S. No
Enhancement method
Technology
Manufacturer
Drug
1
Iontophoresis
E-Trans®
Alza
Fentanyl
2
Iontophoresis
Iontophoretic drug delivery system
Iomed®
Lidocaine and epinephrine
3
Microporation
Microstructured transdermal system
3 M
Hydrophilic molecules, large molecules
4
Microporation
Macroflux®
ZosanoPharma, Inc.
Therapeutic protein
5
Needleless injector
PowderJect®
PowderJect
Pharmaceuticals
Insulin
6
Needleless injector
IntraJect®
Weston Medical
Vaccines
7
Microparticulate delivery
SMP (solvent microparticle system)
Atrix Labs
Dapsone
8
Thermal ablation
Heat-aided drug delivery system
Zars, Inc.
Lidocaine and tetracaine
9
Phonophoresis
SonoPrep®
Echo Therapeutics, Inc
Peptides, other large molecules
20.2 Skin Penetration Enhancement Techniques
An attempt was made to provide an overall overview of information about transdermal patents and to summarize the different major penetration-enhancement techniques used in cutaneous drug delivery systems (Table 20.2).
Table 20.2
Different penetration enhancement techniques
S. No. | Technique | Patent information | Invention/Description | Application/Claim |
---|---|---|---|---|
1 | Iontophoresis | US6119036 Allen Jr (2000) | Aqueous suspension or hydrophilic gel reservoir was replaced with silicone matrix | Problems with existing reservoir, like drug instability, electroendosmotic (EEO) flow, dryness of reservoir are solved, and thus the life span of the device is improved |
2 | Iontophoresis | US6248349 Suzuki et al. (2001) | Consists of peptides in a porous matrix | Reduces skin irritation |
3 | Microneedle | US7184826 Cormier et al. (2007) | Device consisting of a sheet having plurality of microblades has been unveiled for piercing and anchoring to the skin | Increased transdermal flux of polypeptide or protein and improved attachment of device to the skin with minimal to no skin irritation |
4 | Microneedle | US6230051 Cormier et al. (2001) | Consists of plurality of microblades for piercing the skin | Particular microblade geometry allows better penetration of the skin with less “push-down” (i.e., penetration and insertion) force required by the user |
5 | Microneedle | US 8,911,749 Ghartey-Tagoe et al (2014) | Microneedle array comprises a vaccine and a polymeric material | Insertion into the skin of a patient with an array of microprojections which comprises a vaccine for that disease |
6 | Microneedle | US20130245601A1 Pettis et al (2014) | The methods employ small gauge needles, especially microneedles, placed in the intradermal space to deliver the substance to the intradermal space as a bolus or by infusion | Delivery devices which place the needle outlet at an appropriate depth in the intradermal space and control the volume and rate of fluid delivery provide accurate delivery of the substance to the desired location without leakage |
7 | Microemulsion | US5688761 Owen et al. (1997) | Convertible or phase-reversible microemulsion, capable of being changed from w/o to o/w system by the addition of water to the former has been disclosed for delivering protein systemically | System was particularly useful for storing proteins for long periods of time at room temperature and above until they are ready for use |
8 | Ethosome | US5540934 | System composed of phospholipids (0.5–10 %), ethanol (20–50 %), propylene glycol (0–20 %), and at least 20 % water | Enhanced transdermal delivery of various pharmaceutical agents |
9 | Vesicle | US 8,865,208 Akatsuka et al. (2014) | Vesicle composed of glycerin fatty acid ester, a polyglycerin fatty acid ester, and a pyroglutamic acid glycerin fatty acid ester | Vesicle can be contained in an external preparation for use onto the skin |
10 | Transfersome | US6165500 Cevc (2000) | Transfersomes composed of phospholipids/glycerophospholipids and other lipids with added surfactants | Highly deformable vesicles which enhance insulin penetration, and are effective for prolonged drug delivery |
11 | Transfersomes | CN102949341 (A) Lin et al. (2013) | Tacrolimus transfersome prepared with phospholipid (2–10 %), penetration enhancer (3–8 %), surface-active agent (0.8–3 %), antioxidant (0.005–0.2 %), and water | Tacrolimus transfersomes have the advantages of relatively high drug-loading capacity, strong skin permeation, being capable of targeting deep skin |
12 | Transfersome | CN102846546 (A) Chen et al. (2013) | Two phospholipid materials with different phase-change temperatures, which are dipalmitoylphosphatidyl choline and soybean lecithin, are adopted as a composite phospholipid material | Phospholipid transfersome which promotes propranolol percutaneous absorption |
13 | Inclusion complex | US5817332 (A) Uritti et al. (1998) | Cyclized polysaccharide | Capable of improving the solubility of the therapeutic agent in the buffer solution by forming an inclusion complex with the therapeutic agent |
20.2.1 Microneedles
