Chapter 23 Hematologic and hematopoietic response to burn injury
Access the complete reference list online at http://www.expertconsult.com
IN THIS CHAPTER
PowerPoint Presentation Online
Hematopoiesis
Stem cells
Hematopoiesis proceeds in a stepwise, hierarchical fashion.1,2 Hematopoietic stem cells (HSCs), which reside in the bone marrow, are at the top of the hematopoietic hierarchy. HSCs are able to both self-renew and differentiate, ensuring maintenance of their population and replenishment of all hematopoietic cell lines.3–6 There are two forms of HSC, long term (LT) and short term (ST), which differ in their self-renewal and differentiation capacities.7–9 As suggested by their name, LT-HSCs are the permanent self-renewing cells of the bone marrow with minimal response to physiologic stress, whereas ST-HSCs have the ability to increase their production and differentiation during different pathologic states. ST-HSCs differentiate into multipotent progenitor cells (MPPs).10 MPPs do not have the ability to reconstitute the bone marrow, but do maintain the ability to differentiate into all hematopoietic lineages, bridging the stem cell niche with progressively lineage-restricted progenitor cells.11,12 The hierarchy of hematopoiesis, from stem cell to progenitor groups to terminally differentiated cells (Fig. 23.1), allows for the rapid amplification of cell production by the upstream proliferation of stem cells.13 There are other suggested hematopoietic hierarchies14,15 which differ in the branch points at which certain progenitor populations lose lineage potential or suggest that some cell groups have the potential to dedifferentiate and enter a different lineage. In essence, hematopoiesis is controlled by a hierarchical pattern of progressive loss of lineage potential. The expression of a limited set of transcription factors, controlled by the growth factors and cytokines present in the bone marrow milieu, control lineage commitment, cell fate16,17 and, ultimately, the composition of cells in the bloodstream.
HSCs have received extensive attention in the media both because of their complicated ethical dilemma and also because of their extensive potential in treating blood disorders.18,19 The use of these cells as a potential therapy for the anemia or immune dysfunction present in severely burned patients is not currently feasible, but their use in the future is possible.
Progenitor cells
As HSCs differentiate they lose potency and developmental options, and become lineage-restricted progenitor cells. The genetic programs controlling lineage restriction take place over 10–15 cell divisions from the HSC stage. The traditional hematopoietic hierarchy proposes that HSCs differentiate into either common lymphoid progenitors (CLP) or common myeloid progenitors (CMP), based on local cytokine and growth factor levels2 and also on the development of the IL-7 receptor, which prompts lymphoid but not myeloid formation.20 CLPs can differentiate into T and B lymphocytes, natural killer cells and antigen-presenting dendritic cells.2,21–23 CMPs can differentiate into either megakaryocyte–erythroid progenitors (MEP) or granulocyte–monocyte progenitors (GMP). CMPs, MEPs and GMPs are identified by their unique expressions of the Fcγ receptor and CD34. As implied in their names, MEPs become either megakaryocytes or erythroid/red blood cells and GMPs become neutrophils, monocytes, macrophages, eosinophils, basophils and mast cells.24 Although progenitor cells are of the utmost importance in terms of lineage fate and the overall composition of the bloodstream, they comprise less than 1–2% of all bone marrow cells.
Burn injury, stem and progenitor cells
There has been very little research on the effect of burn injury on HSCs and progenitor cells. HSC production is amplified in a mouse model of sepsis, which is a similar physiologic process to burn injury.25 Inflammatory cytokines increase granulocytopoiesis while reducing lymphopoiesis via changes in common progenitor cells.26 Preliminary work from our laboratory has demonstrated an overall increase in the HSC population in mice initially following burn injury, with a gradual decrease 10–14 days post burn. Specifically, ST-HSCs and MPPs increase, but there is no significant change in LT-HSCs. Interestingly, in the progenitor compartment there is a significant increase in GMPs, with slight decreases in MEP, CMP, and CLP production following burn (Fig. 23.1). Most of these changes reverse themselves 2–3 weeks post burn. Given the hierarchical nature of hematopoiesis, changes in ST-HSC and MPP production as well as the lineage shift towards greater GMP production may herald the overall erythropoietic production (anemia) and myeloid function (immune dysfunction) problems present after burn injury. Further work on the HSC and progenitor cell response may provide avenues for early therapeutic intervention, which may ameliorate the negative and deleterious hematopoietic consequences of burn injury.
