- •
There is increasing demand for nonsurgical approaches to skin tightening on the body without downtime and with minimal risk. Lasers and light-based devices are being developed to meet this demand
- •
The ideal patient candidate is one with mild to moderate skin laxity and realistic expectations of outcome
- •
These devices are used extensively and safely on the face. Studies and experienced users have demonstrated their usefulness on the arms, legs, and abdomen
- •
Similar to other noninvasive devices, continual modifications to treatment protocols improve the clinical outcomes
- •
Skin-tightening treatments may be easily combined with other noninvasive body contouring devices aimed at fat reduction to achieve optimal results
Introduction
Patient concerns increasingly focus on the clinical signs of aging, including dyspigmentation, redness, wrinkles, and laxity of the skin. Increasingly, patients are dissatisfied with tissue redundancy and textual changes that appear on the abdomen, thighs, and upper arms. Traditionally, treatment for such concerns required surgical intervention and involved abdominoplasty, brachioplasty, and liposuction. More recently, technological advances have evolved in the direction of noninvasive body contouring and skin tightening. Although outcomes may not be as dramatic as surgical intervention, the favorable side effect profile and minimal downtime associated with noninvasive procedures have generated high demand from patients. Research and development within this realm has predominantly focused on facial and neck skin tightening, with less emphasis off the face.
Radiofrequency, ultrasound, lasers, and broadband light sources are among the devices most widely used. Clinical improvement of facial rhytides was initially noted when treating other aspects of photoaging with lasers. This finding led to histologic and clinical confirmation of neocollagenesis and rhytid reduction following treatment with lasers in the visible, near-infrared, and mid-infrared spectrum. Among the early lasers and light sources, the KTP laser (532 nm), PDL (585 nm and 595 nm), IPL (515–1200 nm), Nd:YAG (1064 nm Q-switched, 1064 nm long-pulsed, 1319 nm, and 1320 nm), diode (980 nm and 1450 nm), and Er:glass (1540 nm) were all found to have some efficacy for facial nonablative resurfacing. However, mid-infrared devices, due to the deeper penetration of their longer wavelengths and lesser absorption by epidermal melanin, were generally found to be the most effective for deeper rhytides and acne scarring after a series of treatments. Near-infrared broadband devices have yielded significant reduction of rhytides and skin laxity of the face and neck, with a histologic correlation of both neocollagenesis and neoelastogenesis.
Research using lasers and light-based technologies for rhytid reduction or laxity reduction on the body are limited. One important challenge for the investigator is differentiating between skin laxity and cellulite. The focus in this chapter is on the treatment of rhytides and skin laxity, termed ‘skin tightening’, on the body using lasers and light-based technologies.
Proposed mechanism of action
‘Skin laxity’ describes loose or redundant skin, with the appearance of prominent skin folds. On the body the most commonly affected areas are the upper arms, thighs, and abdomen. These visible changes are a result of the multifactorial process of skin aging, including both extrinsic and intrinsic factors. Intrinsic factors within the human dermis include loss of collagen, degeneration of the elastic fiber network, and loss of hydration. Extrinsic factors include ultraviolet exposure and the accumulation of elastotic material within the dermis (solar elastosis). Further, skin laxity resulting from mechanical stretching of skin from pregnancy or extreme weight, muscle, or body mass fluctuations may be cosmetically distressing. The goal of noninvasive tissue tightening is to reverse these changes by rebuilding the collagen and elastic scaffold of the dermis.
The mechanism of action of all noninvasive energy devices aimed at skin tightening is deep heating of the tissue. It has been documented that when collagen is heated to 65–70 °C irreversible denaturation of the triple helix occurs with subsequent recoil and contraction. It is then hypothesized that the long-term skin-tightening effects are a consequence of this thermal damage initiating long-term remodeling of the dermis, with new collagen deposition and fibroplasia. The way in which collagen is heated differs for each category of noninvasive skin-tightening device.
The infrared light-emitting devices target water as a chromophore and subsequently heat the surrounding collagen to elicit neocollagenesis and, in some cases, neoelastogenesis, associated with clinical improvement in rhytides and skin laxity.
