when this highly symbolic method of expression afforded more than a mere touch of individuality. The iceman Ötzi, the 5,200-year-old European Bronze-Age mummy discovered in northern Italy, was covered in punctate tattoos dispersed throughout the skin over his arthritic joints, incurred from multiple ritualistic, therapeutic interventions. In ancient Egypt, dermal tattoos branded criminals, stigmatized prostitutes, and marked social rank. In the 17th century, as European explorers settled into overseas colonies, the practice of tattooing was gradually assimilated into Western culture, with the word tattoo itself deriving from ta-tau, Tahitian for “the results of tapping.” With the Industrial Revolution, the mechanization of conventional tattooing tools allowed for more precise and varied patterns, while the incorporation of multiple pigments provided for multicolored designs. The embrace by popular culture, the military community, and private groups and gangs eventually culminated in the widespread cultural phenomenon that has become evident today.
14.2 Classification of Tattoos
Despite the highly decorative nature of the majority of modern-day tattoos, different types exist (▶ Fig. 14.1), with strong implications for removal technique, clearance, and complications. Decorative tattoos constitute the vast majority of those presenting for removal and can be further subdivided into professional and amateur. Modern professional tattoos reflect a mechanized but refined method of dermal puncturing that incorporates diverse needle-tip sizes and configurations, colorful synthetic pigments, and a standardized sterile technique of dermal ink impregnation. Professional tattoo pigments are purposely introduced into the skin at sufficient depths and volumes that secure long-lasting results. These features promote greater pigment retention and, as a result, render the laser tattoo-removal process significantly more challenging than that seen with amateur tattoos.
Fig. 14.1 Examples of different tattoo types. (a) Professional decorative tattoo. (b) Amateur decorative tattoo. (c) Cosmetic tattoo (permanent makeup). (d) Traumatic tattoo.
Amateur techniques have existed for centuries and represent a more imprecise method of dermal tattooing, often relying upon various carbon-based compounds such as soot, graphite, or India ink for coloration. With their characteristic uniformly black hues, amateur techniques were adopted overseas by American military servicemen, who then subsequently introduced them to growing factions of the U.S. population, such as motorcycle groups and gangs. Amateur tattoo pigments often have more superficial distributions, lower ink volumes, and less-polished designs. As a result, these tattoos, with their traditional dark blue and black pigmentations, often respond well to laser removal.
Cosmetic tattoos have slowly garnered widespread popularity within the realm of permanent makeup, serving to imitate lip or eyeliner, enhance eyebrow definition and shape, and disguise scars or dermal lesions. Permanent makeup has the added benefits of being relatively unchanging, waterproof, and time-saving. They are typically applied by a tattoo artist or a dermatologist and often employ a variety of red, pink, and flesh color tones containing titanium dioxide and ferric oxide, both of which, incidentally, are known to predispose to paradoxical tattoo darkening upon laser exposure.
Medical tattoos are frequently applied to define radiation ports in patients undergoing radiotherapy or as personal identification and informative medical tags. Traumatic tattoos are incurred following superficial dermal trauma resulting from blast exposures, such as firearm or firework explosions and motor vehicle accidents with occupant ejection. As small, high-velocity particles penetrate the injured and exposed dermis, they become permanently lodged within the skin following re-epithelialization. The dermal impregnation of these particles of widely varying caliber yields a permanent tattoo. Often, because these embedded fragments are sulfur-containing, they place the individual at risk for localized reactions, ignition, and even blasts during attempted laser removal.1
Regardless of tattoo type, the underlying pathophysiology is characterized by the introduction of pigment-containing particles into the superficial and mid-dermis. This is followed by the gradual but long-lasting incorporation of ink particles into resident fibroblasts and macrophages. Understanding particle properties such as size, absorption spectra, dermal depth, and chemical pigment composition is essential toward achieving maximum tattoo clearance and minimizing complications following laser-tattoo removal. These factors are largely dictated by the tattooing process, and a thorough knowledge of the procedure will assist the cosmetic surgeon in navigating the multiple treatment options and laser settings.