Microneedles (MN) technology for enhanced transdermal drug delivery was first reported by Henry et al (1998). Since then, there has been an increasing interest in this technology. Increased skin permeability by using the microneedle technology involves creating larger transport pathways (of micron dimensions) in the skin using arrays of microscopic needles. MN can penetrate the skin to create micron-sized pores that are big enough to permit the transport of macromolecules and even microparticles (Prausnitz 2004a). Safety studies performed with MN ranging in length between 500 and 1500 μm showed that the pain induced by the MN is significantly lower when compared to hypodermic needles (Gill and Prausnitz 2007). Most drug delivery studies have emphasized solid MN, which have been shown to increase skin permeability to a broad range of molecules. MN-mediated drug delivery has been extensively investigated for transdermal delivery of drug-loaded nanocarriers (Donnelly et al. 2010; Gomaa et al. 2014). MN technology has been disclosed in Patent No. 5879326 to Godshall and Anderson (1999) and Patent No. 5250023 to Lee and Shin (1993)). The use of micron-scale needles in increasing skin permeability has been proposed and shown to dramatically increase transdermal drug delivery, especially of macromolecules, such as insulin, proteins, oligonucleotides, and vaccines (Henry et al. 1998; Prausnitz et al. 2004a). Eppstein (2003) described a noninvasive method for increasing the permeability of the skin, mucosal membrane through thermal microporation. Another approach that has been investigated more recently is an integrated delivery system comprising a hypodermic needle and a transdermal patch (Coulman et al. 2006a). Transdermal delivery of insulin in vivo was studied using solid MN, and it was demonstrated that MN increased insulin delivery and thereby decreased blood glucose levels in diabetic hairless rats (Martanto et al. 2003). Patent of King and Walters (2003) claimed delivery of macromolecules into cells without employing a pressurized fluid injection step. The macromolecules in the electrode coating having a polynucleotide vaccine (deoxyribonucleic acid vaccine and/or ribonucleic acid vaccine) or a protein-based vaccine are administered using MN. Rosenberg (2003) provides a reliable way to deliver individual and multiple pharmaceutical agents in small doses by an intradermal device (MN). The multiple chambers of the delivery device enable the administration of multiple vaccines, adjuvants, and pharmaceutical agents simultaneously without prior reformulation or combination of pharmaceutical agents. A hollow microneedle of the conical shaped body with beveled, noncoring tip that is able to pierce tissue with broad base for transcutaneously conveying fluid was developed by Cho (2005). The base of microneedle was broad relative to height to minimize breakage during insertion. The microneedle device has few disadvantages, such as requiring a direct pushing motion against the skin and less efficient, compelling of drug fluid due to tiny openings. Gartstein and Sherman (2006) suggested a microstructured device (MN) for delivering the drug, which can provide efficient flow for some types of fluid compounds through the SC. The microstructured device could penetrate the outer skin layers by sliding or rubbing motions parallel to the skin surface, rather than perpendicular to the skin surface. Wilkinson and Hwang (2006) patented an invention entitled “valved intradermal delivery device and method of intradermally delivering a substance to a patient.” They explained in their patent about controlled flow of pharmaceutical agents, such as a drug or vaccine, through the device for the delivery into or below the SC to a depth sufficient for the pharmaceutical agent to be absorbed and utilized by the body. US Patent No. 5,457,041 of Ginaven and Facciotti (1995) reported MN made up of silicon, which carry a biologically active substance and are pierced into the target cells within the skin tissue. Thus, the biological substance is transferred from the tip portion and deposited within the target cells of the skin tissue. Gertsek et al. (2003) disclosed that MN have advantages of delivering a reconstituted pharmaceutical agent, where the dried substance is reconstituted by the dispensing diluent from the bladder within the device. MN with suction pump (vacuum generator) were developed to provide painless, precised insertion, controlled depth penetration, and variable, programmable delivery of active principles (Angel et al. 2006). A vacuum generator was attached to MN, which creates suction at the site of application to penetrate the surface of the skin (Angel et al. 2006; Angel and Hunter 2008). This modified device could be attached to the skin more effectively than prior devices and can be made with an extremely reproducible size and shape. Increased transdermal flux of polypeptides or proteins and improved attachment of the device to the skin with minimal to no skin irritation have been achieved by developing a device consisting of microblades (Cormier et al. 2007). These studies suggest that MN may provide a powerful new approach for transdermal drug delivery of macromolecules, especially for the delivery of proteins, DNA, and vaccines.