Among laser and light devices, those in the infrared wavelength spectrum have been found to be the most effective in obtaining any degree of skin tightening. Devices in the mid-infrared spectrum are weakly absorbed by water within the dermis. Infrared devices within the wavelengths of 1000–1800 nm are able to penetrate deeper into the dermis than lasers in the visible light spectrum due to their longer wavelength and decreased absorption by melanin and hemoglobin ( Fig. 2.1 ). Longer wavelengths in the infrared spectrum, such as 2940 nm Er:YAG and 10,600 nm CO 2 lasers, are strongly absorbed by water which significantly limits their depth of penetration. Gentle volumetric heating of collagen from the energy absorbed by water may be achieved when laser/light is administered over longer pulse durations. Mechanisms to cool the epidermis are necessary when treating the dermis with infrared devices to prevent bulk heating related damage to the skin’s surface.
The use of epidermal cooling is essential to prevent bulk heating and injury to the epidermis.

Infrared devices
Clinical studies of infrared devices demonstrate immediate and delayed collagen changes post-procedure. Dermal collagen denaturation was seen on transmission electron microscopy from abdominoplasty skin samples immediately after irradiation with an infrared device (Titan, Cutera Inc., Brisbane, CA). Higher fluences correlated with deeper tissue injury, and the depth of maximal damage occurred at 1–2 mm. Although not fully understood, this immediate injury leads to subsequent wound remodeling over time, which is believed to create the skin-tightening effect that peaks over a 3–6-month period. In human skin treated using a 1450 nm flashlamp-excited Er: glass laser, biopsies obtained 2 months post-irradiation showed zones of dermal fibroplasia with increased numbers of fibroblasts observed at the depths corresponding to the initial thermal-induced changes. In an animal study, skin treated with an infrared laser device showed a significant increase in both type I and type III collagen at 45 days after irradiation. The type I collagen density remained significantly increased on histological examination 90 days after treatment, suggesting neocollagenesis. Furthermore, these investigators found similar findings in human skin. In both sun-protected and sun-exposed skin, there was a significant increase relative to controls in type III collagen and elastin persisting at the last data point taken 90 days post-procedure.
Four devices that are versions of the Titan system have been marketed to promote skin tightening on the body ( Table 2.1 ). The Titan device (Cutera Inc., Brisbane, CA) is an infrared light device emitting the spectrum from 1100 to 1800 nm. These wavelengths have a moderate level of water absorption and therefore can safely heat the dermis over a longer pulse duration when coupled with epidermal cooling mechanisms ( Fig. 2.2 ). The Titan XL (3 cm 2 spot size) has a maximum fluence of 50 J/cm 2 while the Titan S and V (1.5 cm 2 spot size) have a maximum fluence of 65 J/cm 2 ( Fig. 2.3 ). The pulse duration ranges up to 8.1 seconds. The handpiece is equipped with a sapphire window for pre-, parallel-, and post-cooling. A mobile technique has been developed which allows the use of 30% higher fluences, painlessly and safely, while maintaining efficacy in laxity improvement on the face and neck. The Titan is the only nonablative device shown to induce neocollagenesis and neoelastogenesis, which may explain its efficacy in treating both rhytides and laxity.
Device/manufacturer | Wavelengths | Handpiece size | Cooling |
---|---|---|---|
StarLux-IR, Lux-IR (Palomar) | 850–1350 nm | 12 × 28 mm | Sapphire contact cooling |
Titan (Cutera) | 1100–1800 nm | 15 × 10 mm and 30 × 10 mm | Sapphire contact cooling |
SkinTyte (Sciton) | 800–1400 nm | 1.5 × 4.5 cm | Sapphire contact cooling |
VelaShape (Syneron) | 700–2000 nm | 30 × 30 mm and 40 × 40 mm | None |


The second infrared lamp device (Lux-IR Fractional, Palomar Medical Inc., Burlington, MA) uses a near-infrared halogen lamp with a filtered spectrum of 850 to 1350 nm but with a unique fractionated handpiece. The handpiece with a spot size of 12 × 28 mm includes a skin surface cooling sapphire tip and a patterned optical window. The window contains an array of 21 apertures, each with a diameter of 3 mm, which facilitates delivery of the infrared energy within multiple spatially confined thermal lesions within the dermis. The device can deliver a total fluence of up to 200 J/cm 2 over a possible 10-second pulse duration.
