Fig. 10.1
Different human skin membranes for absorption studies: (a) full thickness skin, (b) dermatomed skin, (c) heat separated epidermis, and (d) enzyme split stratum corneum (According to Schaefer et al. 2008)
10.2.1.2 Dermatomed or Split Skin
Dermatomed skin can be prepared by means of a dermatome which performs surface parallel sections, composed of the epidermis and including the stratum corneum as well as parts of the dermis (Fig. 10.1b). Normally, a slice thickness of 200–400/500 μm is recommended for human skin (OECD 2004b; USEPA 2004). In doing so, the hair follicles will be cut, but the resulting holes will rapidly close during incubation with the acceptor medium due to swelling of the tissue, especially if aqueous donor and receptor fluids are used. During dermatomization care must be taken to avoid mechanical damage of the SC, the main barrier of the skin. To overcome this problem, protection of the skin surface by a plastic foil is indicated (Brain et al. 1998). Typically, dermatomed skin is used for permeation studies, especially if the influence of the hydrophilic layers of the epidermis and dermis should be considered on skin absorption (influence of partition step between SC and viable epidermis/dermis). Moreover, dermatomed skin might be of particular interest to study the fate of very lipohilic drugs (e.g., pesticides) due to the limited solubility in rather the hydrophilic viable epidermis and dermis.
10.2.1.3 Epidermis Sheets
For the separation of epidermis (consisting of SC and viable epidermis, see Fig. 10.1c) and dermis mechanical, thermal, and chemical techniques are available. With all techniques splitting occurs at the basal layer of the epidermis. Mechanical division can be achieved by applying a vacuum to the skin surface (suction technique (Kiistala 1968)). However, only relatively small areas can be treated in such a way. Thermal treatment is most common. This technique was established by Kligman and Christophers (Kligman and Christophers 1963). A specimen of full thickness skin is immersed in water of about 60 °C for a time period of 30–120 s. Afterwards the epidermis sheet can easily be removed from the dermis by means of a forceps or a spatula. Care has to be taken not to mechanically damage the epidermis by this procedure. Before use, the epidermis should be floated on a physiological buffer solution to unfold it completely. It was shown that this treatment does not result in changes of the barrier function. Several methods of chemical separation are reported (Kligman and Christophers 1963; Lee and Parlicharla 1986; Scott et al. 1986). However, due to the use of strong alkaline or acidic solutions, the buffer capacity of the skin will be changed and this may also influence the penetration of ionizable substances. Epidermis sheets provide a reduced resistance to permeation in comparison to dermatomed skin due to the absence of the dermis layer. They are typically used for permeation studies like dermatomed skin.
10.2.1.4 Stratum Corneum Sheets
Stratum corneum sheets (Fig. 10.1d) can easily be isolated by incubating full thickness skin or heat separated epidermis in an aqueous buffer solution of pH 7.4 containing trypsin for 24 h at 37 °C (Kligman and Christophers 1963). The SC may be separated from the underlying tissue by gentle shaking. To remove residual amounts of trypsin, the sheets can be rinsed in isotonic aqueous buffer solution pH 7.4. Due to its fragility the separated SC should be taken up by a supporting membrane, for example, cellulose membrane (Reichling et al. 2006). In addition, the SC can be lifted by a Teflon foil and afterwards be allowed to dry for storage. After reconstitution with isotonic aqueous buffer pH 7.4, the SC can be used for in vitro experiments.
The influence of the type of skin preparationused in permeation experiments is depicted exemplarily in Fig. 10.2. It is clearly shown that with full thickness skin the permeation is reduced compared to heat separated epidermis and SC, which do not differ in permeability but provide differences in the lag-time. SC sheets are primarily used for mechanistic studies and partition coefficient determination.