Red blood cells
Sources of anemia: clinical and laboratory findings
Burn patients commonly suffer from anemia. Anemia is a reduction in red blood cells below normal laboratory limits. Often, a mild reduction in red blood cells is of little clinical significance.27 Only when the red blood cell concentration is significantly reduced does it become clinically relevant, leading to impaired end-organ perfusion and oxygenation.28 Defined hemoglobin concentrations and hematocrit levels trigger the transfusion of packed red blood cells (pRBCs) in order to restore perfusion. In patients who are not actively bleeding, without coronary ischemia or without hemodynamic compromise, the transfusion trigger is typically in the range of 7–8 g/dL of hemoglobin or 21–24% hematocrit. Traditionally, a hemoglobin trigger of 10 g/dL or a hematocrit of 30% has been used. However, both large-scale work in the critical care literature29 and a smaller, retrospective review of both adult30 and pediatric31 burn patients have established the infectious, cost and survival benefit of a lower, more restrictive transfusion trigger.
Burn patients are anemic because of both acute surgical blood loss and the anemia of critical illness. Acute surgical blood loss stems from repeated debridements of the burn wound and wound coverage with autologous skin graft. For grafting to be successful, the wound bed must be prepared with well-vascularized tissue. Often, a clinically reliable gauge of the viability of this tissue is bleeding during debridement. As a result, much blood is lost during debridement, despite the wound eschar being devoid of vascularized tissue. Surgical blood loss has been estimated and measured in a variety of ways,32–37 but can most simply and reliably be reported based on surgical and anesthesia team estimates.38 In addition, owing to blood type and cross-matching expenses, and overestimation of operative transfusion needs,39 a preoperative estimate of 1.78 units of pRBCs per 1000 cm2 of burn wound excised is the best use of blood bank resources.40
Although acute surgical blood loss is obvious and most prominent during the treatment phase of burn injury, burn patients also suffer a prolonged anemia of critical illness during their recovery. The anemia of critical illness is the inability of red blood cell production to meet red blood cell demand and losses during a critical illness.41 Over 50% of all transfusions during a burn patient’s hospital course may be due to the anemia of critical illness.42 It has been equated to an acute form of the anemia of chronic disease.43,44 The etiology of the anemia of critical illness is multifactorial, with blood loss from iatrogenic factors such as phlebotomy and dressing changes,45,46 or erythropoiesis stunted by nutritional deficiencies,47 and bone marrow dysfunction. Specifically, bone marrow dysfunction leading to dampened erythropoiesis has been explored in autopsy studies and in the mouse model of burn injury and in vitro. In an autopsy study of patients who died from either myocardial infarction, sepsis or burns, the bone marrow of burn patients contained significantly fewer erythroblasts than that in the other groups.48 In the mouse model, both previous work from Wallner and recent work in our laboratory have demonstrated a shift in hematopoietic lineage commitment in the bone marrow away from erythroid cell formation at the expense of myeloid cell formation.49–51 Although Wallner’s work helped to establish this phenomenon, hierarchical models of hematopoiesis and the simple and reliable use of flow cytometry to identify rare populations of cells (as opposed to histologic and morphologic examination) have allowed us to track the source of these changes from modifications in HSCs with concomitant shifts in myeloid, erythroid, and lymphoid progenitor groups, as mentioned earlier. This insight into hematopoietic lineage commitment patterns following burn injury may not only help to better explain the dampened erythropoiesis of the anemia of critical illness, but may also shed light on a source for the dysfunctional leukocytes that contribute to immunomodulation. Further functional studies linking the changes of hematopoietic stem and progenitor cells with the cells present in the bloodstream may yield findings that could significantly benefit patient care in terms of anemia treatment/prevention and a better understanding of the origin of immunodysfunction.