20.2.2 Sonophoresis
In 1990s, sonophoresis received a great attention from researchers for the delivery of macromolecules through the skin (Rizwan et al. 2009b). It uses low-frequency ultrasound (f <100 kHz) that enables the penetration of the drug. Commonly used frequencies for sonophoresis are generally separated into two: (i) low-frequency sonophoresis (LFS) (range of 20–100 kHz), and (ii) high-frequency sonophoresis (HFS), which includes frequencies in the range of 0.7–16 MHz (Mitragotri et al. 1995). The systemic absorption of drugs through the transdermal route depends upon the treatment duration, intensity, and frequency of ultrasound (Rizwan et al. 2009b). Mitragotri and associates reported in their two patents (US Patent No. 6,002,961 (1999) and U.S. Patent No. 6,018,678 (2000)) about applications of low-frequency (20 KHz) ultrasound for enhanced transdermal delivery of high-molecular weight proteins. Their findings indicated that permeation enhancement is dependent on the energy dose and length of ultrasound pulse which is consistent with a cavitation-based mechanism. Mitragotri et al. (1998) used low-frequency (20 KHz) ultrasound of intensity between 12.5 and 225 mW/cm2 for enhanced transdermal delivery of high-molecular weight proteins. The application of ultrasound significantly enhances permeation through human cadaver skin, that is, by 3.3, 8, and 9.8 times for insulin, gamma interferon, and erythropoietin, respectively. Therefore, it was possible to deliver an insulin dose of about 13 U/h from a patch having an area of 100 cm2 and providing an insulin flux of 100 mU/cm2/h. Weaver et al. (2012) disclosed in their patent that this method provides rapid, controlled, and higher drug transdermal fluxes, and allows drug delivery or analyte extraction at lower ultrasound intensities (f <100 kHz) than when ultrasound is applied in the absence of an electric field. Shimada and Shapland (1993) invented the use of multiple frequency sonophoresis for enhancing the diffusion of substance to or through the SC layer of the skin. This invention used two different frequencies, that is, 1 and 3 MHz. The method has many advantages such as decreased required time to administer substances and increase in blood perfusion at the treated area, which promote penetration of diffused substances across the skin membrane. Weimann (2007) reported a device consisting of a suspension of encapsulated drug as microparticulate system in place of drug solution. The radiation frequency was applied for a short period of time at a distance from the skin area, effective to generate cavitation bubbles. The penetration studies showed that microparticles of up to 25 μm penetrated into the skin under the conditions tested, whereas particles larger than about 40 μm did not penetrate.
