© Springer-Verlag Berlin Heidelberg 2017
Nina Dragicevic and Howard I. Maibach (eds.)Percutaneous Penetration Enhancers Drug Penetration Into/Through the Skin10.1007/978-3-662-53270-6_2222. Active Enhancement Methods in Transdermal Drug Delivery: Current Status and Future Perspectives
(1)
School of Pharmacy, Queens University Belfast, Medical Biology Centre, 97 Lisburn Road, Belfast, BT9 7BL, UK
Keywords
Transdermal drug deliveryIontophoresisSonophoresisElectroporationMicroporationMicroneedlesLaserRadiofrequencyJet injectionThermal22.1 Introduction
Transdermal drug delivery has many advantages, including:
Controlled delivery, achieving a steady-state profile, thus reducing the likelihood of peak-associated side effects and ensuring that drug levels are above the minimal therapeutic concentration
Reduced dosing frequency, with one transdermal patch delivering drug from typically 24–72 h
Avoidance of first-pass metabolism
Non-invasive means of drug delivery, putting the patient in control (dosage form can be easily removed in the event of an adverse reaction)
Less susceptible to bioavailability issues compared to the oral route
Provides an alternative route when the patient is unable to take drugs orally
However, the use of the route is severely limited by the restrictions imposed by the lipophilic stratum corneum barrier, such that only lipophilic drugs of relatively low molecular weight and reasonable potency (low dose) are suitable candidates for conventional transdermal delivery (Williams 2003). Modulation of formulation excipients and addition of chemical enhancers can increase drug flux, but often not sufficiently to ensure delivery of pharmacologically effective doses of drugs not possessing the specific physicochemical properties outlined above. Therefore, several active rate-controlled transdermal drug delivery technologies (electrical-based, structure-based, velocity-based, etc.) have been developed for the transdermal delivery of ‘difficult’ drugs. This is particularly so, given the high economic value of the transdermal delivery market, despite the relatively small number of actives (currently around 20) that can be delivered by this route. Broadly, facilitated delivery falls into two categories: technological, of which microneedles, electroporation, jet injectors, etc. are all good examples, and formulation approaches, most notably the focus on nanoscale delivery systems. The latter is discussed elsewhere in this book. Here, we will concentrate on the current state of play in active enhancement technologies used to enhance transdermal drug delivery, possible future developments and key milestones that must be achieved before patient benefit and commercial return on investment can be realised.
22.2 Active Enhancement Strategies
One of the most exciting developments currently ongoing in the transdermal drug delivery field is the use of active delivery devices. Such devices are minimally invasive in nature and can enhance the permeability of a biological membrane by targeting the shunt route (hair follicles and sweat glands) and creating transient aqueous transport pathways of micron dimensions across that membrane. In transdermal drug delivery, these devices force molecules across hair follicles and sweat glands or create micropore pathways in the skin’s stratum corneum barrier which, in turn, enables delivery of a wide range of drug molecules. Often hydrophilic small molecules, as well as macromolecules of biological origin (e.g. insulin, vaccines), are investigated for delivery using such strategies. Recently, such ‘active’ devices, namely, iontophoresis, laser, thermal ablation, electroporation, radiofrequency, ultrasound, high-pressure jets and microneedle technology, have all gained immense attention for their promising applications, both in the pharmaceutical and cosmeceutical industries. The ability of such strategies to achieve therapeutically useful plasma concentrations of hydrophilic drugs and macromolecules and enhance vaccine efficacy whilst reducing dose is beyond doubt. However, cost, safety and ease-of-use by patients remain concerns.
22.3 Moving Forwards
Given the ever-increasing evidence available within the academic and patent literature that a wide variety of active enhancement strategies are capable of achieving successful intradermal and transdermal delivery of conventional drugs, biopharmaceuticals, vaccines and active cosmeceutical ingredients, it is envisaged that the already-concerted industrial effort into development of such devices will now intensify. Furthermore, ever-more novel applications of active enhancement technologies are likely to come to the forefront. The ability of such devices to extract bodily fluids for drug and/or endogenous analyte monitoring is particularly interesting. For example, the use of microneedle-based devices to both deliver and extract molecules across the skin in a minimally invasive manner opens up the possibility for the development of a closed-loop responsive device, with a microneedle-based delivery component delivering a therapeutic molecule in response to information provided by a microneedle-based monitoring component. As technological advances continue, active enhancement devices may well become the pharmaceutical dosage forms and monitoring devices of the near future. However, there are a number of barriers that will first need to be addressed in order for the various technologies in this field to progress.