Dampened erythropoiesis following burn injury may be caused by proinflammatory cytokines and/or elevated levels of catecholamines. Wallner suggested that there may be an ‘erythroid inhibitory substance’49 following burn injury, as the sera of burn patients reduce erythroid colony production compared to the sera of healthy patients.51 Although this work did not identify an ‘erythroid inhibitory substance’, later work on erythropoiesis has shown that both proinflammatory cytokines52–55 and catecholamines,56–60 which are both significantly elevated following burn injury, may inhibit erythropoiesis. In vitro, the addition of tumor necrosis factor,61,62 β-interferon,62 interferon-γ,63,64 and interleukin-6,65 all proinflammatory cytokines, reduces erythroid colony formation. Catecholamines are increased beyond their baseline state in the bone marrow following burn injury,66,67 and erythropoiesis is significantly altered with changes in catecholamine levels,68–70 which may contribute to the dampened erythropoiesis following burn injury. The prolonged proinflammatory and hypermetabolic state following burn injury may also significantly alter erythropoiesis and be a major contributor to the anemia of critical illness.
Clinical implications and treatment
Several methods have been developed to reduce acute surgical blood loss, including epinephrine tumescence, thrombin-soaked pads, and tourniquet use. Although the studies that examine epinephrine tumescence differ in their methods and results, the injection of dilute epinephrine into the subdermal space promotes vasoconstriction and reduces blood loss during surgical management of a wound.71–74 For example, in a prospective trial in children, epinephrine tumescence alone reduced blood loss from 3.5–5% to 0.98% of total blood volume/% of body excised and grafted.73 Epinephrine tumescence has no significant hemodynamic consequences, nor does it alter wound healing.75–77 The addition of thrombin-soaked pads for extra hemostasis following completion of the operative procedure has been studied in the context of epinephrine tumescence and provides additional hemostasis by reducing unnecessary ooze from the dressing site.33,78,79 Finally, the use of an extremity tourniquet during excision and debridement can reduce surgical blood loss without compromising graft adherence.80–83 The combination of all three techniques (epinephrine tumescence, tourniquets, and thrombin-soaked pads) can reduce intraoperative transfusion from 3.3 to 0.1 units per operative case, with 96% graft take33 and total units transfused from 15.7 to 7.9 units per patient.79
Recombinant human EPO helps to augment erythropoiesis in patients with chronic anemia (end-stage renal disease, HIV with antiretroviral use, non-myeloid malignancies with chemotherapy), reducing transfusion rates and improving quality of life. To reduce transfusion rates and correct the anemia of critical illness, multiple trials have explored rhEPO use in the critically ill, including burn patients. Unfortunately, both large clinical trials and meta-analyses of critically ill medical, surgical, and trauma patients show no significant reduction in transfusion rates with rhEPO use.84,85 Two studies of rhEPO have been performed in burn patients with no significant increase in hematocrit percentage or decrease in transfusion rates.86,87 Critically ill patients may possess a resistance to erythropoietin,88 which may be a function of antierythropoietin antibodies,89 or a relative reduction in erythropoietic response due to less EPO-responsive erythroid precursors secondary to burn-induced dampened erythropoiesis. Regardless of the cause, given the cost of rhEPO and the lack of transfusion reduction, rhEPO is not indicated for use in burn patients.