20.2.3 Electroporation
This permeation enhancement technique involves the application of high-voltage pulse for a very short duration of time (<1 s). The high-voltage pulse forms aqueous pathway across the lipid bilayer of the skin (Prausnitz 1999; Rizwan et al. 2009b). The use of electroporation in transdermal delivery of macromolecules and physostigmine is documented in literature for organophosphate poisoning (Vanbever et al. 1997; Rowland and Chilcott 2000). US Patent No. 5,318,514 of Hofmann (1994) disclosed an apparatus including plurality of needle electrodes which can penetrate into the skin of the patient. To overcome the barrier, a novel, nonviral approach, involving the basic concept of electroporation to introduce genes and other therapeutic agents into the epidermis was provided by Zhang and Rabussay (2005). Rabussay (US US6266560 (B1)) (2001) disclosed a noninvasive method for treating erectile dysfunction using electroporation for enhanced delivery of a vasoactive or androgenic drug composition. It is applied to the penis which affords an alternative treatment for patients affected by erectile dysfunction.
20.2.4 Magnetophoresis
Magnetophoresis is a kind of a penetration-enhancement technique, in which flux enhancement is achieved by applying magnetic fields inducing mechanical forces to charged particles in such a way to force them into the skin (Giammarusti 2004). The skin barrier exposed to a magnetic field also might show structural alterations that could attribute to an increase in permeability (Murthy and Hiremath 2001). Ostrow et al. (2003) developed a system to provide an unencumbered self-powered transdermal patch for large-molecule drugs, which delivers the drug in an efficient manner directly to the affected area. The transdermal patch consists of a membrane composed of an inert biochemical substance. When an electric charge is passed through the membrane, it becomes electro-osmotic, which enhances the drug solution to pass through the skin surface to create an electroporative effect. The galvanic and electromagnetic current drivers can be utilized to propel permeant drugs through means of iontophoresis, magnetophoresis, or combined electromagnetophoresis. By this technique, the multiple patches could be configured as a linked patch unit for the user with a flexible cuff apparatus to provide circumferential treatment of a body part.
20.2.5 Thermal Ablation
In the transdermal thermal ablation technique, the skin surface is heated to vaporize tissue, which can locally remove the stratum corneum at the site(s) of heating. It is a noninvasive technique to remove small portions of the stratum corneum and thereby to increase skin permeability through micron-scale channels in the skin (Jeong et al. 2011). The major advantage of this technique is that the micropores are surrounded by healthy tissue, which facilitates skin recovery; typically, only 5–15 % of the skin surface is removed (Gold 2007; Trelles et al. 2009). Due to short exposure of the skin to ablation, the temperature gradient across the stratum corneum can be steep enough, so that the skin surface is extremely hot, while the viable epidermis and deeper skin tissues do not experience a significant temperature rise. Park et al. (2008) reported only a few-fold increase in skin permeability to a hydrophilic tracer compound after heating human cadaver skin to 150 °C, while after heating the skin to 300 °C, a 1000-fold increase in skin permeability was observed. A novel microfabricated device P.L.E.A.S.E.® (Precise Laser Epidermal System; Pantec Biosolutions, AG) is used to convert electrical energy into thermal and mechanical energy by ejecting a jet of superheated steam at the skin on a timescale on the order of 100 μs (Bachhav et al. 2011). The excitation and evaporation of stratum corneum lead to the formation of micropores, structures with a diameter of 100–150 μm by fractional ablation, which serve as transport conduits across the epidermis. The depth of each micropore is determined by the laser fluence (energy applied per unit area) used to create the pore and can be modulated to ensure either selective removal of the stratum corneum or to reach progressively further into the epidermis down to the dermal–epidermal junction. In a few seconds, this P.L.E.A.S.E.® device can create an array of several hundred micropores with a given depth, which lead to significant increase in molecular transport rates through the skin (Zech et al. 2011; Bachhav et al. 2010).
20.2.6 Photomechanical Waves
Photomechanical waves (PW) are pressure waves that can act by intense laser radiation, which lead to enhance skin permeation (Ogura et al. 2002). These waves act for a range of few nanoseconds to microseconds, while the amplitude is in hundreds of atmospheres (bar). The pressure waves interact with cells and tissues in ways that are different from those of ultrasound (Doukas and Kollias 2004). The application of PW for transdermal delivery has a number of advantages, such as the substantial penetration depth as well as the large size of molecules (proteins and genes) that can be delivered. These waves do not cause pain or discomfort and injury to the viable skin (Lee et al. 1999). They further reported that when a drug solution is placed on the skin and covered by a black polystyrene, and irradiated with a laser pulse, the photomechanical waves are responsible for stressing the horny layer and enhancing the permeation of drug solution. The drugs delivered via the epidermis can enter the vasculature and produce a systemic effect. PW can thus be applied for rapid delivery of human σ-aminolevulinic acid (ALA) allergen (Nethercott 1990) and insulin (Lee et al. 1998). Lee et al. (1998) have shown that 40 kDa dextran could be delivered 400 mm deep into the skin in vivo in an animal model using PW.
