Neuroma formation occurs because of some degree of nerve injury followed by improper intrinsic nerve repair. The cause of neuroma pain is incompletely understood, but appears to be multifactorial in nature, including local and system changes. A comprehensive understanding of nerve anatomy, injury, and repair techniques should be used when dealing with neuroma formation and its physical manifestations. Diagnosis of neuroma is clinically characterized by pain associated with scar, altered sensation within the given nerve distribution, and a Tinel sign. The pathophysiology of neuroma formation is reviewed.
Key points
- •
Neuroma formation occurs because of some degree of nerve injury followed by improper intrinsic nerve repair.
- •
The cause of neuroma pain is incompletely understood, but appears to be multifactorial in nature, including local and system changes.
- •
The pathophysiology and genesis of neuroma formation are reviewed.
Introduction and history of neuroma
The peripheral nervous system is delicate and susceptible to injury. Nerve injury occurs from multiple mechanisms: pressure, stretch, chronic irritation, ischemia, crush, sharp transection, iatrogenic, and poor repair of prior injuries. Peripheral nerve injuries resulting in abnormal axonal regeneration and organization lead to neuroma formation. One of the earliest reports on neuromas came from Abroise Pare, in 1634, describing treatment of this entity with massage. In 1811, Odier provided the first gross description of a neuroma as a sensitive bulbous stump of a severed nerve. The first pathologic studies of neuromas followed closely, by Wood in 1828. Neuroma formation represents a challenging scenario for both the patient and the surgeon. Painful symptoms can be both physically and psychologically debilitating, leading to lifestyle modifications and decreased productivity. A multitude of research has been devoted to eliciting the mechanism of neuroma formation, preventative techniques, and treatments.
Nerve anatomy
To comprehend nerve injury and neuroma formation, a general knowledge of peripheral nerve anatomy is required. A basic review of the peripheral nerve and its cross-sectional anatomy is highlighted in this article. A more detailed description of the anatomy and physiology of the peripheral nerve, including microscopic and macroscopic structures, can be found in an article by Topp and Boyd.
Nerves are composed of neural, vascular, and connective tissues. Peripheral nerves arise from the central nervous system and may contain both motor and sensory nerve fibers. The cell body of the nerve resides in the brainstem, spinal cord, or dorsal/ventral root ganglia. Individual nerve fibers, known as axons, extend from the cell body to the periphery of the body. The axons may be unmyelinated or surrounded by Schwann cells and a myelin sheath. In cross-section, the axon represents the basic subunit of the nerve. The axon is surrounded by endoneurium, a type of connective tissue. Axons with the same end organ target are grouped together into fascicles, or bundles of axons. Fascicles are surrounded by connective tissue called perineurium. Groups of fascicles make up a peripheral nerve and are surrounded by an internal and external covering of epineurium. Vascular supply to the nerve courses within the nerve’s connective tissue and in a longitudinal fashion outside of the epineurium.
Nerve injury classification
Nerve injury can occur because of systemic and local pathologic conditions. Typically, local pathologic conditions, such as compression, stretch, blunt and sharp trauma, or iatrogenic injuries, are of greater interest to the surgeon. Injury to a nerve may vary in severity and involve one or more anatomic components: myelin, axon, or connective tissue. The 2 most commonly used nerve injury grading systems are the Seddon and Sunderland classifications.
In 1943, Seddon classified nerve injuries into 3 groups: neurapraxia, axonotmesis, and neurotmesis. Neurapraxia involves local myelin damage; however, the axon is preserved, and no distal degeneration occurs. Axonotmesis results as a loss of continuity of axons with varying degrees of connective tissue damage. Neurotmesis involves complete transection of the nerve with damage to axons, endoneurium, perineurium, and epineurium. Wallerian degeneration of the distal nerve occurs with both axonotmesis and neurotmesis.
In 1951, Sunderland further expanded this classification system to involve 5 degrees of neural injury. Sunderland’s first- and fifth-degree nerve injuries are equivalent to neurapraxia and neurotmesis, respectively. Second-, third-, and fourth-degree nerve injuries are variations of Seddon’s axonotmesis based off an inside-out injury pattern. Second-degree injury involves axonal injury with preservation of endoneurium, perineurium, and epineurium. Full recovery is expected from second-degree injury. Third-degree injury includes disruption of the axon and endoneurium, with partial recovery expected. In fourth-degree injury, the axon, endoneurium, and perineurium all incur damage, but the epineurium remains intact. Fourth- and fifth-degree injuries require surgical repair because little to no recovery can be expected.
Mackinnon and Dellon later added a sixth degree of nerve injury to Sunderland’s classification. Sixth-degree injury represents a mixed picture nerve injury. Along a given segment of nerve, 1 section might sustain a first-degree injury, but another portion of the nerve sustained a fourth-degree injury. The exact pattern of nerve injury is often difficult to determine, relying on both clinical examination and electromyography findings.
