Nerve imaging is an important component in the assessment of patients presenting with suspected peripheral nerve pathology. Although magnetic resonance neurography and ultrasound are the most commonly utilized techniques, several promising new modalities are on the horizon. Nerve imaging is useful in localizing the nerve injury, determining the severity, providing prognostic information, helping establish the diagnosis, and helping guide surgical decision making. The focus of this article is imaging of damaged nerves, focusing on nerve injuries and entrapment neuropathies.
Key points
- •
The history and physical examination are the cornerstones of evaluation for patients presenting with peripheral nerve pathology. Imaging is an important adjunct, but not a replacement for a thorough and skillful history and physical examination.
- •
Magnetic resonance neurography (MRN) and ultrasound are the most commonly utilized modalities for evaluating peripheral nerves. Each has its advantages and disadvantages.
- •
Important parameters to assess on imaging studies of peripheral nerves include the caliber of the nerve, changes in signal intensity (whether magnetic resonance signal or echogenicity), course of the nerve, fascicular structure, continuity/discontinuity of the nerve, and the presence of mass lesions.
- •
Fat-saturated images and gadolinium contrast enhancement are valuable in gleaning information from magnetic resonance neurography studies.
- •
Emerging techniques for evaluating peripheral nerves include 7 T MRN, ultrasound elastography, and positron emission tomography using novel radiotracers.
Introduction
Nerve damage occurs in various ways. One commonly thinks about traumatic nerve injuries and nerve entrapments, but a variety of other conditions can damage nerves, including inflammatory and autoimmune conditions, primary nerve tumors, and perineural spread of malignancy. Regardless of the cause, damage to the nerve can cause weakness, numbness, paresthesias, neuropathic pain, and/or autonomic dysfunction. The mainstays in diagnosis are the history and physical examination. As an extension of the physical examination, electrodiagnostics can be helpful in localizing the injury, characterizing the injury, and in some cases, suggesting the pathology responsible for the injury. Although never a replacement for the history and physical examination, imaging also is increasingly playing an important role in the evaluation of damaged nerves. Again, imaging studies can not only help localize the nerve injury but can also play an important role in diagnosing the pathology responsible for nerve injury.
Improved imaging of damaged nerves has the power to transform clinical care and the way clinicians approach nerve injuries. For example, after closed traumatic nerve injuries, the typical approach is to wait 3 to 6 months to see if there will be evidence of spontaneous recovery before deciding whether surgery is appropriate. Imagine how this would change if one could image the damaged nerve and predict which nerves will go on to spontaneous recovery and which will not. As imaging improves, this may become a reality. Although new techniques are emerging, old techniques and the improvements that have already occurred are still an important part of the evaluation of nerve injuries and nerve pain and are already reshaping the way one thinks about nerve pathology. As an example, the literature would suggest that complex regional pain syndrome (CRPS) type 1 is more common than CRPS type 2. However, the authors have found at their institution that using a combination of a multidisciplinary approach and magnetic resonance neurography (MRN), many times a nerve injury can be identified in patients diagnosed with CRPS type 1, converting the diagnosis to CRPS type 2 and changing the approach to care. The authors observed that CRPS type 2 is more common than type 1 using this approach (Johnson, unpublished data, 2020).
The focus of this article is imaging of damaged nerves, focusing on nerve injuries and entrapment neuropathies. It discusses the currently available imaging techniques and some emerging techniques that may take a more prominent role in the future.
Magnetic resonance neurography
Introduction
With the advent of widespread MRI technology in the 1980s, the technique has been applied to imaging of a variety of specific anatomic structures. MRN is nothing more than MRI of peripheral nerves. This technique continues to evolve, allowing better depiction of peripheral nerves, their anatomic structure, and their pathologic states. Initial applications included anatomic imaging of the carpal tunnel, where the median nerve was identified as a structure with slightly higher signal intensity compared with surrounding tendons. One of the first demonstrations of peripheral nerve pathology via MRN was the finding that in carpal tunnel syndrome, the median nerve demonstrates T2-hyperintensity and an increased caliber. As MRN technology has developed, its indications have continued to expand.
