Humoral Immunity and Complement: Introduction
|
B Lymphocytes
During evolution, jawed vertebrates developed the capacity to respond with exquisite specificity to foreign organisms.1 Specific immunity is characterized by an enormous diversity of possible responses and by refinement in the immune response with successive exposures to the organism.2 The cells that can discriminate with fine specificity through their vast repertoire of receptors are lymphocytes. Specific immunity, also called adaptive immunity because it develops as an adaptation to infection, can be segregated into humoral immunity, mediated by antibodies produced by B lymphocytes, and cellular immunity, mediated by T lymphocytes. These two forms of specific immunity developed to serve different functions. Humoral immunity is directed primarily toward extracellular antigens such as circulating bacteria and toxins. Cellular immunity is directed primarily toward antigens that infect or inhabit cells (see Chapter 10). To combat extracellular pathogens, the defending agent needs to be abundant and widely distributed in the body, particularly at its interfaces with the environment. Antibodies fulfill these characteristics by being capable of being secreted in great quantity from the cells that produce them and by being distributed in blood, mucosa, and interstitial fluid. In addition, antibodies can attach through Fc receptors (FcRs) to the surface of certain other cells of the immune system, such as mast cells, conferring antigen specificity to cells that do not have their own endogenously produced antigen-specific receptors. In addition to their major function in humoral immunity as antibody producers, B lymphocytes have a role in antigen presentation, regulation of T-cell subsets and dendritic cells, organization of lymphoid tissues, and cytokine and chemokine production.3,4
Antibodies, or immunoglobulins (Ig), are a family of glycoproteins that share a common structure.2,5,6 The antibody molecule has a symmetric Y-shape consisting of two identical light chains, each about 24 kDa, that are covalently linked to two identical heavy chains, each about 55 or 70 kDa, that are covalently linked to one another (Fig. 37-1). Within the light and heavy chains are variable and constant regions. The major function of the variable region is to recognize antigen, whereas the constant region mediates effector functions. The light and heavy chains contain a series of repeating, homologous units of about 110 amino acids that assume a globular structure and are called Ig domains. The Ig domain motif is found not only in antibody molecules but also in a variety of other molecules of the Ig “superfamily,” including the T-cell receptor, the major histocompatibility complex (MHC), CD4, CD8, intercellular adhesion molecule 1, among other molecules. The light chain has two major domains, (1) a variable (VL) and (2) a constant (CL) domain. The heavy chains have four or five domains, a variable (VH) and three (in IgA, IgD, and IgG) or four (in IgM and IgE) constant (CH1–4) domains. In IgA, IgD, and IgG, there is a hinge region between CH1 and CH2 that confers additional flexibility to the molecule. The variable domains are at the N-terminus. At the C-terminus are the constant domains and, in the heavy chains of membrane-bound antibodies, the transmembrane and cytoplasmic domains.
Within the variable regions of the light and heavy chains are three areas of intense variability called hypervariable regions. These three regions, which are in proximity to one another in the three-dimensional structure of the antibody, are the areas most responsible for binding antigen. Because the hypervariable regions form a shape complementary to that of the antigen, the hypervariable regions are also called the complementarity-determining regions. The unique areas formed by the hypervariable regions are present in too low an amount in the individual to generate self-tolerance. Thus, the immune system may not distinguish the unique portion of the antibody as self and may produce antibodies to that region of the antibody. The area of the antibody capable of generating an immune response is called an idiotope, and antibody responses to idiotopes result in a network of idiotypic–anti-idiotypic interactions that may help regulate the humoral immune response.7
There are two types of light chains, κ and λ, each encoded on different chromosomes. Each antibody molecule has either two κ or two λ chains, never one of each. The functional differences, if any, between κ and λ are not known. There are five types of heavy chains, (1) α, (2) δ, (3) ϵ, (4) γ, and (5) μ, corresponding to the antibody classes IgA, IgD, IgE, IgG, and IgM, respectively. The different heavy chain classes have significantly different functions, as discussed in Section “Antibody Classes”. The IgA and IgG classes contain closely related subclasses, consisting of IgA1 and IgA2, and IgG1, IgG2, IgG3, and IgG4 (Table 37-1).