Nerve degeneration and regeneration
Axonal transection triggers a sequence of events within the nerve proximally and distally to the injury site. Proximally, the cell body undergoes chromatolysis, a reorganization of rough endoplasmic reticulum and shift in metabolic production from neurotransmitters to structural materials for axon growth and repair. , The proximal axon undergoes traumatic degeneration to the next node of Ranvier. Distally the transected nerve undergoes Wallerian degeneration. Macrophages and Schwann cells phagocytize the myelin debris at the distal axon.
Schwann cells align in columns to form bands of Bugner with nerve growth factor receptors present on the surface acting as a scaffold or tunnel for the regenerating axon. The distal nerve and surrounding cells produce a gradient of neurotrophic factors and neurite-promoting factors to guide axon regeneration in a process known as neurotropism. The regenerating axon detects permissive or inhibitory signals from the microenvironment via sprouts and growth cones, which guide direction of growth based off motor versus sensory, topographic, tissue, and end-organ signaling specificity. The neurotrophic growth factors important for nerve survival are transported retrogradely to the cell body. Neurite-promoting factors, laminin and fibronectin, promote growth extension of the axons toward the distal target. Additional growth factors and contact factors promote cell migration and nerve generation. The process of nerve generation proceeds at roughly 1 mm/d or 1 inch/mo.
Pathophysiology and classification of neuroma formation
Nerve injuries can result from pressure, stretch, chronic irritation, ischemia, crush injury, sharp transection, iatrogenic, and poor repair of prior injuries. All neuroma formation occurs because of some degree of nerve injury followed by improper intrinsic nerve repair.
Neuromas can be divided into 2 main categories: terminal neuromas and neuroma-in-continuity (NIC). Terminal neuromas result from complete transection of a nerve or amputation of a distal extremity. This injury pattern is classified as neurotmesis or fifth-degree Sunderland. In this case, the distal axon is too far away for neurotrophic and neurite-promoting factors to be sensed by the proximal axon. The proximal axon cannot progress toward its distal target, resulting in unorganized fascicular overgrowth at its end. NIC results from damage to the perineurium in a Sunderland fourth-degree or Mackinnon mixed injury. Damage to the perineurium allows fascicular escape and disorganized nerve repair of axons in the surrounding epineurial tissue, along with deposition of scar tissue by fibroblasts. An NIC following nerve graft repair is also possible secondary to defect length, scarring, or mismatched fascicles, permitting only some fascicles to regenerate appropriately. Disorganized patterns of axons with accompanying proliferating fibroblasts, Schwann cells, and blood vessels are characteristic findings of neuroma on microscopic analysis.
The exact incidence of neuroma formation after injury is unknown. However, postoperative, painful neuroma rates range from 1% to 30%. The amount of axoplasmic flow, ratio of fascicles to epineurial tissue, and nutritional status of the peripheral nerve appear to affect neuroma formation. Diagnosis of neuroma is clinically characterized by pain associated with scar, altered sensation within the given nerve distribution, and a Tinel sign. Ultrasound sonography can help determine the exact location of neuroma and reproduce symptoms.
Neuropathic pain associated with neuroma can be classified into 4 types according to Sood and Elliot : spontaneous pain, pain with pressure over neuroma, pain on movement of adjacent joints, and hypersensitivity with light skin touch. The cause of neuroma pain is incompletely understood as well, but appears to be multifactorial in nature, including local and system changes. Repetitive mechanical irritation owing to tethering scar tissue or compression in an area of thin overlying tissue can result in persistent pain. Increased scarring and myofibroblast proliferation have been associated with neuropathic pain. Myofibroblasts have been postulated to cause pain by direct contraction around surrounding nerve tissue and release of alpha smooth muscle actin. Abnormal accumulation of sodium and potassium channels within the neuroma has been found to account for hyperexcitability and spontaneous discharge from injured nerves. Local tissue damage surrounding neuromas can result in an inflammatory response thought to chemically sensitize nociceptive A-delta and C fibers resulting in transmission of painful stimuli. Melzack and Wall proposed another mechanism for neuroma pain termed gate control theory representing a complex interplay between the substantia gelatinosa, dorsal column of the spinal cord, and T cells in the dorsal horn. Reorganization and release of inflammatory cytokines, such as tumor necrosis factor-alpha and interleukin-1, within the central nervous system have also been proposed as mechanisms for neuroma pain.
The pain pathway surrounding symptomatic neuromas remains complex and poorly understood. Multiple pharmacologic therapies have been used to try to break this chronic and unpredictable pain cycle. Topical therapy and neuromodulation are often attempted before any surgical intervention. More than 150 surgical interventions have been used to attempt neuroma prevention, treatment, and pain control. Burying the proximal severed nerve end in muscle or bone was the traditional mainstay of neuroma prevention. Neurolysis, nerve wrapping, neuroma resection, and reconstruction. Emerging techniques involving targeted muscle reinnervation and regenerative peripheral nerve interfaces have shown promising results in neuroma prevention and management and are discussed in subsequent articles. A comprehensive understanding of nerve anatomy, injury, and repair techniques should be used when dealing with neuroma formation and its physical manifestations.