Magnetic Resonance Neurography Technique
The mainstay of MRN is the identification of peripheral nerve anatomy and pathology using T1-weighted and T2-weighted fluid-sensitive (eg, short tau inversion recovery [STIR]) sequences, which are analyzed to understand nerve signal intensity, size and caliber, course, fascicular pattern, perineural tissues, and nerve-associated mass lesions. Imaging is generally performed on scanners with 1.5- or 3-T (T) magnets using phased-array surface coils shaped around the area of interest to increase signal-to-noise ratio and parallel imaging that allows for precise localization of the magnetic resonance signal. Normal nerves display an intermediate signal intensity similar to muscle on T1-weighted images and intermediate to slightly increased intensity on T2-weighted images, depending on the amount of background fat suppression and the presence of endoneurial fluid. Obtaining fat-saturated images is extremely important when trying to image nerves ( Fig. 1 ). Healthy peripheral nerves do not normally enhance with gadolinium contrast because of the presence of the blood-nerve barrier. Contrast administration may assist in cases where there is suspicion for infection, inflammation, diffuse lesions, or tumors, or in physical injury, where nearby denervated muscles may also demonstrate contrast enhancement. Thus, in the authors’ opinion, contrast should be given for all MRN studies, unless there is a specific contraindication. In abnormal nerves, the most common and easily identifiable change is an increase in the signal intensity of the nerve on T2-weighted images, approaching the intensity of adjacent blood vessels.

During the first 2 decades of MRN imaging, clinicians were limited to 2-dimensional sections in standard anatomic planes (ie, axial, coronal, and sagittal), which are adequate for anatomy that is aligned with the axis of an MRI magnetic field, such as an upper extremity lying flat against an examination table. Although advances in magnetic resonance sequences, such as T1-weighted spin echo (SE) and T2-weighted SPAIR (spectrally adiabatic inversion recovery) allowed for more homogeneous fat suppression, helping to show a nerve’s fascicular microstructure in greater detail. Anatomic structures obliquely situated within the body, such as the brachial plexus, were not easily depicted with even the most advanced 2-dimensional sequences. The development of 3-dimensional magnetic resonance sequences, such as curved multiplanar reconstructions (MPRs), allowed for the visualization of nervous structures with tortuous courses or oblique orientations. Newer sequences such as 3-dimensional STIR SPACE (Sampling Perfection with Application optimized Contrasts using different flip angle Evolutions) combine modern fat suppression techniques with MPR and maximal intensity projection (MIP) technology to optimize the visualization of the brachial plexus and other anatomically complex structures. Evaluating small, distal nerves situated next to blood vessels may be difficult. At their institution, the authors have found one of the most useful sequences to be the double-echo steady-state (DESS) sequence, which is sensitive to motion and helps differentiate blood vessels from nerves, particularly when attempting to image small nerves ( Fig. 2 ). For intradural applications, such as imaging nerve root avulsions, heavily T2-weighted myelography sequences, such as FIESTA, DRIVE, and CISS are preferred to compensate for cerebrospinal fluid flow. ,

Magnetic Resonance Neuropathy for Traumatic Nerve Injuries
MRN allows for the identification of nerve transection and helps distinguish injury patterns in nontransected nerves by visualizing details of nerve architecture. The resulting detailed characterization of nerve injury is important for management and prognostication. , Seddon’s classification system for nerve injuries defined 3 types of injuries: neurapraxia, axonotmesis, and neurotmesis, each associated with different pathologic features and clinical outcomes. Sunderland expanded the original Seddon classification based on the degree of injury to the axons and surrounding connective tissue. The increase in routine use of MRN has established findings suggestive of each of these levels of nerve injury. Although these findings can be suggestive, MRN cannot always reliably differentiate between different injury types or grades.