Fig. 10.2
Permeation experiments with the FD-C. Permeation of flufenamic acid (mg/cm2 ± S.E.; n = 3–5) through different skin layers in dependence on the incubation time (h) (Figure taken from Wagner et al. (2001))
10.2.1.5 Skin with Impaired Barrier Function
In diseased, injured, or premature skin, the barrier function of the skin is reduced. Skin with an impaired barrier is sometimes also desired for in vitro skin absorption experiments. By stripping with adhesive tape (Wagner et al. 2000) or glue, the outermost cornified cell layers of the SC can be gradually removed, allowing to generate different levels of a decrease in barrier function, corresponding to differently increased permeabilities. In order to compare the results of such experiments, the degree of damage has to be known. In principal this can be done by different methods, e.g., by determining the amount of SC removed (Hahn et al. 2010) or measuring the transepidermal water loss (TEWL) (Kalia et al. 1996; Russell et al. 2008). Complete removal of stratum corneum and epidermis can best be achieved preferably by rigorous dermatomization rather than by heat separation or trypsinization. The latter two methods may compromise the protein structure of the epidermal/dermal tissue due to precipitation or digestion of proteins.
10.2.2 Animal Skin
As human skin has only limited availability, alternatives are necessary. Besides, due to widespread demands in skin absorption studies based on regulatory aspects (e.g., REACH program of the EU (EC 1907/2006, 2006) the pressure to establish alternative models was enforced. In addition, some researchers have ethical and cultural restraints to use human skin (Hikima et al. 2012). Skin from mouse, hairless rat, hamster (cheek pouch), snake (shed skin), pig (ear, flank, abdomen, or back), and cow udder has been suggested as alternatives (Haigh and Smith 1994). However, depending on the animal species used, differences in SC thickness, number of corneocyte layers, hair density, water content, lipid profile and morphology, and different permeabilities in comparison to human skin have been found. Typically the permeability of animal skin was enhanced in comparison to human skin (Bronaugh et al. 1982; Netzlaff et al. 2006b). In general, porcine skin is reported to match human skin best. In addition, porcine skin, especially the ear, is easily available from slaughter houses. However, it has to be kept in mind that porcine skin must not be scalded. Moreover, there are references pointing also to the alternative of cow udder skin (Kietzmann et al. 1993; Netzlaff et al. 2006b; Pittermann and Kietzmann 2006). By the 7th Amendment to the Cosmetic Directive for cosmetic products (EC (2003c) Directive 2003/15/EC; Rossignol MR 2005) a ban of tests on animals and of the marketing of products/ingredients tested on animals came into action in September 2009. Since then only alternative methods such as animal skin form sources of food supply (pig ear, cow udder) or bioengineered skin surrogates can be used.
10.2.3 Bioengineered Skin
For some time bioengineered skin has been introduced in the field of skin research due to the limited supply of human skin, some ethical or cultural restraints to use human skin, and the “3R”-initiative concerning animal welfare (“Refinement, Replacement, Reduction”) (Russell and Russell 1957). Firstly, skin corrosion (Kandárová et al. 2006a, b), sensitization, (Bernard et al. 2000; Spielmann et al. 2000), metabolic phenomena (Gysler et al. 1999), and phototoxicity (Liebsch et al. 1999) were addressed and afterwards skin permeability was evaluated (Dreher et al. 2002). Meanwhile various commercial reconstructed epidermis models are available (Netzlaff et al. 2005) and also accepted for skin corrosion (OECD 2004c, 2006) and irritation testing (OECD 2010). Moreover, many attempts have been made to compare in detail bioengineered skin to human skin and animal skin (Schreiber et al. 2005; Netzlaff et al. 2005, 2007). In two large studies sponsored by the German BMBF (Federal Ministry of Education and Research), the usefulness of the commercially available reconstructed epidermis models EpiskinTM (L’Oreal, France), Epiderm® (MatTek corporation, USA), SkinEthic® (SkinEthic Laboratories, France) were tested with nine substances in aqueous solution covering a wide range of lipophilicities and molecular weights (Schäfer-Korting et al. 2006, 2008). As a result, it could be clearly shown that all reconstructed epidermis models were more permeable than human heat separated epidermis and dermatomed porcine skin; however, the rank order of permeability agreed reasonably among these models. Besides, it could be shown that reconstructed epidermis provides less variability in comparison to human and animal skin which is consistent with results from the EDETOX (Evaluations and Predictions of Dermal Absorption of Toxic Chemicals) project (van de Sandt et al. 2004). A review about morphology, biochemical characterization, and application of the bioengineered models EpiskinTM, Epiderm®, and SkinEthic® is reported by Netzlaff et al. (2005). Besides, a list of materials tested with Epiderm® is provided by MatTek, USA (www.mattek.com/pages/products/epider/materials-tested).