The ultimate commercial success of active enhancement delivery and monitoring devices will depend upon not only the ability of these devices to perform their intended function, but also their overall acceptability by both health care professionals (e.g. doctors, nurses and pharmacists) and patients. Accordingly, efforts to ascertain the views of these end-users will be essential moving forward. A study by the Birchall Group in this regard on microneedle-based drug delivery systems was highly informative (Birchall et al. 2011). The majority of health care professionals and members of the public recruited into this focus group-centred study were able to appreciate the potential advantage of using microneedles, including reduced pain, tissue damage, risk of transmitting infections and needle-stick injuries, feasibility for self-administration and use in children, needlephobes and/or diabetics. However, some concerns regarding effectiveness, means to confirm successful drug delivery (such as a visual dose indicator), delayed onset of action, cost of the delivery system, possible accidental use, misuse or abuse were also raised. Health care professionals were also concerned about interindividual variation in skin thickness, problems associated with injecting small volumes and risk of infection. Several other possible issues (accidental or errors based) and interesting doubts regarding microneedle use were discussed in this study. Overall, the group reported that 100 % of the public participants and 74 % of the health care professional participants were optimistic about the future of microneedle technology. Such studies, when appropriately planned to capture the necessary demographics, will undoubtedly aid industry in taking necessary action to address concerns and develop informative labelling and patient counselling strategies to ensure safe and effective use of microporation-based devices. Marketing strategies will, obviously, also be vitally important in achieving maximum market shares relative to existing and widely used conventional delivery systems.
Of the most commonly investigated active enhancement strategies, iontophoresis is the only one that does not create transient aqueous micropores in the skin, but rather uses an electrical potential difference to force charged, polar or neutral hydrophilic molecules of a wide range of sizes (up to about 12,000 daltons) across the low resistance shunt route in the stratum corneum, consisting of hair follicles and sweat glands. Iontophoresis induces a sensation of tingling or itching, depending on the density of the applied current. Besides these uncomfortable but harmless effects, skin irritation is the most common local adverse effect of cutaneous iontophoresis (Roustit et al. 2014). It occurs at both the anode and the cathode. Erythema is the most frequently described adverse effect, with a variable frequency according to the iontophoresis protocols. Skin irritation spontaneously and rapidly resolves, does not lead to permanent skin damage and does not disturb the barrier function of the skin. Although rare, burns have been observed, mainly due to operator error and the incorrect choice of electrodes/formulation composition. Indeed, the electrochemistry at bare metal or graphite electrodes involves electrolysis of water, which induces changes in the pH of the skin by generating H+ and OH− at the positive and negative electrodes, respectively. Variations in pH beyond the buffer capacities of the skin may lead to burns. High current density or prolonged application, as well as positioning of the electrodes over skin defects, increases the risk of burns. Burns are generally more serious under the cathode, due to involvement of OH− and rise in pH. Indeed, an alkaline phase erodes the epidermis and reduces skin resistance, making skin erosion worse. Therefore, an appropriate choice of buffer concentration in the formulation is needed to reduce the risk of burns (Roustit et al. 2014). A better solution is to use Ag–AgCl rather than bare metal or carbon-active electrodes, because they function at a lower potential and do not operate by water electrolysis. Several simple recommendations can decrease the risk of skin injury, such as avoiding pressure on the electrodes (not taping, binding or compressing either electrode) and ensuring that the electrode is uniformly wetted. Indeed, most commercially available electrodes are made of small sponges in contact with the skin. After dampening the sponge with the drug solution, it conducts the current. Therefore, heterogeneity in sponge dampening locally increases current density and may lead to skin injury. In the same way, the adhesive seal should adhere uniformly to the skin to avoid leaks. Moreover, cleansing the skin with alcohol and avoiding skin defects and contact between metal components and the skin are also recommended. Finally, current intensity should be <0.5 mA cm−2.
Material defects are actually the main cause of skin injuries, such as burns, usually resulting from direct contact between metal components and the skin. Another consequence of material defects can be overdose, which is potentially harmful when drugs are delivered for a systemic action and have a narrow therapeutic index. The most striking recent example was the iontophoretic delivery of fentanyl (Ionsys®). After receiving marketing authorisation for the European Union in 2006, corrosion of a component within the system was found in one batch. Although no case of fentanyl overdose was reported, this defect could have resulted in fentanyl release without activation by the patient. This could have exposed patients to fentanyl overdose, with a risk of severe respiratory depression. Ionsys® has not been marketed in Europe since October 2008, and the marketing authorisation holder did not apply for renewal of authorisation. Ionsys® has recently been acquired by another pharmaceutical company (Incline Therapeutics Inc., Redwood City CA, USA), currently developing new features into the system in order to obtain regulatory approval (Roustit et al. 2014).
