Introduction
Lymphedema can develop as a result of developmental or genetic abnormalities intrinsic to the lymphatic system (primary lymphedema) or secondary to extrinsic factors (infections, trauma, malignancy, obesity, etc.) that impair lymphatic function. Although the common denominator in both lymphedema subtypes is inadequate lymphatic function, the pathophysiology of the disease is complex, and the phenotype that is displayed clinically is highly variable.
Recent research has shed light on some of the molecular mechanisms that regulate disease progression and translate lymphatic abnormalities or injury to fibroadipose deposition. While this research is exciting and has provided some hope for therapies that may be effective in treating lymphedema, there is much that remains unknown. It is not clear, for example, why some patients have a mild, smoldering form of lymphedema while others develop a rapidly progressive, fulminant disease course. We do not understand why some areas of the limb are more prone to fibroadipose deposition and disease progression than others are. Similarly, it is unclear why a subset of patients develop collateral lymphatic vessels that bypass the zone of lymphatic injury, thus avoiding lymphedema development. Cellular and molecular studies with clinical biopsy specimens and preclinical models of lymphedema are therefore a critical and significant biomedical need and can help elucidate the mechanisms that regulate these pathologic changes. This understanding will improve not only our ability to prevent lymphedema development but also our ability to treat this disease medically and surgically. This chapter will serve as a review of the recent cellular and molecular studies that have shed light into the pathophysiology of lymphedema.
Pathophysiology of Primary Lymphedema
DNA sequencing studies have identified genetic abnormalities in patients with primary lymphedema. These genetic defects can be transmitted in families as a result of germline mutations or can develop from spontaneous somatic mutations. Genetic defects in patients with primary lymphedema have a broad range of effects: abnormal expression or function of growth factors that regulate lymphatic growth and repair, lymphatic valve abnormalities, leaky lymphatics, and changes in lymphatic smooth muscle cells. It is important to note, however, that the genetic defects that cause the development of lymphedema in most patients with primary lymphedema remain unknown. Nevertheless, the most common cause of primary lymphedema is deficits in the number and function of collecting lymphatics. These defects likely are present at birth and worsen over time, eventually resulting in overt lymphedema development.
The timing and severity of primary lymphedema are highly variable and may be modulated by the penetrance of genetic abnormalities, environmental factors, or hormonal fluctuations. For example, patients with subtle genetic lymphatic abnormalities may be asymptomatic due to the excess capacity of the lymphatic system to transport macromolecules. However, additional insults to the lymphatic system—for example, surgical injury or obesity—may lead to further declines in lymphatic function and overt development of lymphedema. In other patients with germline mutations of the lymphatic system, genetic abnormalities may have limited penetrance and involve only a subset of lymphatic channels. These patients may develop lymphedema later in life, as the pathophysiology of the disease advances to a point where the capacity of the lymphatic system to transport macromolecules is exceeded. Thus, the timing of lymphedema development in primary lymphedema is variable, with some patients exhibiting the disease shortly after birth— congenital lymphedema —or, more commonly, disease development later in life, termed lymphedema praecox or lymphedema tarda .
Congenital lymphedema, most commonly manifesting as lower extremity swelling in female patients, accounts for approximately 10–25% of all cases of primary lymphedema. These patients have variable severity of the disease, with some exhibiting mild swelling, while others display severe pathology. Further, primary lymphedema may present unilaterally or bilaterally, with differential severity between limbs. Milroy disease is a familial, sex-linked disease and is the classic form of congenital lymphedema, accounting for 2–3% of all patients with congenital primary lymphedema. Patients with Milroy disease have heterozygous inactivating mutations of vascular endothelial growth factor receptor 3 (VEGFR3) and usually present with leg swelling and chylothorax shortly after birth. VEGFR3 activation by its ligands—vascular endothelial growth factor C (VEGF-C) and VEGF-D—is a critical mechanism regulating lymphatic endothelial cell differentiation, proliferation, migration, and function. Homozygous mutations of VEGFR3 are embryologically lethal in mice; in contrast, heterozygous inactivating mutations allow for some lymphatic development. As a result, patients with Milroy disease have hypoplastic lymphatic vessels of the extremities, usually affecting the lower extremities more severely.