Additional reports are available for other, sometimes self-made, bioengineered skin models. Suhonen et al. reported permeability experiments for 18 compounds in buffered aqueous solutions with a cell culture model based on keratinocytes from rat skin (Suhonen tm et al. 2003). A close relationship to isolated human cadaver skin was found; however, again the permeability in the cell culture model was enhanced. The group of Mueller-Goymann developed an artificial skin construct based on collagen and HaCaT (human adult low calcium high temperature keratinocytes) cells cultivated at the air-liquid interface (Savic et al. 2009). Investigating the permeation of caffeine and diclofenac from various formulations containing natural surfactant/fatty alcohols mixed emulsifiers they reported that the vehicle skin interaction was mirrored in the artificial skin construct.
A full-thickness commercial skin model, the Phenion® model (Henkel AG & Co.KGaA, Germany) was tested by Ackermann et al. (2010) for percutaneous absorption testing of the OECD (Organisation of economic Co-operation and Development) reference compounds caffeine and testosterone. They concluded that the results closely paralleled human skin (Ackermann et al. 2010). One of the major problems with bioengineered skin is that the sheets must be transferred to standard diffusion cells after the cell layer has been punched out from the cultivating support. To overcome this drawback, Grégoire et al. improved the experimental setup for screening skin absorption with the reconstructed epidermis model Episkin® (Grégoire et al. 2008). By modifying the culture conditions, it was possible to omit bypass diffusion in the inserts. In contrast to studies in static diffusion cells the cell layer no longer has to be punched out. In summary, although there are references suggesting the helpfulness of bioengineered skin in skin absorption studies, the basic problem is the lack of building up a barrier at the same level as natural human skin (see Fig. 10.2). However, the actual value of such reconstructed skin might be in the development of disease models to simulate specific situations (Semlin et al. 2011).
10.2.4 Artificial Skin Surrogates
Artificial skin surrogates have a long lasting history. Starting from simple membranes of dialysis tubing and polymeric membranes for example of regenerated cellulose, cellulose derivatives, polycarbonate, polyolefines, silicon, etc. more and more complex systems became available (Haigh and Smith 1994). It was recognized early that these very simple membranes do not resemble the skin characteristics and therefore their recommended use was to determine the release of active compounds only (FDA 1997). However, by loading of porous filter materials with lipids, these surrogates better resemble the skin properties especially if native SC lipids are used. Jaeckle et al. used systematic variation of lipid composition to establish suitable membranes which provide properties similar to heat-separated epidermis (Jaeckle et al. 2003). Recently, the Bouwstra group published different methods to establish SC model membranes (De Jager et al. 2004, 2005). For benzoic acid, they could nicely demonstrate that the flux is comparable to human SC (Groen et al. 2008). Based on the PAMPA technique (parallel artificial membrane permeability Assay) that has been successfully applied to predict gastrointestinal and blood–brain barrier absorption (Avdeef 2005) Ottavianai et al. reported on the use of a PAMPA-membrane loaded with an optimized mixture of silicone (70 %) and isopropyl myristate (30 %) to address skin permeation (Ottaviani et al. 2006). They could show for 31 substances of a diverse data set a good correlation to k p -values of human skin. Recently, two commercial models based on synthetic lipid mixtures, Strat M® (Joshi et al. 2012) and Skin-PAMPA (Sinkó et al. 2012), have become available.
The Skin-PAMPA assay mimics the lipid phase of the SC using an optimized lipid mixture composed of certramides, free fatty acids, and cholesterol. It can be considered as a refined approach of the method of Ottavani et al. mentioned above. The method was validated comparing the permeation (k p ) of various solutes through three different kinds of skin preparations (isolated SC, isolated epidermis, and full thickness skin). Moreover, it could be shown that the assay is capable to predict the permeation in human skin with a reasonable correlation between Skin-PAMPA permeability and Franz cell permeability (Sinkó et al. 2012). When it comes to mimic different skin preparation, the best correlation was found for full thickness skin. Since Skin-PAMPA is a rather new approach, it should be investigated further to prove its reliability and applicability to various scenarios.