A | Subtypes | Secreted Form | Approximate Molecular Weight of Secreted Form (kDa) | Serum Concentration (mg/mL) | Serum Half-Life (Days) | Functions |
---|---|---|---|---|---|---|
IgM | None | Pentamer, hexamer | 970 | 1.5 | 5 | Primary antibody response; antigen receptor on naive B cells; complement activation |
IgD | None | Monomer | 180 | Trace | 3 | Antigen receptor on naive B cells |
IgA | IgA1 | Monomer, polymer (usually dimer) | 160 (monomer), 390 (secretory IgA) | 3 | 6 | Mucosal immunity; neonatal immunity |
IgA2 | Monomer, polymer (usually dimer) | 160 (monomer), 390 (secretory IgA) | 0.5 | 6 | ||
IgG | IgG1 IgG2 IgG3 IgG4 | Monomer Monomer Monomer Monomer | 150 150 170 150 | 9 3 1 0.5 | 23 23 7 23 | Neonatal immunity; opsonization; complement activation (except IgG4); phagocytosis; antibody-dependent cell-mediated cytotoxicity; feedback inhibition of B cells |
IgE | None | Monomer | 190 | 0.05 | 2 | Immediate hypersensitivity; defense against parasites |
Enzymatic digestion of IgG molecules by papain results in three cleavage products, two identical Fab fragments consisting of a light chain bound to the V–CH1 region of the heavy chain and an Fc portion consisting of two CH2–CH3 heavy chains bound to each other. Fab was so named for its property of antigen binding, and Fc was so named for its property of crystallizing. When IgG is digested by pepsin, the C-terminal region is digested into small fragments. The remaining product consists of the Fab region along with the hinge region. Fab fragments containing the hinge region are termed Fab′. When the two Fab′ fragments in an antibody molecule remain associated, the fragment is called F(ab′)2.
Antibody Classes
IgM is evolutionarily the most ancient antibody class and is the first Ig molecule to be expressed during B-cell development.1 Its secretory form exists mainly as a pentamer consisting of five IgM molecules joined at their C-termini by tail pieces and stabilized by a molecule called a joining (J) chain. The engagement of membrane-bound IgM by antigen results in the activation of naive B cells. Secreted IgM recognizes antigen, usually through low-affinity interactions, and it can activate complement. IgM is the major effector of the primary antibody response. Although IgM interactions are typically low affinity, IgM can be very effective in responding to a polyvalent antigen (such as a polysaccharide with repeating epitopes) because its pentameric structure allows for multiple low-affinity interactions, resulting in a high-avidity interaction. (Avidity refers to the overall strength of attachment, whereas affinity refers to the strength of attachment at a single antigen-binding site.)
The IgD molecule exists primarily in a membrane-bound form and is the second antibody class to be expressed during B-cell development. Its function is not completely understood, but in its membrane-bound form it can serve as an antigen receptor for naive B cells.8 Secreted IgD has been found on the surface of basophils, where it induces production of antimicrobial, opsonizing, inflammatory, and B-cell–stimulating factors.9
IgA is the most abundant Ig in the body, being present in large quantity at mucosal sites. It is responsible for mucosal immunity and is secreted in breast milk, thus contributing to neonatal immunity. In its secreted form, it exists as a monomer, dimer, or trimer, with the multimers being formed by interactions between tail pieces and stabilized by the J chain. For transport across epithelial surfaces, IgA dimers attach to a type of FcR called the polymeric Ig receptor.10 Once the transport process is complete, the IgA dimers remain attached to the extracellular portion of the receptor, called the secretory component, which protects the IgA from proteolysis. Cells of the immune system that have receptors for IgA include neutrophils, eosinophils, and monocytes.
IgG is the most abundant Ig in the circulation. Its secreted form is a monomer. IgG plays an important role in secondary antibody responses, and its interactions with antigen tend to be high affinity, particularly as the immune response matures. A number of cells have FcRs for IgG, including monocytes, neutrophils, eosinophils, natural killer (NK) cells, and B cells. IgG opsonizes (coats) antigen, allowing phagocytosis of the antigen, and activates complement. An exception is IgG4, which does not activate complement. IgG is important in neonatal immunity, as it is the only Ig class to cross the placenta, and it is secreted in breast milk. The interaction of IgG with the MHC class I-related receptor FcRn is involved in the delivery of IgG across the placenta as well as in prolonging its level in the circulation.11 The serum half-life of IgG is 23 days, considerably longer than that of the other Ig classes.