Lymphedema development before the age of 35 is referred to as lymphedema praecox . Patients with this form of lymphedema are most commonly female (ratio of females to males is 4:1) and usually develop unilateral lower extremity lymphedema around the time of puberty. The timing of disease development and its overlap with female hormone fluctuations have led some investigators to hypothesize that sex hormones may play a role in disease development/progression. However, the cellular mechanisms that regulate this response remain unknown, and only a few studies have investigated the role of female sex hormones in regulating lymphatic function. Pathologically, most patients with lymphedema praecox have fewer initial lymphatics and hypoplastic collecting vessels—although the severity of the disease is highly variable.
Patients who develop primary lymphedema after the age of 35 have lymphedema tarda . This presentation of primary lymphedema is infrequent and is a diagnosis of exclusion. Lymphedema tarda most commonly involves the lower extremity of women, and the genetic causes of the disease remain largely unknown. However, recent genetic studies have shown that some patients with lymphedema tarda have mutations in FOXC2 , a gene that regulates lymphatic valve development.
Most researchers concede that an arbitrary classification of primary lymphedema based on the age at which symptoms present is not particularly helpful when evaluating individual patients. This approach does not, for example, delineate the primary pathophysiology of the disease and is therefore not useful for developing targeted interventions. Instead, most patients are treated with palliative measures such as compression and physiotherapy, aiming to prevent disease progression. More recent studies have developed classification schemes that combine the clinical presentation of the disease with genetic sequencing. This approach categorizes congenital lymphedemas into five main groups: syndromic, systemic or visceral, disturbed growth, congenital onset, and late onset. This classification is more precise and provides more pathological information that may aid diagnostic or therapeutic interventions.
Pathophysiology of Secondary Lymphedema
Secondary lymphedema results from obstruction or injury to the lymphatic system; the most common worldwide cause of this form of lymphedema is an infection by roundworms ( Wuchereria bancrofti , Brugia malayi , and Brugia timori ) that cause obstruction of the lymphatic system—a disease commonly referred to as filariasis. It is estimated that as many as 200 million patients (or more) are afflicted with this form of secondary lymphedema. The parasites that cause filariasis are transmitted by mosquitos that deposit larvae in the tissues; the larvae then grow and migrate along lymphatic channels. Overgrowth of larvae, and the severe inflammatory response that follows, occludes the lymphatic system resulting in progressive and often disabling lymphedema. Treatment for this disease is limited to antiparasitic medications in the early stages of the disease that kill the developing larvae. Because the adult worm is resistant to these treatments, the disease process continues to advance in many patients, necessitating debulking procedures to remove lymphedematous tissues and maintain quality of life.
In Western countries, most patients who develop lymphedema do so as a result of iatrogenic injury to the lymphatic system during cancer treatment. Because solid tumors—breast cancer, melanoma, gynecological, or urological tumors—can spread along lymphatics and metastasize to regional lymph nodes, resection of these structures during cancer treatment is an essential aspect of local tumor control and cancer staging. Other cancers, such as sarcomas, do not metastasize to regional lymph nodes, and lymphadenectomy is usually not needed for surgical control or staging. However, even in these cases, lymphedema can develop due to severe lymphatic injury resulting from wide soft tissue resection and radiation therapy. Although the reported rates of lymphedema development are highly variable, it is estimated that 15–30% of all patients who undergo surgical treatment of solid tumors go on to develop lymphedema.
Most patients who undergo surgical management of solid tumors develop transient, mild postoperative limb or tissue swelling that resolves spontaneously in 2–6 weeks without any additional intervention. A subset of patients develop lymphedema 4–36 months after surgery (average is approximately 6–8 months). Nearly 80% of patients who develop lymphedema after axillary lymph node dissection for breast cancer do so within the first 3 years following surgery. However, the disease may present many years after the initial surgery, often after a minor infection or trauma to the affected extremity. Lymphedema tends to develop more rapidly in the lower extremities in patients treated for gynecological or pelvic tumors. In some cases, the transient swelling that occurs after surgery never resolves, and these patients often experience rapid progression of lymphedema. The cellular mechanisms that regulate these differences in progression times remain unknown; however, undoubtedly, pooling of interstitial fluid in the lower extremities due to gravity and positioning is a contributing factor.