14.3 Technique
The process of dermal tattooing can be defined as the controlled introduction of pigment particles into the dermis through the action of traumatic micro-puncturing of the skin. Modern-day tattoo artists rely heavily on the electric tattoo machine to create elaborate patterns and designs, whereas the variety of pigmented compounds provides for colorful depictions. The electric tattoo machine consists of one or more fine needles (diameter 200–350 μm) contained within a hollow tube. The needle group is soldered onto a bar, which is itself attached to an oscillating unit, allowing for the vertical vibration of the needle tips into and out of the tube. When applied to the skin, each needle produces hundreds of micro-punctures measuring approximately 1 to 2 mm in depth, sufficient to reach the papillary and superficial reticular dermis.2 When the tip of the needle is immersed into the ink prior to skin application, its action permits the introduction of the pigment into the dermal layer. Tattoo artists use several needle groupings to create different effects. Single needles are used for lining, groups of three or more needles are used for shading, and rows of needles for feathering. Following completion of the work, a healing period over 1 to 2 weeks is allowed to pass before the final appearance is appreciated.
14.4 Histopathology
The sequence of events that follows the placement of a dermal tattoo is similar to any other traumatic injury to the skin (▶ Fig. 14.2). As the tattoo needle punctures the dermis, cellular injury releases mediators that evoke the early inflammatory response. Despite the introduction of pigment particles, the influx of inflammatory cells is only mild due to the particles’ low immunogenicity. Within 24 hours after tattooing, the epidermis begins to peel, akin to that seen with a second-degree burn. In the process, a moderate amount of the deposited tattoo pigment is lost through epidermal shedding and dermal transudation, as well as due to the immediate action of dermal macrophages, which distribute small pigment particles to regional lymph nodes. After 10 to 14 days, the epidermis has fully healed and the majority of the pigment now resides within the superficial and mid-dermis. With passing months and years, due to the chronic phagocytic action of dermal macrophages, the distribution of the remaining pigment particles becomes limited to perivascular fibroblasts and resident phagocytic cells.3 It is this restricted distribution of pigment particles within long-lived dermal fibroblast and phagocytic cells that gives the tattoo its ultimate long-lasting qualities.
Fig. 14.2 Microscopic features of the tattooing process. (a) Dermal tattooing is achieved via the traumatic micro-puncturing of the dermis, depositing ink pigment into the superficial and mid-dermal regions. (b) Within 24 hours, an acute inflammatory phase sets in, characterized by epidermal sloughing and mild lymphocytic infiltrates. Early pigment clearance results from transcutaneous ink escape as well as by phagocytic and lymphatic clearance. (c) A fully healed tattoo represents dermal impregnation of the dermis with ink pigment, covered by a pigment-free epidermal layer. Following chronic phagocytic and lymphatic clearance, dermal ink particles become restricted to within perivascular resident phagocytic cells and fibroblasts.
14.5 Ink Particle Properties
With the recent introduction of an entire spectrum of tattoo ink colors, the market for multicolored tattoos has exploded. The treating physician is now often presented with a multitude of synthetic compounds whose properties strongly influence the response to laser-tattoo removal but are otherwise completely unknown. In preparation for tattoo removal, it has become important for the laser surgeon to be cognizant of specific ink particle attributes such as color, size, chemical nature, and laser wavelength absorption peaks, among others.
14.5.1 Color
Tattoo ink color compounds have undergone considerable evolution since the 1980s, with the gradual incorporation of numerous additional colorant groups. Tattoo ink particles can be divided into pigments and dyes. By definition, a pigment is insoluble and unaffected by the medium in which it is dispersed, resulting in a long-lasting and unchanging color pattern. In contrast, dyes represent water-soluble colorants that tend to chemically react and change with time, resulting in fading attributes. Due to these intrinsic features, the majority of tattoo inks incorporate pigments, whereas cosmetic tattoos, also known as permanent makeup (PMU), usually consist of organic dyes.4 The newest types of tattoo inks however, such as the erasable and invisible-fluorescent varieties, also contain organic dyes complexed to beads of polymethylmethacrylate (PMMA) that facilitate the laser-removal process.5
Tattoo pigments can be further subdivided into organic and inorganic compounds. Inorganic pigments, such as metallic oxides, sulfides, and minerals, were widely utilized in tattooing from an early stage. Agents such as cobalt (blue), cadmium sulfide (yellow), mercury sulfide (red), and lead carbonate (white) were commonly employed until their discontinuation due to the occurrence of significant allergic reactions or concerns regarding exposure to toxic heavy metal levels. Metallic pigments in current use today include iron oxide (red, brown), chromium oxide (green), manganese (purple), and titanium dioxide (white). Titanium dioxide and iron oxide deserve special mention because these two pigments are still widely in use and can undergo laser-induced, oxidation-reduction reactions that result in tattoo darkening and a greater likelihood of treatment failure.6
Organic tattoo pigments constitute 80% of all modern tattoo ink mixtures and can be chemically categorized into azo and nonazo (polycyclic) compounds. Azo compounds are often seen in yellow, orange, red, and brown ink mixtures. Azo tattoo pigments have recently received increased scrutiny because some of these compounds have been shown to yield potentially carcinogenic aromatic amines when exposed to the action of quality-switched (Q-switched, QS) lasers.7 Nonazo compounds, such as dioxazine and phthalocyanine are commonly present in modern violet, blue, and green tattoo inks.