20.2.7 Inclusion Complex
The most extensively studied agents to form inclusion complexes are cyclodextrins (Pankaj and Samir 2009). The cyclodextrin drug complex has been used to enhance the solubility and permeability of a drug by encapsulation of an active drug molecule in its core. Inclusion complex contains a lipophilic core in which appropriately sized insoluble molecules can form noncovalent inclusion complexes which lead to increased aqueous solubility and chemical stability (Loftsson and Brewster 1996). Cyclodextrins have been reported to varyingly increase skin penetration, alone or in combination with other permeation enhancers (Legendre et al. 1995). Cyclodextrins can complex with enhancers like quaternary ammonium salts and reduce their toxic side effects on the skin, while still maintaining their skin permeabilization capacity, thereby showing a synergy between safety and potency (Martini et al. 1996). The skin penetration enhancement has also been attributed to extraction of stratum corneum lipids by cyclodextrins (Bentley et al. 1997).
20.2.8 Solid Lipid Nanoparticles (SLN)
Solid lipid nanoparticles (SLN) were developed at the beginning of the 1990s as an alternative carrier system to emulsions, liposomes, and polymeric nanoparticles (Muller et al. 2000). The research activities on SLN during the last decade focused almost exclusively on pharmaceutical applications. However, topical application of SLN was also studied, for both pharmaceutical and cosmetic products containing SLN. Major ingredients of SLN include solid lipids, emulsifiers, and water. The lipids include triglycerides, partial glycerides, fatty acids such as stearic acid, steroids like cholesterol, and waxes. SLNs have been used recently as carriers for transdermal drug administration. The encapsulated drug may penetrate across the skin and then expel drug from the SLN matrix as a consequence of polymorphic transitions occurring in the solid lipid (Shah et al. 2007). The active compound of cosmetic products must be retained in the skin, that is, it should penetrate sufficiently deep, but not too deep into the skin, which would lead to systemic availability (Muller et al. 2002). SLNs have been reported to deliver many macromolecules into the skin, including antibodies, hormones, RNA, and DNA (Chen et al. 2008; Westesen and Siekmann 2001). They exhibit high physical stability, potential of epidermal targeting, protection of incorporated labile actives against degradation, and excellent in vivo tolerability (Muller et al. 2000; Herzog. 2006). SLN composed of oil-soluble UV absorbers (1–40 %), solid lipids (20–98.9 %), emulsifiers (0.1–20 %), and liquid lipids (0–40 %) have been formulated, and skin protection efficiency was evaluated. The developed formulation showed improved effectiveness in skin protection by reduced absorption of UV absorbers (Herzog. 2006). The patent of Constantinides et al. (2006) describes SLN suspensions for mucosal and parenteral administration. However, the developed formulation could also be administered transdermally in the form of creams, ointments, etc. Muller and Olbrich (2004) described SLN in their US patent No. 6,770,299 entitled “Lipid matrix-drug conjugates particle for controlled release of active ingredient.” The SLN was prepared by high-pressure homogenization in order to obtain particles of submicron size. Westesen and Siekmann (2001) used the emulsifying process to prepare SLN and reported about nonspherical particles showing controlled release of poorly water-soluble drugs, primarily applied by i.v, and also by other administration routes including the dermal route.