Disease progression in patients with lymphedema is highly variable—some patients have a mild and slowly progressive disease that does not require significant intervention; others have a more fulminant course with rapid disease progression despite maximal medical intervention. Most patients with severe disease have additional risk factors for lymphedema (see later), most prominently radiation therapy and obesity. These risk factors can amplify the severity of the lymphatic injury and may exert additional effects on immune responses that regulate adipose deposition and fibrosis. For example, using mouse models, we have shown that radiation therapy significantly decreases the capacity of the lymphatic system to transport interstitial fluid and immune cells. These changes do not result in overt lymphedema; however, when combined with surgical injury, radiation therapy decreases the potential to form lymphatic collaterals and amplifies the lymphatic defects that are caused by lymphatic disruption. Similarly, we have found that obese mice tend to develop more severe lymphedema after lymphatic injury due to amplified inflammatory responses and decreased global lymphatic function.
Early diagnosis and aggressive physical therapy/compression are helpful in most patients who develop lymphedema and can help prevent disease progression and improve long-term outcomes. This approach is particularly important in the surgical management of lymphedema since treatments that decrease local tissue accumulation of interstitial fluid before surgery may promote the formation of collateral lymphatics by modulating inflammatory responses. These treatments may include both physical interventions such as compression stockings and complete decongestive therapy, as well as lifestyle changes such as weight loss and exercise as these measures may independently improve lymphatic function.
Cellular and Molecular Mechanisms of Secondary Lymphedema
The pathophysiology of lymphedema has historically been thought to be related to a failure of lymphatic collaterals to form after lymphatic injury. Indeed, anatomic studies in dogs by Suami and colleagues have shown that collateral lymphatics develop following axillary lymphadenectomy and that these channels bypass the surgical site to drain in the ipsilateral or contralateral neck/axilla. This hypothesis is also supported by genetic studies demonstrating abnormalities in gene expression or function of lymphangiogenic growth factors and their receptors. Not surprisingly, numerous preclinical studies have shown promising results in treating experimentally induced lymphedema by delivering growth factors—VEGF-C, hepatocyte growth factor, platelet-derived growth factor, among others—that promote lymphatic endothelial cell proliferation and differentiation. Other studies using porcine models of lymphadenectomy have shown that VEGF-C gene therapy increases lymphatic regeneration following lymph node transplantation. These findings have led to clinical trials combining VEGF-C gene therapy (Lymfactin, Herantis Pharma, Inc.) with vascularized lymph node transplantation in patients with breast cancer–related lymphedema. The results from this prospective, randomized, phase 2 clinical trial have not yet been reported.
However, clinical adoption of lymphangiogenic treatments for lymphedema treatment is controversial and has been hampered by the fact that more recent studies have failed to show improved lymphedema outcomes in other experimental models. The concept that lymphedema results from a deficiency in VEGF-C has also been called into question since the expression of this growth factor is paradoxically increased in the lymphedematous tissues and serum of patients with lymphedema. Clinical translation of VEGF-C gene therapy in cancer patients may also be complicated by the fact that this growth factor is also a key regulator of breast cancer growth and metastasis.
The lymphatic injury and collateral vessel formation hypothesis also fails to provide a rationale for the clinical characteristics of the disease. For example, lymphatic injury and collateral formation do not explain why lymphedema develops in a delayed fashion; collateral lymphatics usually form within weeks or months of surgery, yet most patients develop lymphedema months or even years after surgery. In addition, this model of lymphedema pathology does not provide a rationale for the finding that some patients develop lymphedema even after a seemingly minor surgery (e.g., sentinel lymph node biopsy or vein stripping). Perhaps the most intriguing aspect of lymphedema pathology that argues against a vascular defect model is the finding that changes in lymphedema involve the entire lymphatic vascular tree of the limb rather than the lymphatics at the site of injury. These clinical characteristics of secondary lymphedema suggest that lymphatic injury is an initiating event and that in some patients, additional pathologic pathways are activated that then mediate disease development and progression. Treatment of these additional pathological pathways may thus be a more rational approach than supraphysiologic doses of lymphangiogenic therapies.