Pigment colors can significantly impact a tattoo’s susceptibility to laser removal because they influence laser–particle interactions that strongly depend upon the pigment’s intrinsic light-absorption properties. ▶ Fig. 14.3 displays the absorption spectra for several common tattoo colors. Ink pigments, upon being introduced into the dermis, act as external chromophores, and naturally compete with dermal melanin, hemoglobin, and water for absorption of radiant laser energy. The color of a pigment represents the wavelength-specific light reflected off that compound; as such, a red pigment naturally reflects red light and absorbs all other visible wavelengths. In order to maximize laser–chromophore interactions, and therefore pigment clearance, it is often beneficial to utilize a laser wavelength that is closest to the chromophore maximum absorption peaks. For example, the frequency-doubled, QS 532-nm neodymium:yttrium aluminum garnet (Nd:YAG) laser delivers a wavelength that is closest to the maximum absorption peaks for red and orange pigments and has become the preferred laser for removal of those tattoo colors.8 Black and other dark pigments tend to absorb most wavelengths and therefore respond to most currently available QS lasers. Several additional ink particle and laser properties should be considered when selectively targeting tattoo pigments, such as particle size and thermal relaxation times, as well as particle dermal location and laser depth of penetration, which are discussed next.
Fig. 14.3 (a–c) Wavelength-dependent absorption of several commonly utilized tattoo pigment colors. Arrowheads indicate the wavelength of common Q-switched (QS) lasers utilized in tattoo removal. P.Y., yellow; P.R., red; P.V., violet; P.G., Green; and P.B., blue. (Adapted with permission from Bäumler W, Eibler ET, Hohenleutner U, Sens B, Sauer J, Landthaler M. Q-switch laser and tattoo pigments: first results of the chemical and photophysical analysis of 41 compounds. Lasers Surg Med 2000; 26(1): 19.)
14.5.2 Size
The size of a pigment particle has significant consequences on a laser’s ability to selectively target the ink while avoiding possible injury to surrounding dermal structures. The length of time that a particle requires to dissipate the heat incurred from laser exposure is termed its thermal relaxation time (TRT), and is directly proportional to the square size of the chromophore. As a result, the smaller the size of a target particle, the shorter is its TRT. The relationship between the TRT and the laser pulse duration can determine the likelihood of collateral thermal injury to surrounding tissues. If the laser pulse duration is greater than the pigment particle’s TRT, then thermal damage will be incurred by the particle’s immediate surroundings. In contrast, if the pulse duration is shorter than the target particle’s TRT, then injury will be avoided.
The thermal relaxation times for common skin structures are listed in ▶ Table 14.1. Because of the size differential between tattoo pigment particles and surrounding dermal structures, adjusting the laser beam’s duration to less than the target particle’s TRT may help avoid unnecessary collateral skin damage. A frequency-doubled, 532-nm Nd:YAG laser with a pulse duration of 5 nanoseconds (ns) allows for the treatment of a red-colored tattoo without inducing substantial thermal damage to capillaries. The average tattoo pigment particle sizes are diagrammed in ▶ Fig. 14.4, with the majority of compounds existing between 2 to 400 nm. Unfortunately, dermal melanosome sizes significantly overlap with those of tattoo particles, and therefore both have similar thermal relaxation times. To prevent collateral injury to melanocytes, and subsequent dermal dyspigmentation, higher wavelength lasers that minimize melanin competitive absorption, such as the 1,064-nm Nd:YAG, are commonly employed in the treatment of dark blue or black tattoos.9
Structure | Size (μm) | Thermal relaxation time |
Tattoo ink particle | 0.5–4 | 10 ns |
Melanosome | 0.5–1 | 1 μs |
Erythrocyte | 7 | 2 μs |
Blood vessel | 50 | 1 ms |
Blood vessel | 100 | 5 ms |
Blood vessel | 200 | 20 ms |
Hair follicle | 200 | 10–100 ms |
Data from Bogdan Allemann I, Kaufman J. Laser principles. Curr Probl Dermatol 2011; 42: 7–23.) |
Fig. 14.4 Particle-size profiles obtained by laser diffraction for selected tattoo pigment colors. (Adapted with permission from Høgsberg T, Loeschner K, Löf D, Serup J. Tattoo inks in general usage contain nanoparticles. Br J Dermatol 2011; 165(6): 1215.)