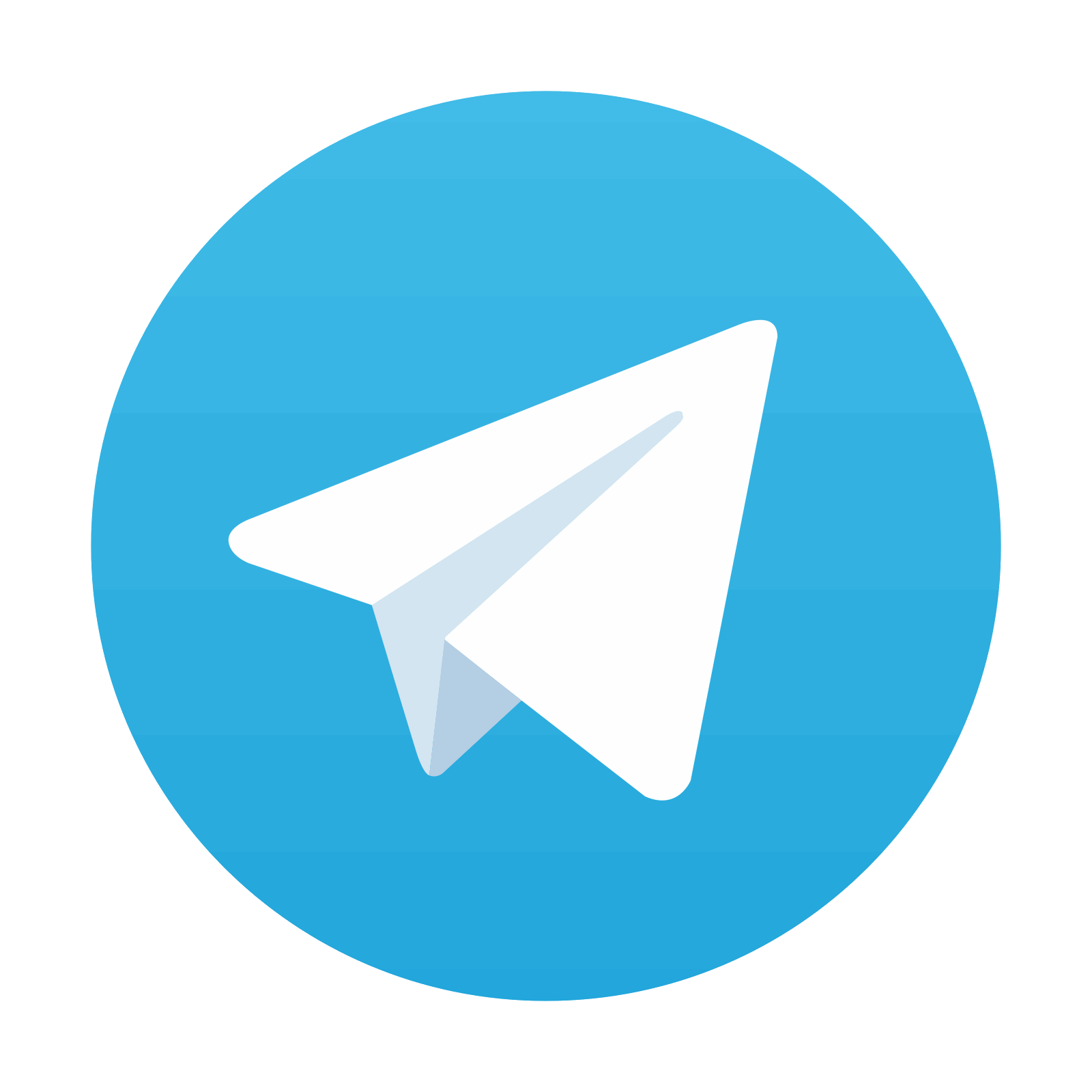
Stay updated, free articles. Join our Telegram channel

Full access? Get Clinical Tree
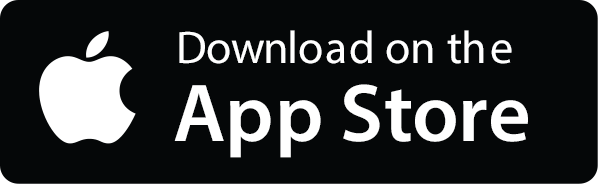
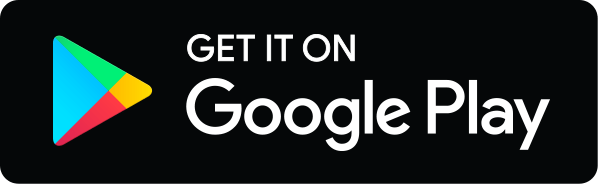