Ultimately, anemia is most easily corrected by the transfusion of pRBCs. Burn patients, as a result of both acute blood loss anemia and the anemia of critical illness, require a considerable number of transfusions during their hospital course. Transfusion rates increase with the size of the burn.39,90,91 In a large study of transfusion trends in burn patients, those with ≥ 20% TBSA required 13.7 ± 1.1 units, whereas those with ≥50% TBSA required >30 units of pRBCs.90 Although pRBC transfusion rapidly and reliably corrects anemia, blood transfusion is associated with a considerable number of consequences. The direct transmission of hepatitis B, hepatitis C and HIV are well-known consequences of transfusion, but their transmission rate has significantly decreased with better screening methods.92,93 More importantly, pRBC transfusion is associated with immunomodulation, including increased infectious morbidity,94 with a 13% increase in infections per unit of blood transfused.90 Other significant consequences include transfusion-related acute lung injury (TRALI), which is difficult to diagnose in burn patients as simultaneous lung injury due to resuscitation or inhalation injury may also contribute to the diagnostic criteria for TRALI;95 and ABO incompatibility, which can be rapidly fatal.96 As mentioned earlier, the implementation of restrictive transfusion strategies in which pRBCs are transfused only for hemodynamic instability or at lower, but still safe, hemoglobin concentrations, have reduced overall transfusion rates while reducing infection rates and providing cost and survival benefits.30,31
White blood cells
The primary effectors of host defense are the main populations of leukocytes, lymphocytes, NK cells, polymorphonuclear cells (PMN), and monocytes/macrophages. A balanced activation of these cells is important for robust host defense. Inappropriate activation of these leukocytes, especially the PMN and macrophages, can lead to tissue destruction and systemic inflammation, with dire consequences for the burn patient. Overactivation of PMN and macrophages has been implicated in the development of adult respiratory distress syndrome (ARDS) and multiple organ failure.97–104
Early post-burn period
In the first 24–48 hours following burn, a leukocytosis, and more specifically a granulocytosis, is generally observed that is dependent on the size of the burn wound, with larger burns giving rise to a greater degree of leukocytosis.105 The initial increase in the white blood cell count (WBC) is attributable to three major factors: the acute plasma volume loss due to the burn, demargination of mature neutrophils from peripheral blood vessels, and the rapid release of bone marrow reserves. Bone marrow myeloid progenitors from post-burn day 1 mice have an altered phenotype compared to controls, reflecting the more rapid maturation needed to replenish reserves.106 Following this initial period of leukocytosis burn patients frequently develop leukopenia, which is related to trauma-induced failure of bone marrow hematopoiesis.107 Leukopenia is also frequently seen in burn patients whose wounds are treated with silver sulfadiazine, a common topical antimicrobial agent.108 The severity of the leukopenia that develops from drug toxicity is directly proportional to the amount of agent that is applied to the wound, and thus indirectly to the size of the burn wound itself. The mechanism underlying this drug-induced leukopenia is yet to be elucidated, though direct bone marrow and cellular toxicity may play a role. In general, leukopenia is self-limiting and does not require discontinuation of silver sulfadiazine treatment.
Leukopenia and granulocytopenia often persist in patients when severe bacterial infection is superimposed on burn injuries, especially those with Gram-negative sepsis.109–111 In the early 1970s, Newsome and Eurenius demonstrated in their rat model of thermal injury that the initial granulocytosis was due to demargination of PMN from the blood vessels and through accelerated release of bone marrow stores.112 This granulocytosis, however, is transient, and is followed by a robust bone marrow response to replenish granulocyte stores.113,114 If the burn injury is complicated by sepsis, granulocytopenia persists.115,116 Granulocytopenia under these conditions appears to be due to bone marrow failure.114,115,117,118 McEuen demonstrated a significant inhibition of granulocyte colony-forming units in the bone marrow of burn septic rats.117 In addition, serum from septic burn-injured animals retarded the granulocyte colony formation when added to bone marrow cells from normal non-septic animals.114 In septic animals and in burn patients with sepsis, the observed granulocytopenia is probably not due to lack of available colony-stimulating factor.
Myeloid cells
In addition to alterations in the kinetics of production and maturation, PMNs exhibit qualitative functional changes after burn injury in both animals and humans.119–122 Some of the documented functional changes include depressed chemotaxis, phagocytosis, and intracellular killing.123–130 For example, isolated neutrophils of burn patients were shown to be defective in intracellular killing of Pseudomonas aeruginosa, and the impairment in phagocytosis and intracellular killing are further exacerbated with sepsis.131,132 These alterations in the production and function of PMN provide a framework for understanding the contributing factors in the depressed immune status of severely burned patients.