The Strat M® approach uses a synthetic membrane sandwich composed of polyether sulfone treated with synthetic lipid materials to mimic the human skin. The method was tested using various substances in different formulations in comparison to human cadaver skin in a Franz cell setup. For aspirine, nicotine, and hydrocortisone, the usefulness of the model could be demonstrated showing comparable fluxes in comparison to human skin. Furthermore, comparable vehicle effects, such as penetration enhancement, could be observed for different caffeine formulations. The authors of the study state that the model was investigated against a diverse data set but unfortunately did not state exactly which compounds were tested and what kind of human skin preparation was used in the study (Joshi et al. 2012). Besides, a definition of the lipid mixture composition is missing. Since this is also a novel approach its reliability and versatility should be shown in greater detail in further studies.
10.3 In Vitro Experimental Setups for Skin Absorption Studies
10.3.1 Finite Versus Infinite Dosing
For skin absorption studies one differentiates usually between two different modes of application, finite and infinite dosing. Both terms are defined in the OECD Guideline 428 (OECD 2004b) and more specifically in OECD Guidance 28 (OECD 2004a). In general, finite dosing mimics the “in use” conditions where normally a limited dose of the formulation is applied to the skin. In contrast by infinite dosing a disproportionally high amount of formulation is applied. Typically a maximum of 1–5 mg/cm2 for powders, up to 10 μl/cm2 for solutions, and up to 10 mg/cm2 for semisolid preparations are considered finite doses. One problem with finite dosing is to spread the formulation evenly across the skin surface. Hahn et al. could demonstrate that this effect may influence the results of in vitro skin absorption studies depending on the applied drug (Hahn et al. 2012). Also, the finite dose kinetics of drug concentration over time are usually quite complex due to multifactorial overlapping processes such as the depletion of active in the donor phase (which should usually lead to a decrease in the rate of absorption), changes in the donor composition which affect the skin permeability, and elimination of the active due to metabolism and systemic clearance to name but the most relevant. The complexity of the problem shall be illustrated by the following example. If the formulation is applied without an occlusive dressing, the donor concentration may increase due to evaporation of components of the vehicle and consequently increase the rate of absorption. This may even lead to over-saturation or recrystallization of formulation components or the active on the skin which further complicates matters. Normally true finite dosing is assumed if the donor concentration depletes to at least 10 % of the initial concentration of the applied formulation after about 20–24 h (WHO 2006).
By infinite dosing, the amount of formulation should be large enough to assure that practically no donor depletion occurs. From that it follows that a maximum rate of absorption is gained and maintained over the whole experimental period. Typically the steady state flux is determined and based on that the apparent permeability coefficient is calculated. In between of finite and infinite dosing all transient cases are possible. For more details on the effects of finite, semifinite, and infinite dosing on skin invasion see Chapter 1 “Basic mathematics in skin permeation of drugs” in this volume.
As stated before finite dosing is normally related to “in use” conditions. However, the conditions during bathing or wearing closely fitting clothes are more realistic depicted by infinite dosing (WHO 2006).
10.3.2 Open Versus Occluded Dosing
Occlusion during in vitro skin absorption experiments will influence the results in different ways. Normally these effects are limited to the finite dose case, because for infinite dosing practically no changes of the donor compartment will take place. In the open system, due to evaporation of vehicle compounds, the concentration of active increases and may even achieve supersaturation. This changes the conditions completely depending on the magnitude of evaporation. Surber and Smith have reported this phenomenon as metamorphosis of the formulation (Surber and Smith 2005). On the other hand, if the active is volatile the concentration will decrease during experimental time. In this case, it is essential to determine the rate of evaporation during the experiment by collecting the volatile substance, for example, by using an adsorption trap (adsorptive powder) mounted on top of the diffusion cell (Rauma et al. 2013). This is essential if mass balance is addressed (Kasting and Miller 2006).