The histology of lymphedematous tissues is characterized by the accumulation of fibroadipose tissues and chronic low-grade inflammatory responses. The fibrotic process involves the dermis and results in the deposition of thick collagen bundles around adipose tissues. In addition, in patients with late-stage disease, the lymphatic capillaries and collecting lymphatics become encased in thick collagen bundles. Progression of the disease is associated with sclerosis of collecting lymphatics, the proliferation of lymphatic smooth muscle cells, and eventual obliteration of the lymphatic vessel lumen. Although the cellular mechanisms that regulate lymphatic smooth muscle cell hypertrophy in lymphedema remain unknown, some investigators have hypothesized that this process results from increased afterload due to lymphatic obstruction secondary to lymphatic injury and fibrosis. Indeed, clinical studies using blood pressure cuffs and lymphoscintigraphy in patients before axillary lymphadenectomy have shown that increased lymphatic pumping pressure is associated with an increased risk of developing lymphedema postoperatively. This increased pressure was thought to reflect underlying lymphatic pathology that accentuated the adverse effects of surgery and may increase the propensity for development of lymphatic pump failure.
Progressive tissue fibrosis and lymphatic vessel obliteration suggest that lymphedema may be fibrotic organ failure of the lymphatic system. This hypothesis is supported by the fact that fibrosis is a common end-pathway for organ failure in all other organ systems; functional parenchyma in these conditions is gradually replaced by nonfunctional scar tissues. Fibrotic diseases and lymphedema also share clinical features: highly variable clinical presentation, chronic disease progression, and modulation by genetic or environmental factors. Many important risk factors for lymphedema—radiation, obesity, and recurrent infections—are also key regulators of fibrosis in a variety of other fibroproliferative diseases. These clinical features have led us to hypothesize that surgical injury to the lymphatic system initiates progressive fibrosis in a subset of patients and manifests as overt lymphedema once a critical threshold in diminished lymphatic function is reached. The risk of disease development is also modulated by factors that independently increase fibrosis.
Pioneering work by Stanley Rockson from Stanford University suggested that chronic inflammation in lymphedema plays a crucial role in the pathology of the disease. Using a mouse model of lymphedema, this group found that lymphedema results in the activation of inflammatory cascades. More importantly, they showed that inhibition of inflammation with ketoprofen, a nonsteroidal anti-inflammatory drug, decreased the severity of lymphedema after lymphatic injury. This report led to a clinical trial testing the efficacy ketoprofen for the treatment of 55 patients with primary or secondary lymphedema of the upper or lower extremity. The trial initially started as an open-label study (21 patients) and later was changed to a randomized, double-blind, placebo-controlled trial with 34 patients. Although treatment with ketoprofen failed to decrease excess limb volumes, biopsy specimens of the lymphedematous skin demonstrated decreased inflammation and improved skin histopathology. Subsequent studies from this group showed that the benefit of ketoprofen in lymphedema was derived from blockade of the leukotriene B4 pathway. A clinical trial with bestatin, a drug that preferentially blocks the leukotriene B4 pathway, was also recently completed, and although the results have yet to be published, early reports of the study indicated that patients treated with bestatin did not have significant improvements in limb volume. This finding is difficult to interpret but may reflect the heterogeneous population of patients who were studied (both primary and secondary lymphedema involving the upper or lower extremities). Alternatively, short-term drug may be insufficient to reverse years of pathological changes or significant fibroadipose tissue deposition.
Our approach to the treatment of lymphedema with anti-inflammatory medications has been more nuanced and based on an effort to identify inflammatory subtypes that are critical regulators of fibrosis in lymphedema. Using mouse models of lymphedema, as well as clinical biopsy specimens from patients with lymphedema, we found that lymphedematous tissues are infiltrated by large numbers of CD4 + T cells (T-helper cells). In addition, the degree of the T-helper cell infiltration positively correlates with the severity of lymphedema. Lack of T-helper cells or depletion of T-helper cells with neutralizing antibodies prevents the development of lymphatic vessel abnormalities and tissue fibrosis. In contrast, depletion of other immune cell types such as cytotoxic T cells, B cells, or macrophages has no effect on the pathophysiology of lymphedema in mouse models.
More recently, we have shown that T-helper cells in lymphedema undergo differentiation to the T-helper 2 (Th2) phenotype. These cells elaborate large amounts of profibrotic cytokines, including interleukin 4 (IL4), IL13, and transforming growth factor B1. Neutralization of these growth factors, like the ablation of T-helper cells in general, is also effective in treating or preventing lymphedema. This concept is important since immunotherapy—the use of neutralizing antibodies to neutralize cytokines or specific immune cell subtypes—is now commonly used for many chronic inflammatory disorders, including atopic dermatitis, Crohn disease, rheumatoid arthritis, psoriasis, among others. These treatments are highly targeted and, as a result, are well tolerated, lacking the side effects of general anti-inflammatory drugs such as corticosteroids. More importantly, Th2 inhibition, unlike VEGF-C injection, does not increase the risk of cancer metastasis or recurrence and can be used safely in cancer survivors. Thus, an immunotherapy approach may be a novel means of treating lymphedema and may be used as an adjunct to surgical interventions to improve outcomes.