IgE is found in very small amounts in the circulation. High-affinity receptors for the Fc portion of IgE are present on mast cells, basophils, and eosinophils, and low-affinity receptors are present on B cells and Langerhans cells. In mast cells and basophils, IgE engagement with antigen activates the cells. IgE mediates immediate hypersensitivity, but its principal protective role may be to combat parasites.
Mechanisms for the Generation of Antibody Diversity
The information encoded by an individual’s DNA is limited by the need for the DNA to fit into a package of the size of a cell. This space is far too small for sufficient DNA to encode billions of different lymphocyte receptors if the genes were encoded separately. Lymphocytes have adapted to this limitation by special mechanisms that increase by orders of magnitude the number of different possible antigen receptors.12 Each clone of B cells produces identical antigen receptors (i.e., antibodies) with unique specificity. It is estimated that an individual has approximately 107 different B-cell clones, resulting in 107 distinct antibodies. A major mechanism for generating this enormous diversity is gene rearrangement, whereby segments of DNA within a lymphocyte undergo somatic recombinations.13 Light chain genes contain three regions, (1) V (variable), (2) J (joining), and (3) C (constant), and heavy chain genes contain four regions, (1) V, (2) D (diversity), (3) J, and (4) C. Within each region are many gene segments from which to select for the final antibody product, which is comprised of one gene segment randomly selected from each region. The initial event in antibody formation is the joining of one D and one J segment from a heavy chain gene, with subsequent deletion of the DNA between the two segments. Next, a V segment is selected to join to the DJ segment, and any remaining D segments are deleted. The VDJ complex has attached 3′ to it any remaining J segments plus the C region. The unused J segments are removed during RNA processing. A similar process occurs in light chain loci; because there are no D segments in light chain loci, a VJ rather than a VDJ complex is formed. (Particularly in the k locus, VJ recombination may occur through a somewhat different mechanism involving inversion of the DNA without deletion of intervening sequences, but the functional result is the same.)
The ability to select one segment each from the many segments available in the V, D, and J regions leads to a vast increase in the repertoire of possible antibodies. Additional diversity is generated by the juxtaposition of a rearranged light chain to a rearranged heavy chain; by the addition, deletion, or transposition of nucleotides at the junctions between V and D, D and J, and V and J segments, a phenomenon called junctional diversity; and by somatic hypermutation after antigen stimulation (see below).
B-Cell Maturation
|
Cells destined to become mature B cells undergo an orderly progression of events during development, resulting in the formation sequentially of heavy chains, light chains, and whole antibody molecules, with checkpoints to select against cells making unproductive gene rearrangements or autoreactive antibodies, and survival signals to select for cells making potentially useful antibodies. The process of B-cell development occurs in distinct stages, characterized by specific events and identifiable by specific cell surface markers and Ig gene expression.
Bone marrow and fetal liver stem cells that give rise to B cells are initially pluripotent.2,14 Stem cells developing in the lymphocytic pathway initially become common lymphoid progenitors, which can give rise to B, T, or NK cells. B cells originating from fetal liver are mainly B1 cells (see Section “B-Cell Activation and Antibody Function”), whereas B cells originating in the bone marrow are primarily follicular B cells. Cells and extracellular molecules in the stromal microenvironment provide signals required for differentiation of lymphocytes. Induction of the transcriptional regulators EBF, E2A, and Pax-5 leads to the expression of proteins critical to B-cell development. Posttranscriptional regulation of mRNA by RNA-binding proteins and microRNAs provides further control over the process of B-cell differentiation.15
The earliest cell committed to the B-cell lineage is called a pro-B cell. At the pro-B cell stage, the cell expresses recombination activating gene (RAG) and terminal deoxyribonucleotidyl transferase (TdT) proteins, which will be needed subsequently for somatic recombination and nucleoside transfers involved in junctional diversity, respectively. At the pro-B-cell stage, limited somatic recombination has taken place, and Ig is not yet expressed.