10.3.3 Diffusion Cells
In the past, many different protocols have been used to address skin invasion (ECETOC 1993; EC 2003a, b; SCCNFP 2003; SCCNFP/0690/03 2003; EC 2004; WHO 2006; SCCS/1358/10 2010; EFSA 2012). This results in problems of comparability of results. Meanwhile in 2004 the OECD Guideline 428 and OECD Guidance 28 became effective and provide general rules for conducting in vitro tests for dermal absorption (OECD 2004a, b). In principal special protocols are allowed; however, deviations from the recommended procedures should be restricted and have to be rationally motivated on a case-to-case basis.
In principal two different types of test chambers are available. These are either upright/vertical (Fig. 10.3a) or side-by-side/horizontal cells (Fig. 10.3b) with receptor volumes from 0.5 to 15 ml and application areas of about 0.2–2 cm2 (Brain et al. 1998). As by manufacturing processes dimensions may vary in certain limits calculation of data has to be related to individual cells. Vertical cells are preferable for testing semisolid formulations which can be spread on the incubation area analogously to the in vivo situation. In addition, it is easy to realize both occlusive effects by capping the donor chamber and in-use condition (nonoccluded) by leaving the donor chamber open. Usually, in vertical cells the receptor phase is mixed by stirring with a magnetic bar. Side-by-side cells consist of two chambers separated by a membrane. Both sides are stirred leading to highly controlled diffusion conditions, including continuous concentration equilibrium in both chambers and well-defined unstirred layers. Therefore, side-by-side diffusion cells are preferable for kinetic studies, especially if solutions are tested. For testing semisolid preparations, the design of side-by-side diffusion cells has to be changed by replacing the donor side by a static system without stirring (Feldmann and Maibach 1969; Bronaugh and Stewart 1984, 1985). For vertical as well as side-by-side diffusion cells, the static and the flow-through mode are available. Using the static mode the receptor phase is not replaced continuously except for the volume exchange during sampling. This leads to a constant increase of substance in the receptor fluid, and depending on the saturation concentration of compound diffusion may be affected. In general it is agreed upon that until reaching a maximum of 10 % saturation concentration in the acceptor, diffusion will not be influenced (known as sink conditions). Therefore, care must be taken not to exceed this limit. One possibility to overcome this problem is to exchange the receptor medium completely at each sampling point. However, it has to be considered that problems with compound quantification, due to quantification limits, and air bubbles may occur.


Fig. 10.3
Sketch of a static vertical Franz diffusion-cell (a), static side-by-side diffusion cell (b), after static side-by-side diffusion cell, flow-through cell (c), and Saarbruecken penetration model (d)
Because of the easy handling the most widely used static diffusion cell is the Franz diffusion cell which was introduced in 1975 (Franz 1975). As an alternative a flow-through version can be used (Fig. 10.3c). In this mode the receptor volume is continuously replaced by means of a pump, which more or less mimics the vivo conditions of drainage by the systemic circulation and facilitates maintaining sink conditions. A typical flow-through cell, based on a side-by-side diffusion cell, has been designed by Bronaugh and Steward (1985). It has to be kept in mind that flow rate, chamber volume, and detection limit are related variables. The flow rate has to be adjusted in a way that during the entire experimental time sink conditions apply in the receptor chamber, the amount can be detected, and proper mixing exists. In addition adsorption of analytes to the tubing used to pump the receptor medium may influence the results. A real benefit of flow-through systems especially for higher numbers of tests such as in an industrial setting is that continuous replacement of the receptor fluid can easily be automated (Moody 1997, 2000). Automation of sampling is available for vertical diffusion cells too, for example, described by Solich et al. (2001, 2003). In general several comparative studies have shown the equivalence of static and flow-through cells (Bronaugh and Maibach 1985; Bronaugh and Stewart 1985). Due to the easy handling and realization of in-use application conditions, static Franz diffusion cells are generally preferred. However, for studies where metabolic effects are involved flow-through systems like the Bronaugh cell are superior because the continuous solvent replacement better mimics the in vivo situation (Bronaugh 2004a, b).