In more recent studies, we have shown that application of tacrolimus—a drug that U.S. Food and Drug Administration approved for the treatment of atopic dermatitis by decreasing T-cell activity—to the skin is also highly effective in mouse models of lymphedema. Importantly, topical delivery of tacrolimus does not result in significant systemic drug absorption and avoids many of the potential side effects of this drug. Additional clinical studies are needed to test the efficacy of tacrolimus for the treatment of lymphedema.
Molecular Mechanisms of Risk Factors of Lymphedema
Many factors—obesity, radiation, infections, aging, and genetic factors—increase the risk of developing lymphedema after surgery. Obesity is a significant risk factor for lymphedema development. Obese patients with a body mass index (BMI) >30 have a two- to fivefold increase in the risk of developing lymphedema as compared with patients who have a healthy body weight (BMI <25). Even gaining weight after lymphadenectomy increases the risk of developing lymphedema twofold. Consistent with these observations are level 1 evidence derived from randomized controlled, prospective clinical trials demonstrating that weight loss or resistance exercise significantly decreases upper limb volume independent of additional interventions. These findings are supported by prospective longitudinal studies showing that the incidence of lymphedema is increased nearly twofold in patients with a sedentary lifestyle (without obesity), suggesting that exercise alone can modify lymphatic function.
Clinical and experimental studies have analyzed the mechanisms that regulate the effects of obesity and exercise on the lymphatic system. An interesting clinical study on healthy obese and normal-weight volunteers demonstrated that obesity significantly decreases macromolecular clearance from adipose tissues, suggesting that obese patients have impaired lymphatic clearance at baseline. These findings are supported by observational studies demonstrating that severely obese patients (BMI >59) can develop lower extremity lymphedema spontaneously even without surgery or other insults. Although this report was based on a small sample size, these patients exhibited abnormalities on lymphoscintigraphy—delayed uptake of tracer by draining lymph nodes and dermal backflow—that was identical to those noted in patients with primary or secondary lymphedema. These findings suggest that obesity progressively decreases lymphatic function; once the capacity of the lymphatic system to transport macromolecules is exhausted, overt lymphedema develops. This hypothesis is supported by the finding that animals with baseline lymphatic defects develop more severe forms of lymphedema after surgical lymphatic ablation. Other experimental studies have shown that weight gain resulting from high-fat Western diets is negatively and linearly correlated with lymphatic vessel number, lymphatic vessel pumping, and lymphatic vessel leakiness. These changes are mediated by a chronic inflammatory reaction induced by obesity in adipose tissues with resultant peri-lymphatic accumulation of macrophages and T cells. Increased production of nitric oxide resulting from upregulated expression of induced nitric oxide synthase by inflammatory cells is an important pathological event that increases lymphatic collecting vessel dilation and impairs pumping capacity. Interestingly, the inflammatory manifestations of obesity on the lymphatic system are partially reversible with exercise or weight loss in mouse models. However, clinical studies analyzing the lymphoscintigraphic findings of patients before and after weight loss surgery have suggested that some of the pathological effects of obesity on the lymphatic system are irreversible. Taken together, these findings suggest that weight loss and exercise programs may be beneficial in patients who may be candidates for lymphatic surgery since these behavioral modifications improve lymphatic function and regeneration. Additional research is needed to determine if the pathologic effects of obesity on the lymphatic system are reversible and how these effects are mediated on an intracellular level.