Neurapraxic, or Sunderland grade 1, injuries result in damage to the myelin sheath, with preservation of the axon and surrounding connective tissues. , The most characteristic sign on MRN is nerve enlargement and hyperintensity on T2-weighted and STIR images adjacent to the site of injury. This is most likely because of a combination of endoneurial fluid collection and vascular congestion, although the phenomenon is poorly understood. Mild muscular atrophy without gross signal change or denervation can also be seen in the muscles innervated by the affected nerves. ,
Axonotmesis was characterized by Seddon as damage that results in axonal disruption, typically with good recovery, as the epineurium and supporting structures are preserved. The Sunderland system further distinguishes 3 levels of axonotmesis, each with different clinical presentations that correlate with MRN findings. Sunderland grade 2 injuries have axonal disruption with preserved endoneurium allowing the potential for good recovery. On MRN, this is observed as hyperintensity and enlargement of the nerve, as in Sunderland grade 1, along with effacement and disruption of the fascicular architecture. Additionally, signs of muscle denervation and blurring of perivascular fat may be present. In Sunderland grade III injuries, the endoneurium is damaged, which leads to inappropriate routing of axons to targets, potentially as a result of fascicular scarring. Initially, MRN findings are similar to Sunderland grade 2, but when imaging is obtained subacutely or chronically, an area of hyperintense, fusiform enlargement, representing a neuroma-in-continuity, may be present. Sunderland grade 4 represents more severe nerve injury, including damage to the perineurium and poor prognosis for recovery without surgery. This consistently results in evidence of a neuroma-in-continuity on MRN, although not if imaging is obtained in the immediate postinjury period, as well as absence of fascicular appearance.
Sunderland grade 5 corresponds to Seddon’s neurotmesis, in which the entire nerve, including the epineurium, has been damaged. , To maximize diagnostic utility of MRN in suspected grade 5 injuries, imaging should be performed as soon as possible after injury, as the discontinuity fills with granulation tissue and fluid over time, leading to a swollen, thickened proximal ending of the nerve, which can diminish signal intensity and prevent diagnosis of a terminal neuroma.
Recently, a practical classification of nerve injuries based primarily on interpretation of MRN imaging that is more similar to Seddon’s original 3-tiered system has been proposed. Neurapraxic-type stretch injury is identified by increased nerve signal intensity and mild to no enlargement compared with nearby nerves. In the next tier of injury, nerve enlargement and fascicular effacement are seen, with or without a neuroma-in-continuity. Finally, the most severe injuries show evidence of complete discontinuity, with either frank transection or terminal neuroma on imaging. Particularly when evaluating neuropathic pain, the identification of a terminal neuroma, such as can occur with a transected nerve following an amputation, can be important in guiding treatment ( Fig. 3 ).

Changes visible on MRN in denervated muscle secondary to peripheral nerve injury may be important clues to the extent and chronicity of a peripheral nerve injury. In the subacute setting, denervated muscles can exhibit hyperintensity on T2-weighted imaging, particularly on STIR or similar sequences, often referred to as subacute denervation changes. More chronic denervation can result in atrophy with additional fatty infiltration of the muscle.
Magnetic Resonance Neuropathy for Entrapment Neuropathies
Entrapment neuropathies are defined as chronic, pressure-induced compression injuries. The injury is thought to occur secondary to increased endoneurial fluid pressure, resulting in microvascular congestion, infarction, and fibrosis. The criteria to diagnose a compression injury include close contact between a nerve and a compressive structure, the disappearance of the normal fat plane around an affected nerve, and a change in the nerve structure, including the fascicular architecture, nerve caliber, anatomic course, or signal intensity. In general, compressed nerves demonstrate high signal intensity on T2-weighted or STIR images, with denervation changes of surrounding muscle if the compression is advanced. The caliber of the nerve can sometimes be observed to be small at the site of compression, but more commonly, what is visualized is enlargement of the nerve immediately proximal to the site of compression ( Fig. 4 ). MRN imaging has been useful in cases of clinical ambiguity, where nerve function testing is indeterminate, or for postsurgical evaluation, as the hyperintense signal can normalize as soon as 8 weeks after neurolysis. ,

Pitfalls of Magnetic Resonance Neuropathy
MRN has several pitfalls that clinicians and radiologists must bear in mind. MRN is limited by technical challenges inherent to MRI technology, such as the magic angle phenomenon, which can cause an increase in T2 signal that may falsely be interpreted as an injury. Additionally, subclinical neuropathy may also present with increased T2 signal intensity within peripheral nerves, potentially misleading interpreters of MRN imaging to believe an injury exists, when, in fact, there is none. In the case of MRN imaging for remote peripheral nerve injuries, it can be difficult to distinguish regenerating from chronically degenerating nerves, which may pose a challenge to the diagnostician. Furthermore, the identification of a neuroma-in-continuity does not provide information about whether the degree of axonal regeneration across the site of injury will be sufficient to allow meaningful clinical recovery. Altogether, for its many advantages, the sensitivity of MRN at either 1.5 or 3.0 T is between 40% to 70%, suggesting that a normal or negative MRN study should not preclude further workup if clinical suspicion for a peripheral nerve injury is high.