14.6 Tattoo Removal
As the popularity and prevalence of tattoos in the United States have grown, so has the interest in inexpensive and scarless tattoo-removal services. Approximately 24% of the adult American population is estimated to have at least one tattoo, and, when surveyed, 17% of these are actively considering pursuing tattoo removal, with 6% undergoing removal every year.10 Whereas the motivations for tattoo acquisition often invoke a need for individuality and personal expression, individuals usually seek tattoo removal due to loss of interest or the frequent need for tattoo concealment resulting from previous negative comments, embarrassment, or changes in career.11 As newer, more rapid techniques of laser-tattoo removal have emerged and the adverse effects and complications have decreased with the development of nonablative modalities, the interest in laser-tattoo removal has seen a very rapid, recent growth. The American Society for Aesthetic Plastic Surgery (ASAPS) estimates that the number of laser tattoo-removal procedures increased by 43% between 2011 and 2012 alone.
14.7 History of Tattoo Removal
A variety of tattoo-removal techniques have been available for as long as tattooing has existed. The oldest method, salabrasion, described by Aetius around 543 AD, induced dermal injury via the application of coarse salt granules to the tattooed skin. Other techniques, such as dermabrasion and caustic (tannic acid and silver nitrate) or thermal (fire, hot coals) means of tissue destruction quickly fell out of favor due to their unacceptably high rates of scarring and dyspigmentation.
The introduction of ablative lasers for dermal resurfacing heralded a new era for tattoo removal and incited a growing interest in the underlying basis of ink particle–laser interactions. In the late 1970s, ablative modalities such as the 10,600-nm carbon dioxide (CO2) laser resulted in pigment clearance but, due to its indiscriminate targeting of water molecules in the skin, produced dermal fibrosis and scar-tissue formation. Subsequent attempts to selectively target ink particles with the 488/514-nm argon laser also met with challenges, such as significant dermal scarring, due to excessively long pulse durations (50–200 ms) and resultant nonselective tissue destruction.
In 1983, the principle of selective photothermolysis was postulated by Anderson and Parrish. The theory stated that if a particle is exposed to a well-absorbed laser wavelength, thermal tissue injury will be minimized as long as the laser exposure time (pulse duration) is shorter than the particle’s thermal relaxation time.12 This new concept revolutionized the approach to laser-tattoo removal and sparked the search for, and development of, ultra short-pulsed lasers, leading to the introduction of (Q-switched, QS) into the field of laser dermatology. QS involves the implementation of an electro-optical switch that enables the rapid release of extremely high-energy laser emissions. Modern QS lasers can deliver high-peak power pulses in the range of several nanoseconds (10–9 ns) to picoseconds (10–12 ps).
14.8 Laser Tattoo Removal
14.8.1 Laser–Pigment Interactions
The dissipation and clearance of tattoo pigment particles following laser exposure is due to several incompletely understood physical interactions. Tattoo-removing lasers have a photothermal effect upon pigment particles, in which the radiant energy of the laser is converted into heat, resulting in temperatures in excess of 1,000°C that subsequently produce target vaporization. In addition, the photoacoustic effect leads to a rapid, thermally induced ink-particle expansion that incites localized shock waves. This aspect of laser–particle interactions is most significant for tattoo removal because it is thought to result in the fragmentation of ink particles that renders them amenable to phagocytosis and immune clearance. Finally, a laser interaction can also yield a photochemical effect, in which permanent cleavage of molecular bonds within the ink pigment induces a shift in its optical properties, leading to a perceived lightening of the tattoo.13
14.8.2 Laser Parameters
Different laser parameters should be considered when determining the ideal laser settings in preparation for tattoo removal. Factors such as tattoo type, color, location, and Fitzpatrick skin type should influence the treating physician’s choice of laser wavelength, pulse duration, fluence, and spot size. These become important in minimizing adverse reactions and complications.