Radiation therapy increases the risk of developing lymphedema by two- to fivefold in patients who undergo extensive soft tissue resection or lymph node dissection. Radiation without surgery rarely causes lymphedema clinically. Experiments in preclinical models support this finding by demonstrating that although radiation decreases the capacity of the lymphatic system for transporting macromolecules, this decrease is not enough to cause overt lymphedema. The pathological effects of radiation on the lymphatic system are related primarily to fibrosis since the prevention of radiation therapy-induced fibrosis was highly effective in preserving lymphatic function in a mouse model. The adverse effects of radiation and obesity are additive because obese patients treated with radiation are more likely to develop lymphedema as compared to obese patients who were not treated with radiation therapy. A few studies have not shown a link between radiation and lymphedema development in breast cancer survivors; these inconsistencies are likely related to the fields (breast vs. regional lymph node drainage basins) used for radiation and heterogeneous patient population. However, large prospective studies have shown that radiation therapy delivered to regional lymph node basins significantly increases the risk of lymphedema development.
Many studies have linked postoperative infections and cellulitis with lymphedema development in patients who underwent lymph node dissection. These studies had suggested that infections injured the lymphatic system, further contributing to the impairments in lymphatic drainage capacity caused by the index surgery. This hypothesis is supported by recent studies demonstrating that bacterial toxins and staphylococcal infections permanently injure lymphatic endothelial cells and lymphatic smooth muscle cells. Other animal studies have shown that lymphatic injury results in the accumulation of T-regulatory cells, perhaps as a means of modulating the chronic inflammation that is associated with lymphedema (see later). T-regulatory cells play a key role in suppressing immune responses and are important modulators of autoimmunity. However, in lymphedema, this abnormal accumulation of T-regulatory cells results in impaired bacterial phagocytosis and processing by macrophages and decreases adaptive immune responses by T and B cells. These abnormal immune responses may therefore contribute to the increased risk of infections in patients with lymphedema. Thus innate and adaptive immune defects may predispose patients with lymphatic injury to develop infections that can then cause additional damage to the lymphatic system, thereby setting up a vicious cycle that leads to the rapid progression of the disease.
Some studies have suggested that older patients are at higher risk of developing lymphedema. For example, in a study of 287 Australian breast cancer survivors, patients who were more than 50 years old were 3.1 times more likely to develop lymphedema following axillary lymph node dissection as compared with those who were younger than 50 years old at the time of surgery. The importance of age as a risk factor for lymphedema is debated, however, as other studies have failed to show a significant correlation. Nevertheless, preclinical studies in mice have shown that aging decreases lymphatic collecting lymphatic vessel pumping, decreases lymphatic endothelial cell proliferation, and increases lymphatic permeability. These pathological changes may be related to mast cells and the production of histamines. It is therefore possible that aging may increase the risk of lymphedema; however, the cellular mechanisms that regulate this effect and the effects of physiologic vs. biologic age require additional study.
Genetic mutations are associated with primary lymphedema; thus, it is not surprising that some studies have shown that these mutations may also increase the risk of secondary lymphedema development, possibly by decreasing baseline lymphatic function. Finegold et al. reported that mutations in the connexin 47 or hepatocyte growth factor genes significantly increase the risk of breast cancer related lymphedema. Other studies have shown that some single nucleotide polymorphisms—normal variants of the same gene—significantly increase the risk of secondary lymphedema in patients who undergo axillary lymph node dissection for breast cancer treatment. A recent meta-analysis summarized the previous reports of genetic variations in patients with secondary lymphedema and suggested that coding variations in as many as 18 genes increase the risk of lymphedema development. This is important since decreases in costs of genetic testing may enable screening of patients preoperatively to identify high-risk patients, thus justifying additional medical or surgical interventions that may decrease the risk of lymphedema development. The critical point here is that lymphedema is a complex disease and that the risk of this disease can be modified by intrinsic (i.e., genetic, or patient-related) and extrinsic (i.e., surgical or treatment) risk factors. It is also clear that these independent variables interact with each other, further modulating the risk of disease development.
Conclusions
Secondary lymphedema is a common complication of cancer treatment and a significant source of morbidity in cancer survivors. Recent studies have helped elucidate the pathophysiology of this disease and are a vital step in the development of targeted treatments. These studies can also help improve outcomes following surgical treatment of lymphedema.