Monocyte/macrophage function is also altered with severe burns, but unlike PMNs, where neutropenia is common, in severe burns and sepsis monocyte counts tend to increase in both animals and humans.50,109,133 Studies demonstrate marked monocytosis, with a dramatic increase (3–5-fold) associated with sepsis.133,134 The importance of the burn-induced monocytosis is emphasized by the observation that attenuation of monocytosis as well as blunted macrophage activation results in improved survival.135 Miller-Grazziano and Faist demonstrated that after trauma, including burns, monocytes/macrophages could be broken down into subsets that were either hyper- or hyporeactive.136,137 These cells may develop their phenotypic differences as a result of altered monocytopoiesis within the bone marrow itself, or as a result of local cytokine environments in the peripheral tissues. Ogle et al. have demonstrated that bone marrow-derived macrophages from thermally injured animals produce increased amounts of TNFα, IL-1, and PGE2 compared to macrophages from non-injured animals, suggesting that thermal injury can stimulate the development of functionally different monocytes within the bone marrow.138,139
Lymphoid cells
Patients with severe burns often suffer from suppressed cell-mediated immunity owing to impairments in T-cell function.140,141 Impairment in cell-mediated immunity has been implied by observations of delay in skin allograft rejection, suppression of the graft-versus-host response, and skin hypersensitivity reactions in burn patients.142–144 The various alterations that have been reported include an overall suppression of circulating T lymphocytes, decreased mitogenesis in response to mitogens, reduced response to antigenic stimulation or activation, and redistribution of T lymphocytes in peripheral blood and tissue compartments.141,145–147 T lymphocytes are divided into T-helper (Th) and T-suppressor populations based on expression of lymphokine profiles, cell surface receptors, and ability to affect the function of NK cells, T-cytotoxic, and B lymphocytes. In recent years strong evidence has emerged to support the hypothesis that burn injury-induced immunosuppression is partly mediated by a shift in lymphocyte subpopulations from Th to T-suppressor subtypes.140,142,143 Part of the initial response to a significant burn is the activation of Th cells as assayed by IL-2R, HLA-2R, and transferrin receptor markers.148,149 In vitro functional assays reveal that despite the initial activation of T cells, they were unable to respond to mitogenic and/or antigenic stimulation. The addition of IL-2 fails to reverse T-cell anergy and fails to relieve the suppression of IL-2R.150 In addition, thermal injury triggers the apoptotic pathway in T cells.151,152 The exact mechanisms that initiate T-lymphocyte apoptosis are still unclear. However, Fas ligand-, TNFα-, and IL-2-mediated pathways have been implicated in T-lymphocyte cell death.153,154 Lastly, macrophage activation products such as PGE2 and transforming growth factor-β (TGFβ) can also contribute to T-cell suppression.
B lymphocytes also undergo functional changes with a profound reduction in immunoglobulin levels following thermal injury. All classes of immunoglobulin are reduced, but IgG levels display the greatest reduction, with concentrations reaching as low as 300–400% mg/dL.155–158 This drop in immunoglobulin is rapid and early, and is attributed to plasma leakage, increased protein turnover, and decreased IgG synthesis by the B cells. The initial deficit in immunoglobulins is slowly replenished to near normal levels within 2 weeks.143 Major histocompatibility complex (MHC) glycoproteins are reduced after thermal injury. Non-specific B-cell activation by burn toxins and antigen-dependent mechanisms, activation by phagocytosis of non-specific antigen, may inhibit their ability to form antigen–MHC complexes.159 Additionally, the display of self-antigens by the B lymphocytes could contribute to antigen-dependent cell death in T lymphocytes and T-cell anergy.141,143 Previous work has shown that CD23 expression on activated B cells is abrogated in response to thermal injury.160 IL-7 levels rise during the first week after burn and could account for the decreased proliferative capacity of mature B lymphocytes. Taken together, these data indicate that the functional alterations in B cells induced by thermal injury could further exacerbate the immune suppression in these high-risk patients.