Wavelength
The wavelength of the chosen laser should ideally match the absorption spectrum of the target tattoo particle. In doing so, thermal injury to the surrounding tissues resulting from competitive absorption by melanin, hemoglobin, and water, is minimized. ▶ Fig. 14.5 shows the range of available lasers along the electromagnetic spectrum and absorption curves for the three primary skin chromophores. Each laser demonstrates intrinsic advantages when applied to different tattoo colors and Fitzpatrick skin types. ▶ Table 14.2 lists the different available QS lasers and their color-tattoo applications.14
Fig. 14.5 Absorption curves for the intrinsic dermal chromophores: melanin, hemoglobin, and water. Vertical lines mark the wavelengths of commonly used, quality-switched (QS), tattoo removal (532- , 694- , 755- , and 1,064-nm neodymium) and ablative fractional skin resurfacing (2,940-nm erbium and 10,600-nm carbon dioxide [CO2]) lasers.
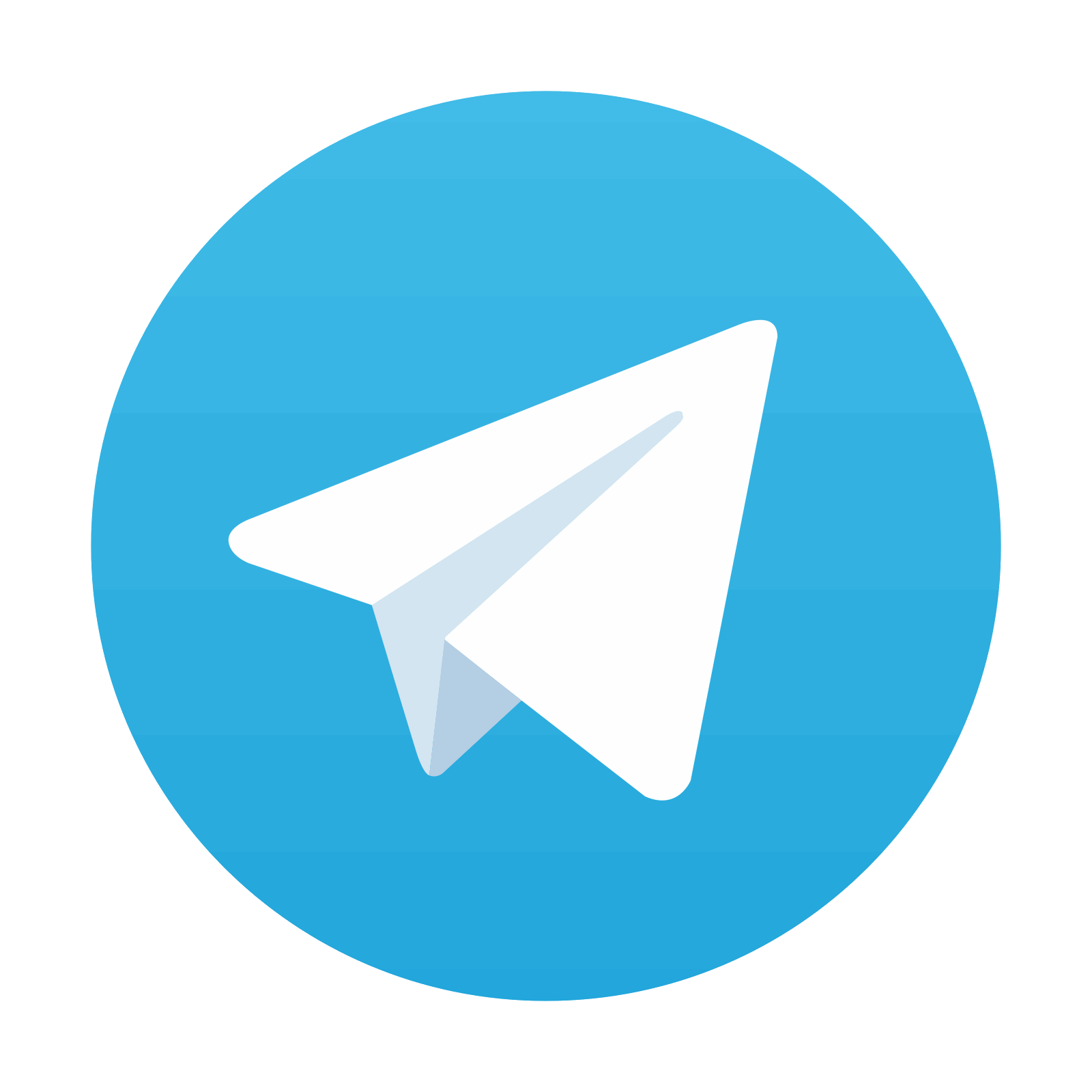