10.3.4 Barrier Integrity Check
Barrier integrity has to be checked prior or after in vitro skin invasion experiments. Common physical methods are measurement TEWL or transcutaneous resistance or alternatively the measurement of the permeation of a marker substance, mostly tritiated water. TEWL measurements are well established for in vivo studies. For in vitro experiments, it is strongly recommended to use a closed chamber measurement setup (e.g., Aquaflux AF200, Biox, London, UK; VapoMeter, Delfin Technologies, Kuopio, Finland). In contrast, Netzlaff et al. reported that devices using the open chamber principle (e.g., the Tewameter® TM 300, Courage-Khazaka Electronic, Koeln, Germany) are not sensitive enough to detect small defects of the skin which nonetheless influence skin permeation (Netzlaff et al. 2006a). However, it has to be kept in mind that TEWL threshold values that might indicate a violation of barrier integrity depend on the TEWL instrument used as well as the skin preparation and therefore no general limits can be provided (Elkeeb et al. 2010). Using tritiated water as a marker is also a well-established method although the exclusion criteria for damaged skin are nowadays under discussion. Too many samples may be rejected using a permeability coefficient of water of 2.5 x 10−3 cm/h as the upper limit to reject cells with damaged barrier (Wilkinson et al. 2004). Another problem is the use of tritiated water before doing the experiment. During the removal of the remaining tritiated water barrier damage may occur. Therefore, the use of tritiated water must be evaluated critically.
10.3.5 Temperature
Diffusion processes are temperature dependent and therefore in vitro permeation experiments should be carried out at the physiological temperature of the skin surface, i.e., 32 ± 1 °C (OECD 2004a, b). This should be maintained during the entire experimental duration. If diffusion cells with a water jacket are used in line configuration may cause a temperature gradient between the first cell and the last cell. To overcome this problem parallel connection of the cells is recommended. Other possibilities are to insert the cells in a water bath (in this case care must be taken that no water enters the cells) or a metal bloc or alternatively to store the cells in an oven with constant temperature (Schäfer-Korting et al. 2006).
10.3.6 Selection of Receptor Fluid
The selection of the right receptor fluid is essential for the results. The following points have to be considered: the receptor fluid should enable sufficient solubility of the active compound under investigation (see also Sect. 10.3.3 for explanation of sink conditions), allow unhindered partitioning of the active from the skin to the receptor fluid, and not disturb the barrier integrity of the skin. For water soluble compounds, normal saline or isotonic buffer systems of pH 7.4 are appropriate. To prevent microbial influences on skin integrity preservatives, preferentially sodium azide (0.05 % wt/V), should be added. To maintain viability of fresh skin cell culture medium is feasible. For lipophilic substances additives to enhance the solubility are needed (OECD 2004a). For example, for essential oils ethanol water mixtures of different concentrations may be used (Reichling et al. 2006). Furthermore, the addition of surfactants (Challapalli and Stinchcomb 2002; OECD 2004a), bovine serum albumin (Dal Pozzo et al. 1991), or cyclodextrines (Sclafani et al. 1995) are reported. In all these cases, the influence of the additives on permeability must be clarified, e.g., by testing different concentrations of a solubilizer. Besides, it has to be considered that the analytical procedure may be affected by the additives.
10.3.7 Duration of Exposure and Sampling Period
Exposure times of the skin with the formulation should always reflect in-use conditions and consequently will vary, e.g., for rinse-off products exposure time will only be a few minutes. However, the sampling time should cover at least a period of 24 h (OECD 2004b). For kinetic studies, e.g., determination of permeability coefficients under infinite conditions or lag time (i.e., the time to reach steady state of diffusion) an extension to 48 h may be needed. Even longer sampling times normally result in impairment of the skin barrier and freshness and the results are questionable. Moreover, where static cells are concerned a sufficiently large number of sampling points should be planned so that a representative number of data points are available especially during steady state (rule of thumb: preferentially at least five sampling points which are evenly spread out during steady state) to describe the permeation kinetics properly. Especially concentration changes between sampling points should be large enough to be detected by the analytical procedure.