20.2.9 Microemulsion
Microemulsions (ME) are lipid-based drug delivery systems, which have been used to enhance the transdermal permeation and bioavailability of poorly soluble drugs (Rizwan et al. 2009a). The permeation enhancement largely depends on the relative composition and concentration of the used oil, surfactant, and co-surfactant. Kreilgaard et al (2000) studied the ME system loaded with lipophilic (lidocaine) and hydrophilic (prilocaine hydrochloride) drug molecules and compared the drug flux to the flux obtained with conventional vehicles. The transdermal flux of both drugs was increased up to four and ten times, respectively, as compared to a conventional formulation. However, researchers also demonstrated the enhancing effect of different individual constituents, such as oil and surfactant present in ME. US Patent No. 6,426,078 (Bauer et al 2002) described oil-in water ME for lipophilic vitamins composed of diethylene glycol monoethyl ether or propylene glycol as co-emulsifier. The co-surfactant provided a considerable effect on the penetration of vitamins through the skin. The suitable combination of surfactants has also influenced the flux across the skin. The transdermal administration of oligonucleotides and nucleic acids offers promise of simpler, easier, and less injurious administration without need for sterile procedures and their concomitant expenses.
20.2.10 Dendrimers
The PAMAM dendrimers are widely used as transdermal carriers for drugs (Sonke 2009). These were proven to pass the cell membrane barrier, and therefore they were used as transdermal carriers to transport several water-insoluble drugs into the cell membrane (Venuganti and Perumal 2009; Borowska et al. 2010). They are three-dimensional nanosized (1–100 nm), highly branched monodispersed macromolecules, obtained by chemical reaction and producing a unique structure (Tomalia et al. 1985). They contain a dendritic core, which can accommodate hydrophilic and hydrophobic drug molecules, and they have been reported to release drugs in a controlled manner (Umesh et al. 2006). The PAMAM dendrimers themselves are biodegradable and nontoxic (Hans and Lowman 2002). Filipowicz and Wołowiec (2011) prepared riboflavin-loaded PAMAM dendrimers and concluded that the transdermal diffusion of dendrimer complexes, both through PVDF (polyvinylidenedifluoride) and PES (polyethersulfone) membranes was enhanced two to three times compared to control. The water-soluble PAMAM dendrimers can be successfully used in cosmetic and dermatological emulsions (Wollensak et al. 2003).
20.2.11 Vesicles
The most recent trend in dermal and transdermal drug delivery is the use of vesicle formulations as delivery systems. Many vesicular carriers have been reported in the literature which include liposomes (Mezei and Gulasekharam 1980), niosomes (Niemiec et al. 1995), Transfersomes® (Idea AG, Germany) (Cevc and Blume 1992), and invasomes (Dragicevic-Curic et al. 2008). These formulations have been reported to solubilize insoluble drugs by which the bioavailability of drugs increased. The walls of lipid vesicles are made up of amphiphilic molecules in a bilayer conformation. In an excess of water, these amphiphilic molecules can form unilamellar or multilamellar vesicles (Gregoriadis and Florence 1993). The internal aqueous compartment of vesicles can entrap hydrophilic drugs, whereas lipophilic and amphiphilic drugs can be attached to the vesicle by hydrophobic and/or electrostatic interactions (Martin and Lloyd 1992). They can improve drug deposition within the skin at the site of action where the goal is to reduce systemic absorption (to provide a localizing effect) and thus minimize side effects. They can provide targeted delivery to skin appendages in addition to their potential for transdermal delivery (El Maghraby et al. 2006). Naturally, all molecules pass through by a combination of all three routes (intercellular, transcellular, and appendageous), the relative importance of which will vary depending on the molecule’s physicochemical characteristics. Skin delivery of drugs by vesicles has gained attention after the commercialization of the dermal therapeutic system containing the antimycotic agent, econazole (Elsayed et al. 2007).