In summary, neuroma formation occurs after nerves are subject to significant damage. The injury may be from a sharp object, blunt trauma, or a traction injury. Complete nerve injuries result in end neuromas, where, as partial injuries, result in NICs . Sprouting axons engulfed in a disarray of fibrosis form a disorganized mass of small, unmyelinated minifascicles. The painful neuromas are associated with enhanced alpha smooth muscle actin. The greater the intensity of the alpha smooth muscle actin, the more painful the neuroma. Also, the size of the neuroma is influenced by the nutritional status of the nerve, the axoplasmic flow, and the extent of Schwann cell and myofibroblast proliferation.
Disclosure
Nothing to disclose.
References
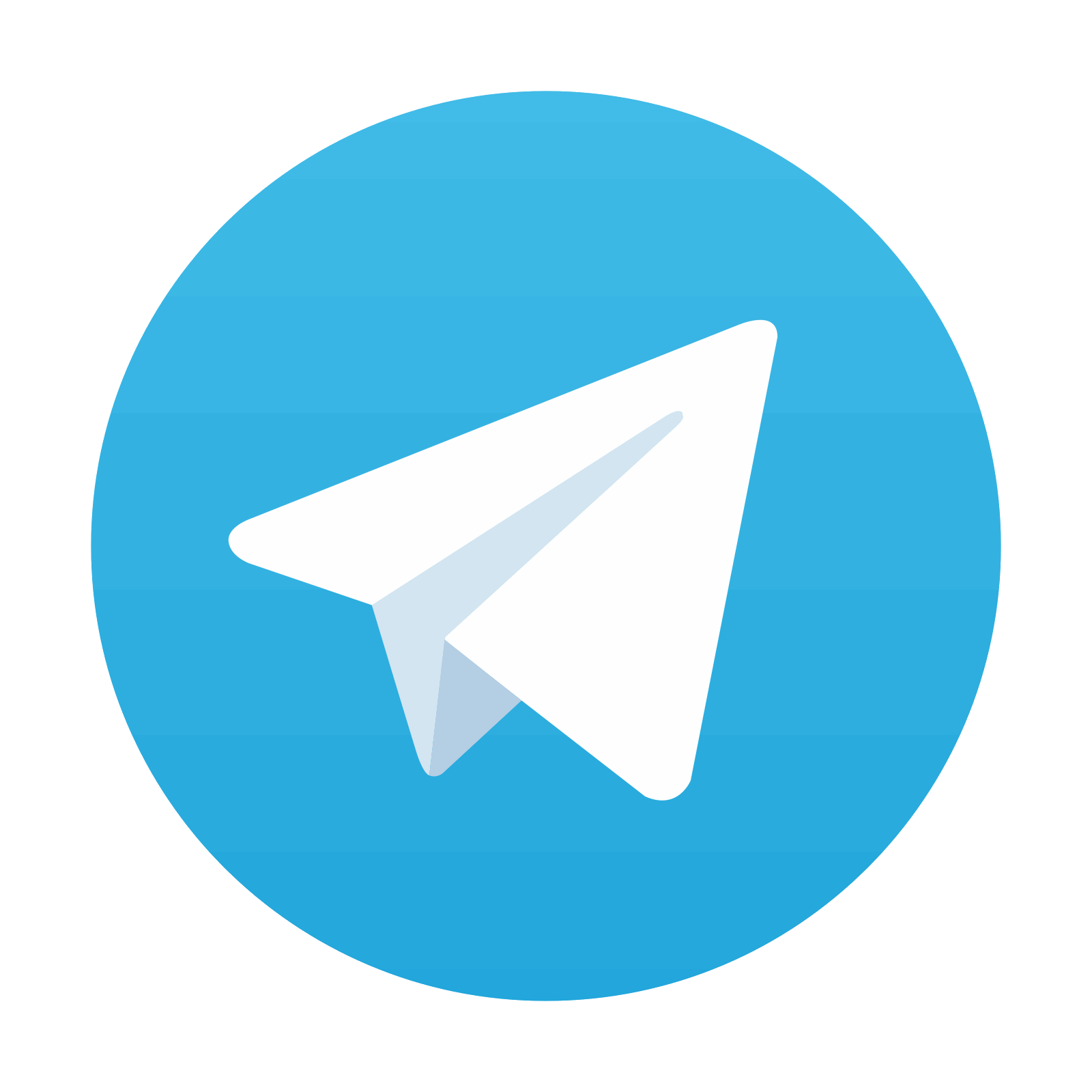
Stay updated, free articles. Join our Telegram channel

Full access? Get Clinical Tree
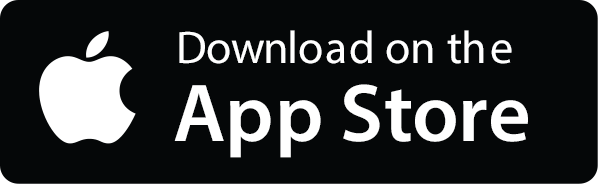
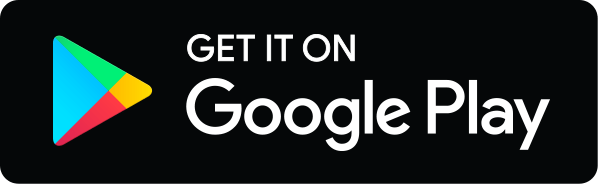