Thirdly, the SkinTyte II filter may be inserted onto Sciton’s broadband light (BBL) device (Sciton, Palo Alto, CA) to emit a broadband infrared spectrum of 800–1400 nm. Fluence ranges from 0 to 60 J/cm 2 for the stationary technique and power ranges from 0 to 25 W/cm 2 for the mobile technique. (These techniques are elaborated further in a later section.) Cutoff filters of 590,695 or 800 nm may be employed with the mobile delivery method. Typical starting fluence for the stationary method is 40 J/cm 2 . Starting power setting for the mobile delivery is recommended at approximately 10 W/cm 2 . There is a sapphire tip to provide contact cooling.
Infrared light and diode lasers have been combined with bipolar radiofrequency to synergistically heat and therefore tighten the skin. This device, called the VelaShape ® (Syneron, Medical Ltd, Israel), has demonstrated tightening on both the arm and post-partum abdominal skin utilizing a combination of bipolar radiofrequency, infrared light, vacuum, and massage. The combination of light and radiofrequency is termed electro-optical synergy (ELOS) technology. The radiofrequency uses emission between 3 kHz and 1 MHz which generates heat by movement of electrons abiding by the principle of Ohm’s law. The use of the infrared light is able to first superficially heat the dermis as described previously, thereby lowering the impedance of the target tissue and allowing for potentially enhanced efficacy of the radiofrequency current. The vacuum device folds the epidermis and dermis between the two electrodes, allowing for less total energy to be employed and decreasing the risk of epidermal damage over unfolded skin.
A near-infrared device (NIR, Harmony, Alma Lasers) is in clinical trials (Alexiades-Armenakas, personal communication) for treatment of skin laxity of the thighs and abdomen. This near-infrared broadband light device has an output of 800–1600 nm, power output of 1–100 W, and a spot size of 18 cm 2 . Epidermal cooling is provided by a sapphire tip. Typical settings include 50 W for the delivery of approximately 30 kJ per 100 cm 2 treatment area using a mobile technique (Alexiades-Armenakas, personal communication).
Patient selection
The ideal candidate for noninvasive tightening procedures has mild to moderate laxity and has excluded surgical tightening procedures at the present time.
Patient selection is essential with these noninvasive devices. Expected results versus surgical outcomes should be reviewed and expectations managed prior to instituting treatment.
It is critical to discuss with patients that the degree of cosmetic enhancement with noninvasive procedures is typically modest at best and that some patients do not obtain appreciable improvement. Management of expectations is paramount for patient satisfaction, and it should be clarified that these devices are reserved for those patients who have ruled out plastic surgery. Pregnancy is a contraindication. Special attention should be paid to patients with implants or prior surgeries in the treatment area, an indwelling pacemaker or defibrillator, a history of keloids, diabetes, or vitiligo, those on a photosensitizing drug, or taking isotretinoin within the past 6 months. Treatments should not be performed if any rash is seen in the treatment area. Infrared devices are generally safe for darker pigmented skin types or tanned skin, though a test spot is generally recommended. It is of the utmost importance to take detailed pre-procedure standardized photographs.

Full access? Get Clinical Tree
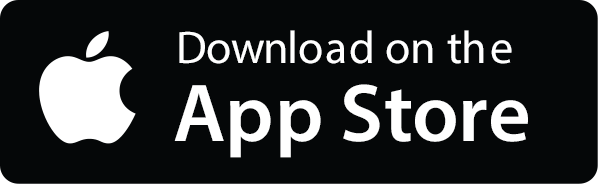
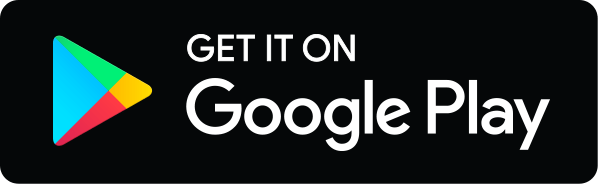