References
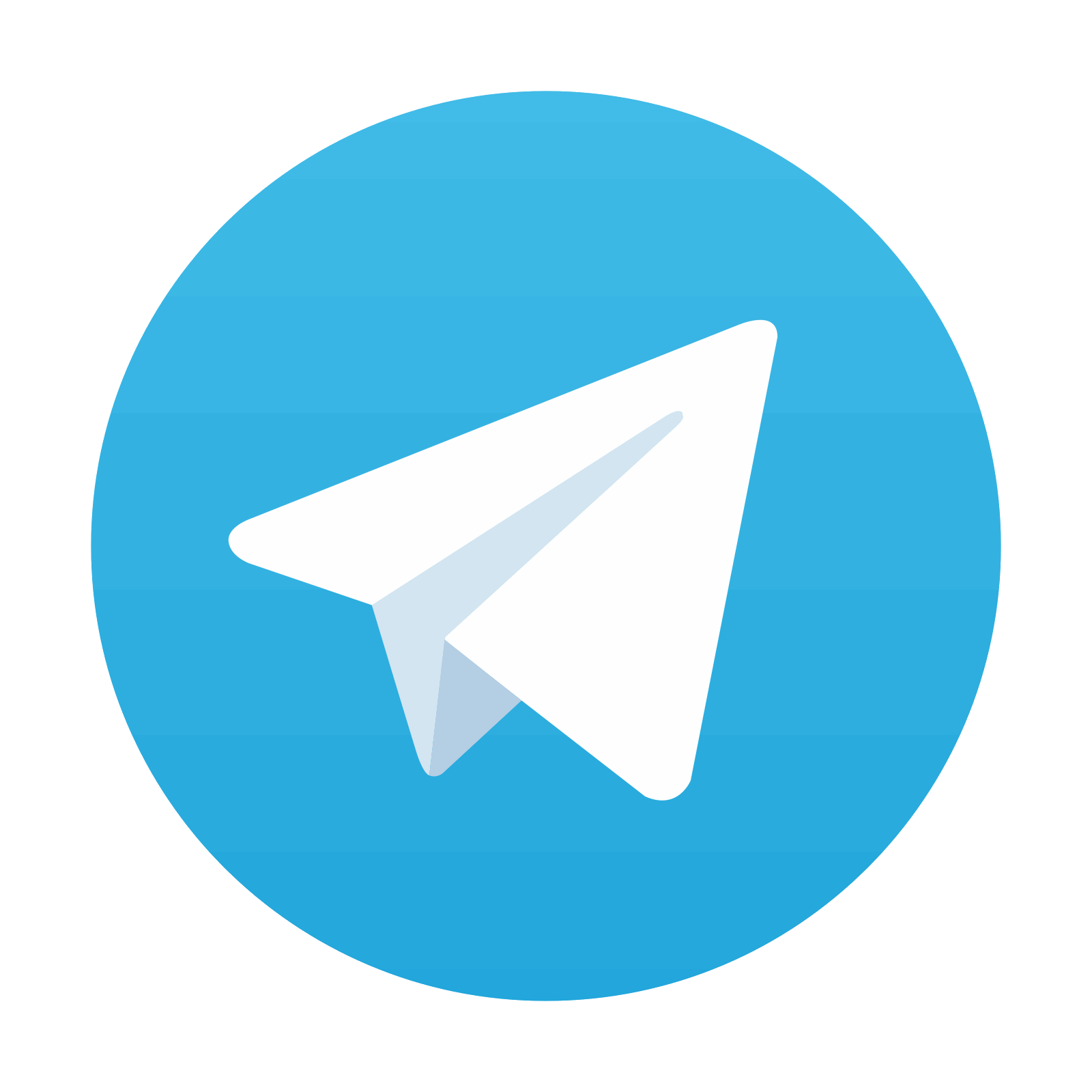
Stay updated, free articles. Join our Telegram channel

Full access? Get Clinical Tree
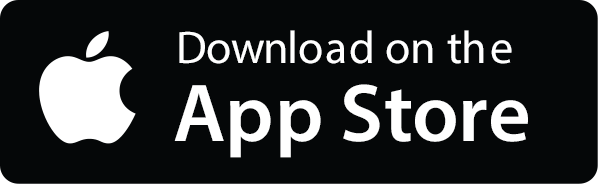
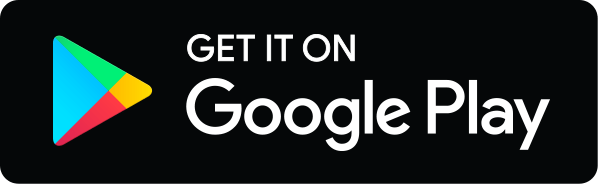