The next stage of B-cell maturation is represented by the pre-B cell and is marked by the synthesis of a cytoplasmic μ heavy chain. Because light chains are not yet expressed at this stage, surface Ig is not present. Some of the μ heavy chains associate with invariant molecules called surrogate light chains and with the signal transducing proteins Ig α and Ig β to form complexes called pre-B cell receptors. Cells that have synthesized heavy chains that are capable of forming part of a pre-B cell receptor are selected for at this stage, as pre-B cell receptors provide important signals for survival, proliferation, and maturation.
The formation of light chains marks the next stage in B-cell maturation, the immature B-cell stage. When light chains join with the μ heavy chains, an IgM molecule results and can be expressed on the cell surface in association with Ig α and Ig β. Although the presence of a B-cell receptor complex confers the ability to recognize specific antigens, at this stage such recognition does not result in proliferation or differentiation. Rather, the cells may undergo negative selection when antigen is encountered. Immature B cells recognizing self-antigen may be negatively selected through deletion,16 anergy, or receptor editing, a process of secondary gene rearrangement by which a new, nonself specificity is acquired.17
The exit of immature B cells from the bone marrow to the spleen marks the beginning of the next stage, the transitional B-cell stage.18 Transitional cells gradually acquire surface IgD, CD21, and CD23 expression and become more immune competent. Alternative splicing of RNA allows the simultaneous expression of IgM and IgD. At the beginning of the stage, cross-linking of the B-cell receptor leads to negative selection. With further maturation, transitional cells become responsive to T-cell help and lose sensitivity to negative selection.
The mature B cell expresses IgM and IgD and is competent to respond to antigen. The cell is considered naive because it has not been activated by antigen. The majority of mature B cells circulate through peripheral lymphoid tissues (spleen, lymph nodes, mucosal lymphoid tissue) and are called follicular B cells, or recirculating B cells. B cells are recruited to the follicle by the chemokine CXCL13, secreted by follicular dendritic cells, and survive in the follicle with the assistance of a cytokine called BAFF (B-cell activating factor), also known as BLyS (B lymphocyte stimulator). A small percentage of mature B cells home to the marginal zone of the spleen and remain resident there.
The encounter of antigen by mature naive B cells leads to B-cell activation, proliferation, and differentiation (see Section “B-Cell Activation and Antibody Function”). A subset of B cells become memory B cells, which can persist for long periods apparently without stimulation by antigen, and which respond rapidly if the antigen is encountered subsequently.19 Another subset of B cells differentiates into cells that make progressively less membrane-bound Ig and more secreted Ig. The terminally differentiated B cells committed to the production of secreted Ig are plasma cells and have abundant rough endoplasmic reticulum, consistent with the function of the cells as antibody factories.20
Antigens Bound by B Cells
B cells recognize a variety of macromolecules, including proteins, lipids, carbohydrates, and nucleic acids. The portion of the molecule recognized by the antibody is called an epitope or determinant. B cells recognize both linear epitopes (epitopes formed by several adjacent amino acids) and, quite commonly, conformational epitopes (epitopes present as a result of folding of the macromolecule).21 In contrast to B cells, T-cell responses are almost entirely restricted to linear epitopes of peptides.
Macromolecules, particularly large proteins, may contain several different epitopes, and a humoral response to a macromolecule typically is comprised of multiple different antibodies. Although each different antibody is specific for a given epitopic configuration, similarities in epitopes may exist such that an antibody to a given epitope on a given macromolecule also may be able to bind a different epitope on a different macromolecule. This phenomenon is called cross-reactivity, and may be important in the genesis of autoimmune antibody responses.
Macromolecules that have multiple identical epitopes are classified as being polyvalent or multivalent. Antibodies to these macromolecules or aggregates of macromolecules may form complexes called immune complexes with the antigen. At a particular concentration of antibody and antigen, called the zone of equivalence, a large network of linked antigens and antibodies forms. At lower or higher concentrations of antibody or antigen, the complexes are much smaller. Immune complexes, formed in the circulation or in tissue, may be responsible for disease through the initiation of an inflammatory response.