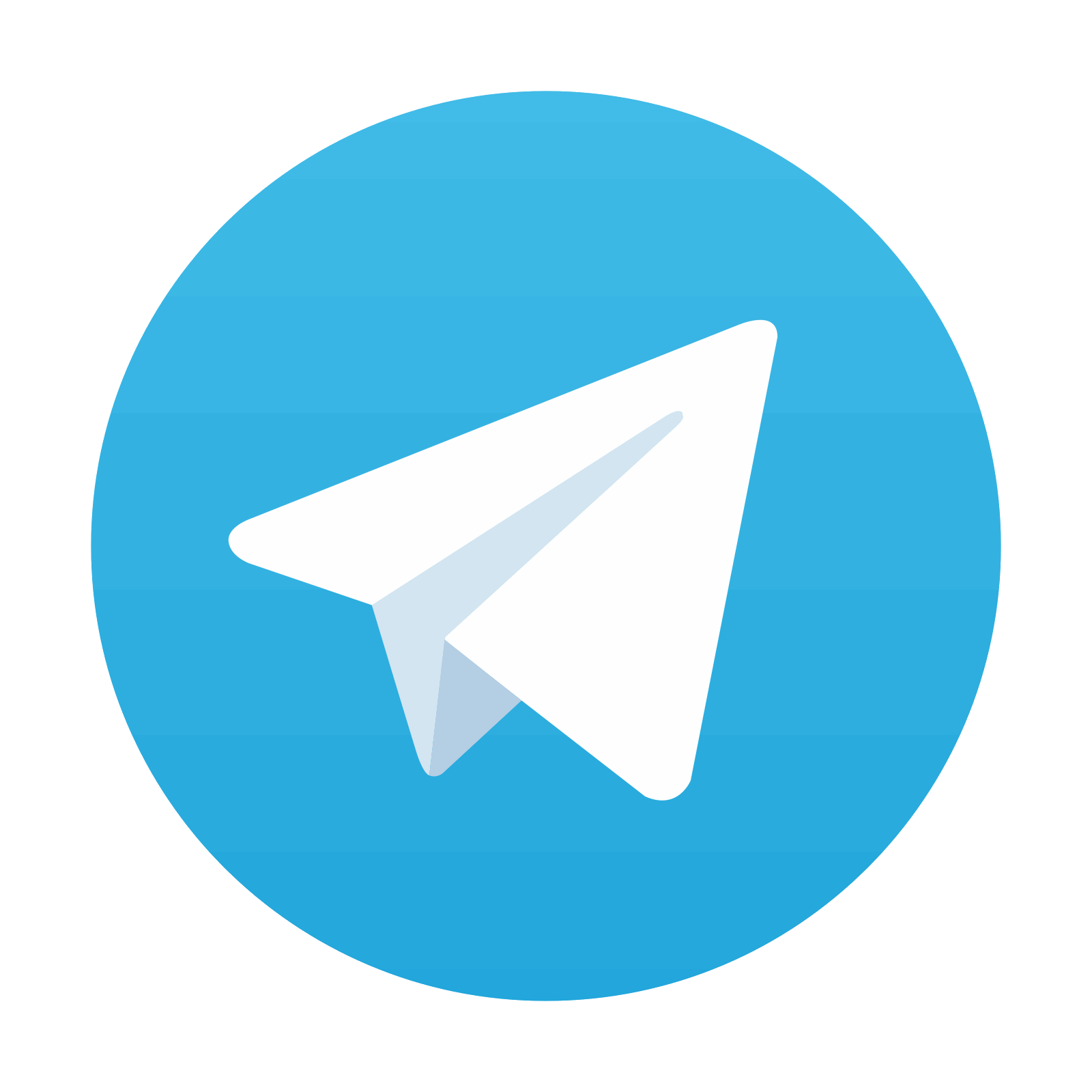
Stay updated, free articles. Join our Telegram channel

Full access? Get Clinical Tree
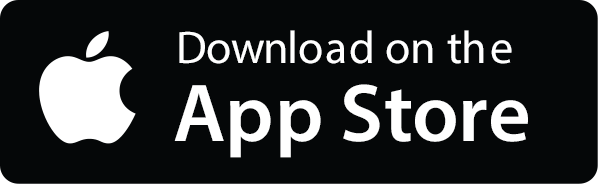
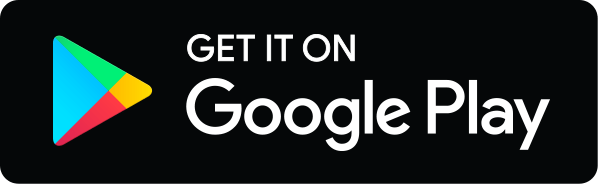