Diffusion tensor imaging
Introduction and Techniques
Diffusion tensor imaging (DTI) is a magnetic resonance-based imaging modality that exploits the preferential diffusion of water in directions that align with the shapes of highly asymmetrical anatomic structures, such as peripheral nerves. DTI allows for both qualitative and quantitative analysis of the integrity of peripheral nerves. Tractography relies on this property of water to trace the anatomic course of a peripheral nerve, while 4 parameters that are traditionally calculated and visualized in DTI sequences allow for quantitative microstructural analysis of peripheral nerves. Fractional anisotropy (FA) reflects the degree of alignment within fiber tracts; axial diffusivity (AD) is thought to correspond with axonal integrity and changes rapidly in axonal degeneration. Radial diffusivity (RD) reflects the potential of water to diffuse perpendicular to the axis of a nerve and may indicate myelin integrity, and the apparent diffusion coefficient (ADC) is a mathematical representation of the mean diffusivity of tissue.
Applications for Peripheral Nerve Injury
DTI has been used to quantitatively measure peripheral nerve integrity immediately after injury and in a delayed fashion. It allows for analysis of microstructural damage to peripheral nerves in pathologic conditions, such as demyelination, axonal degeneration, and Schwann cell necrosis and has been used to demonstrate normal and pathologically altered fiber trajectory in 1.5 and 3 T applications. Changes in the diffusivity of water along the course of a damaged peripheral nerve are caused by blockage of axoplasmic flow, increased venous congestion, and distal Wallerian degeneration, all of which lead to widening of the space between axons and surrounding membranes, thought to be reflected by increases in ADC and corresponding decreases in FA.
Various pathologic entities have been imaged and described using DTI, including compressive neuropathies and nerve injuries. In carpal tunnel syndrome, the compressed median nerve exhibits decreased FA and increased ADC, which is at least partially mirrored by the decreased FA seen in the compressed ulnar nerve in cubital tunnel syndrome. , DTI also may be sensitive to transient, compression-induced neurapraxia. One group has shown decreased FA and decreased ADC of the median nerve along with increased FA and unchanged ADC of the radial nerve after brief tourniquet compression of the upper arm.
Nerve fiber integrity may be assessed with DTI tractography after injury and during recovery. Tractography was used to track regenerating nerve fibers through a median nerve transection after surgical repair in 1 report. One month postoperatively, nerve fibers were seen at the site of transection, followed by regeneration distal to the point of transection after 2 months, with electrodiagnostic evidence of recovery. Another group showed regenerating transected peroneal nerve fibers traveling through a sural nerve graft, forming a compact bundle of fibers through the graft 13 months after injury, again correlating with clinical and electrodiagnostic signs of recovery.
Pitfalls in Diffusion Tensor Imaging
DTI is limited by many of the same factors that make MRN challenging:
-
Thin, superficial nerves that are difficult to track using current techniques
-
Poor contrast resolution or volume averaging relative to nearby blood vessels, muscles, or edematous tissue
-
High susceptibility to ghosting artifacts caused by adjacent fluid flow and organ motion
-
Technical considerations related to imaging structures not in or near the isocenter of the magnetic field in the MRI scanner ,
Additionally, as quantitative analysis of nerve microstructure using DTI is relatively new, there is a dearth of consensus data regarding the normative ranges of diffusivity parameters, as well as significant inter-nerve differences, making assessment of pathology difficult. More confusing still are findings by some groups that FA may not significantly decrease in peripheral nerve injury, and ADC may actually decrease. , The early and as-of-yet immature state of peripheral nerve DTI is reflected by a recent meta-analysis demonstrating sensitivity of 83% and specificity of 78% for identifying peripheral nerve injury. More research and experience are needed to refine this technique before it can join ultrasound and MRN in the arsenal of routine clinical practice.