B-Cell Activation and Antibody Function
|
On cross-linking of the mature B-cell receptor by antigen, clustering of receptors initiates signaling transduced by Igα and Igβ. The complex signaling cascade involving the phosphorylation of tyrosine kinases, including Lyn, Fyn, Btk, and Syk, eventuates in the expression of genes involved in B-cell activation.22 B-cell activation is facilitated by second signals, one of which is provided by the complement protein C3d.23 Complement fragment C3d is formed as a result of complement activation through any of the complement activation pathways (see Section “Complement”). The B-cell surface contains a coreceptor complex consisting of complement receptor 2 (CR2), CD19, and CD81 (also called TAPA-1, or target for antiproliferative antigen-1). Simultaneous binding of antigen by antibody on the B-cell surface and of C3d by CR2 leads to markedly increased B-cell activation. B-cell activation may also occur through Toll-like receptors that recognize specific microbial products.24
The subsequent response to an antigen often involves a complex interaction between B cells and T cells, leading to a fine-tuning of the immune response.25 Recognition of antigen by both B cells and T cells leads to increased expression of cell surface proteins and cytokines that render these cells increasingly capable of migrating toward and productively interacting with each other. T cells recognizing peptide-class II MHC complexes on dendritic cells receive a primary signal from the complex and a secondary signal from costimulatory interactions involving the binding of B7–1 and B7–2 on dendritic cells to CD28 on T cells.26 These activated T cells express CXCR5, the ligand for CXCL13, which results in T-cell migration toward the follicle and therefore increasingly toward B cells. In response to a protein antigen, B cells take up the antigen, process it, and present processed antigen on the cell surface in complex with class II MHC. Activated B cells express less CXCR5, which allows them to migrate from the follicle toward the T-cell zone. At the boundary between follicles and T-cell zones, activated T cells interact with B cells and provide signals to the B cells through the binding of CD40 on B cells to CD40 ligand (CD154) on T cells and through the action of cytokines, notably interleukin 2 (IL-2), IL-4, IL-21, BAFF, and APRIL (a proliferation-inducing ligand).2 These signals will be necessary for subsequent class (heavy chain isotype) switching, affinity maturation, and memory B-cell generation. The overall effects on B cells are stimulation of proliferation and differentiation.
At this phase, some of the activated B cells become short-lived plasma cells, which provide a prompt initial response to an antigen, while others migrate back from the periphery of the follicle to proliferate rapidly and form germinal centers. It is primarily in the germinal centers that class switching, affinity maturation, and generation of memory B cells occur. Class switching from IgM to IgA, IgE, or IgG occurs as a result of T cell–B cell interactions.27,28 The determination of the antibody class selected is based on the site where the antigen is encountered and the cytokine milieu. For example, B-cell responses to antigens encountered on mucosal surfaces characteristically result in class switching to IgA, and transforming growth factor-β is an important contributing cytokine. IL-4 is an important signal for class switching to IgE.