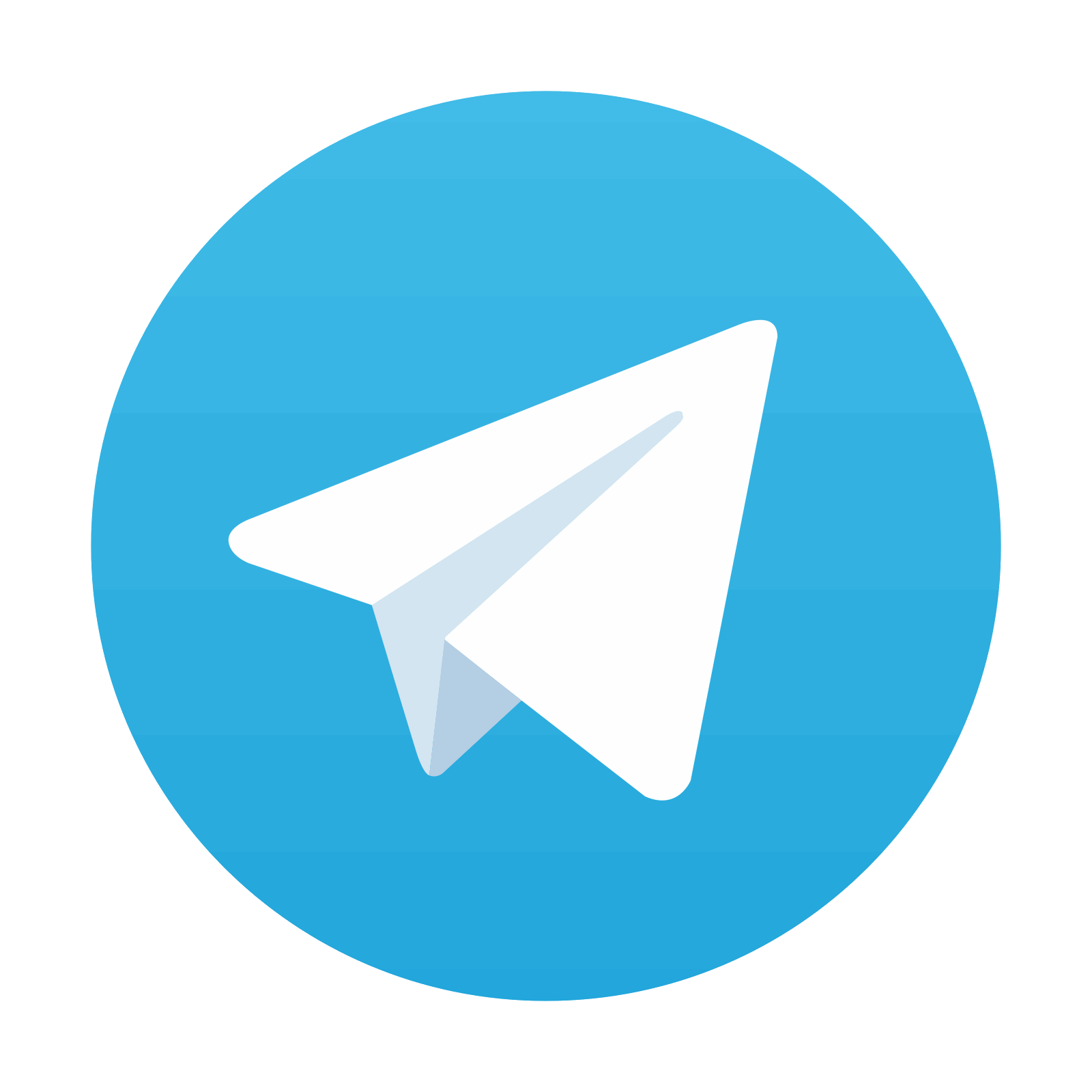
Stay updated, free articles. Join our Telegram channel

Full access? Get Clinical Tree
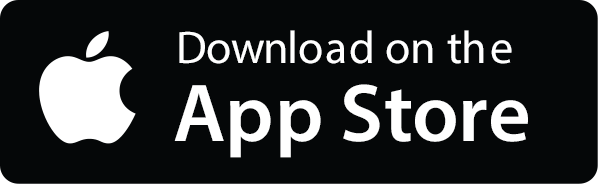
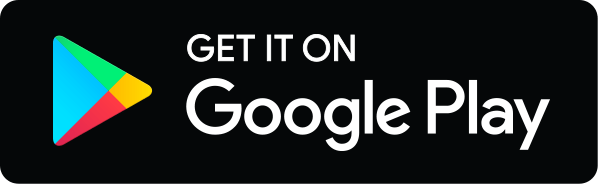