Neuromuscular ultrasound
Introduction
Neuromuscular ultrasound (NMUS) has seen a rapidly growing body of literature and widening applications in nerve and muscle disorders. The technique can be used to supplement the history, physical examination, and EMG/NCS, and in some instances, it can be used as a standalone tool. NMUS effectively evaluates nerve compression and trauma, identifies intraneural tumors and cysts, and screens for hereditary and inflammatory disorders. It is inexpensive and painless, and aids in real-time localization, diagnosis, and surgical decision making.
The relative accuracy of ultrasound and MRI for focal neuropathies varies. Excluding carpal tunnel and cubital tunnel syndromes, ultrasound has been found to be as specific and perhaps more sensitive for detection of focal neuropathies, particularly for identification of multifocal lesions such as autoimmune nerve disorders. , Thus, for sonographically accessible regions of the body, NMUS is often proposed as the initial imaging modality. ,
Technique
A linear transducer generating frequencies of 12 to 18 Hz is optimal, with higher frequencies providing the best images of cutaneous nerves. Piezoelectric elements within the transducer produce sound waves and capture reflected waves from echogenic body tissues. In nerve, the echogenic epineurium and perineurium highlight the outer boundaries of the nerve and fascicles. Individual axons are not visible. Ultrahigh frequency transducers up to 70 Hz have been explored for detailed images of individual fascicles, with potential utility for intraoperative evaluation.
Elements of interest when assessing a peripheral nerve include nerve size (measured as cross-sectional area and sometimes diameter), echogenicity, and fascicular enlargement. Increased vascularity may be seen on Doppler. Anomalous structures are noted and may include cysts, tumors, intruding muscle, penetrating arteries, and foreign bodies.
Neuromuscular Ultrasound for Entrapment Neuropathies
In general, an entrapped nerve will demonstrate an enlarged cross-sectional area (CSA) proximal and distal to its compressive site. Loss of fascicular detail and increased vascularity may be present. Entrapment may be caused by passage through a fibro-osseous tunnel; enlargement or malpositioning of nearby structures (such as muscle or bone); postoperative changes such as scarring, foreign bodies, and hardware; and various other causes. The nerve of interest must always be imaged at noncompressive sites for comparison, and various ratios exist for determining carpal tunnel syndrome and ulnar neuropathy at the elbow. If the nerve is diffusely enlarged at noncompressive sites, an inflammatory or hereditary neuropathy should be considered.
Carpal tunnel syndrome
In carpal tunnel syndrome, NMUS has proven consistently accurate when compared with nerve conduction studies (NCS) and clinical examination ( Fig. 5 ). In some cases with typical symptoms and normal NCS, ultrasound may be more sensitive. Although there are no formal guidelines for using NMUS in lieu of EMG/NCS, it may be considered in cases in which symptoms are classic and no other diagnoses requiring EMG/NCS (eg, radiculopathy, inflammatory neuropathy) are being considered. NMUS can also differentiate typical carpal tunnel syndrome from mimics, such as penetrating or thrombosed persistent median artery, cyst, or nerve tumor.

Full access? Get Clinical Tree
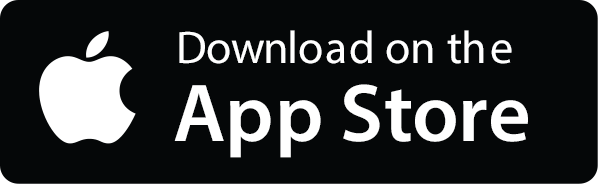
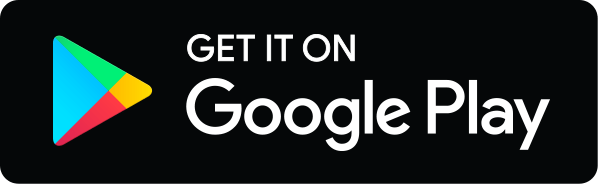