T-cell interaction with B cells also results in affinity maturation, whereby the affinity of antibodies for the antigen progressively increases. During affinity maturation, somatic hypermutations in antibody genes result in antibodies with both greater and lesser affinity for the antigen.29,30 Those antibodies with greater affinity confer a survival advantage on the B cells that produce them. Progressively, the population of B cells evolves in favor of those producing higher affinity antibodies for the antigen. Both class switching and affinity maturation require the expression of an enzyme called activation-induced cytosine deaminase (AID).31
The culmination of germinal center activity is the formation of memory B cells and long-lived plasma cells.32
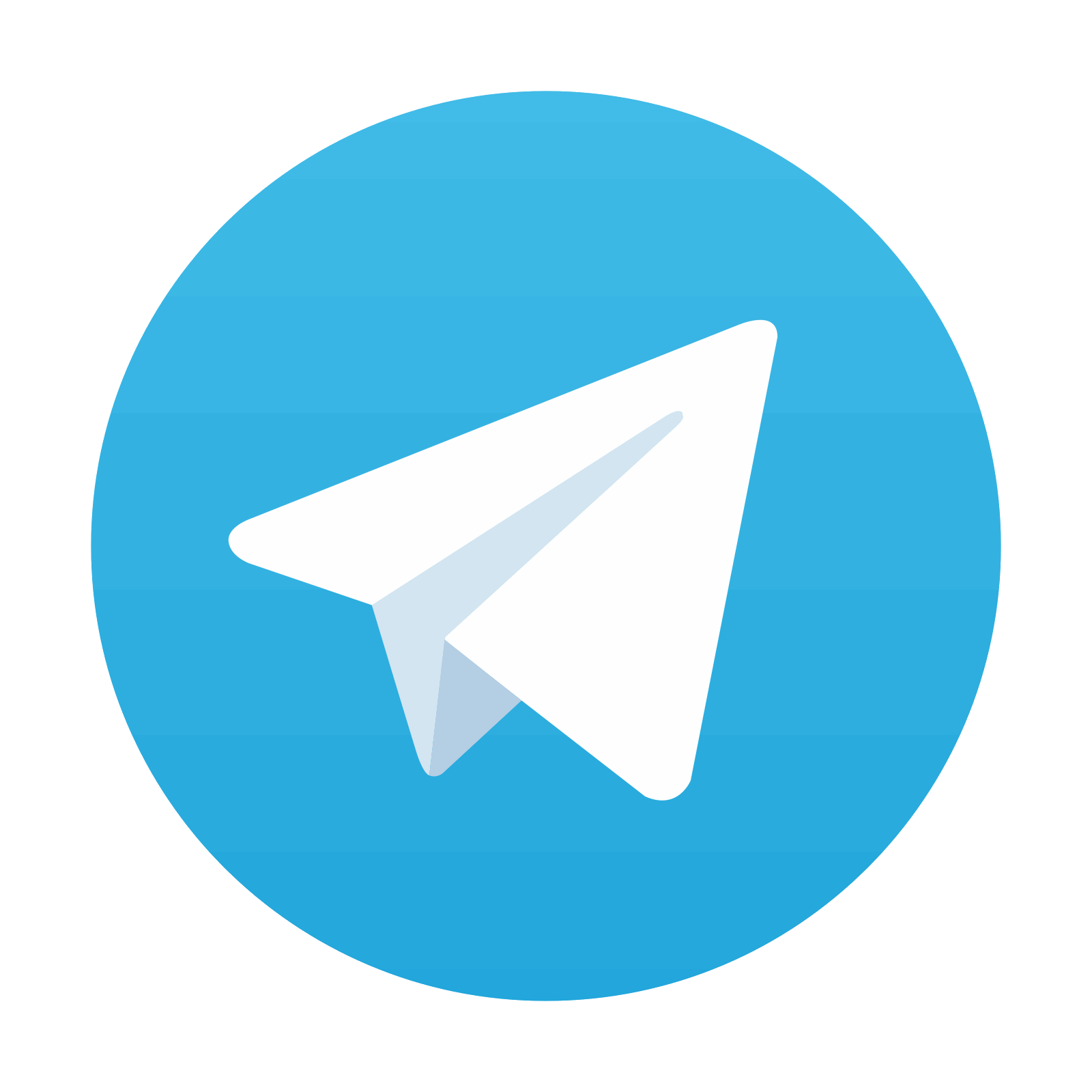
Stay updated, free articles. Join our Telegram channel

Full access? Get Clinical Tree
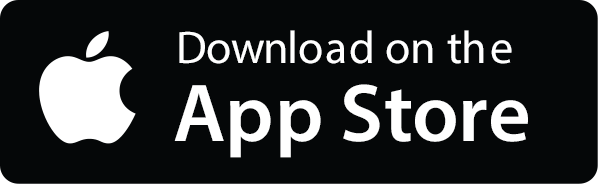
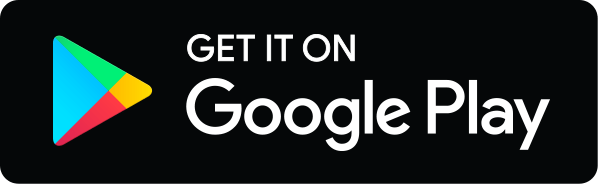