Megakaryocytes and disorders of coagulation
The megakaryocytic lineage stems from MEPs, which are derived from CMPs. Megakaryocytopoiesis leads, ultimately, to the formation of platelets. Once megakaryocyte progenitors proliferate and differentiate, mature megakaryocytes produce platelets from cytoplasmic fragmentation.161 Cytoplasmic fragmentation results in the formation of proplatelets through regulation by the transcription factor NF-E2 that occurs in the bloodstream. Approximately 1 × 1011 platelets are produced daily.161 Platelets function to maintain hemostasis through thrombus formation at sites of injury. Platelet dysfunction occurs due to diminished platelet supply (thrombocytopenia), inability to adhere to damaged tissue and form clots, or when thrombi are produced unnecessarily. Dysregulated coagulation occurs through altered platelet maturation and function, changes in the proteins of the coagulation cascade, and imbalances in the deposition and dissolution of peripheral clots. Because platelets and coagulation disorders are interrelated, pathologic processes associated with both will be discussed in this section.
Platelets
Thrombocytopenia requiring platelet transfusion is rare in burn patients. Often, platelet counts and function are stable unless there is an infectious or septic event. In that case, burn patients should be managed similarly to any critically ill patient with sepsis. In patients with ongoing and hemodynamically significant oozing from wound and donor sites in addition to coagulopathy, the administration of platelets and recombinant factor VIIa has been shown, in two case reports, to significantly improve hemostasis.162,163
The transfusion of platelets is not without complications. Platelets are transfused in an ABO-compatible fashion and immediate transfusion reactions are rare. However, platelets are stored at room temperature, allowing for higher rates of bacterial contamination than other blood products. Bacterial contamination of platelets occurs in 1 : 1000–1 : 3000 units, with one-sixth of these episodes resulting in a septic event.164 In a study of blood bank use by a burn unit, 15% of all admitted patients received platelets in some combination with either pRBCs or FFP.39 So, although platelet transfusion is not common in burn patients, it is necessary to limit its use, given the significant infectious consequences.
Disorders of coagulation
Disorders of coagulation often occur early after the burn injury. Burn patients may suffer thrombocytopenia, disseminated intravascular coagulation (DIC), or other coagulopathies.165–167 A marked reduction in circulating platelet levels during the acute phase of burn resuscitation is primarily due to the consumption of platelets during the formation of systemic microthrombi.168,169 Although local microthrombus formation at the site of injury helps maintain the integrity of the microvasculature surrounding the burn wound, generalized systemic microthrombus formation leads to reduced end-organ perfusion and finally multiple organ failure.168,170 In addition, dilutional effects of extensive fluid resuscitation may also contribute to a reduction in platelet counts. After the initial resuscitation, the thrombocytopenia that is observed during the first week after the thermal injury is believed to be due to the diminished half-lives of platelets in circulation.169 The thrombocytopenic phase is followed during the 2–3 weeks after the initial injury by a period of increased platelet production or bone marrow megakaryocytopoiesis that results in either a return to normal levels or to overt thrombocytosis.171 However, in some burn patients thrombocytopenia persists and is often considered a poor prognostic indicator.172 Among patients with critical burn injuries who develop intravascular hemolysis, an apparent thrombocytosis may be observed which could be due to inadvertent counting of fragmented red cells and red cell microvesicles as platelets in an automated counter.171 Therefore, in severe burns the clinician should be aware of the possibility of spurious platelet counts in the presence of intravascular hemolysis.
Typically, changes in coagulation are transient and resolve with resuscitation and resolution of the acute-phase response to injury.168 In some cases significant alterations in the concentrations of coagulation proteins following thermal injury may persist due to the severity of the burn, sepsis, or the continued inflammatory state during a critical illness. Normally, the interplay among antithrombotic, prothrombotic cellular interactions, and fibrinolytic processes within the vasculature intricately controls the homeostatic regulation of coagulation. Under steady-state physiologic conditions, the fluidity of the blood is maintained by morphological integrity of erythrocytes and the endothelial cells lining the blood vessels.173 Normal levels of prothrombotic and antithrombotic factors, which are well regulated and remain for the most part in their quiescent state, also help maintain the homeostatic balance. In burn patients, however, both the thrombotic and the fibrinolytic pathways are triggered in direct proportion to the extent of the injury.166,174,175 During the early resuscitation phase of a burn injury there is a general reduction in the levels of coagulation proteins.166 Dilutional effects of volume administration during this period and the loss of plasma proteins to the interstitium could partially explain the decrease in plasma concentrations of many coagulation proteins. Clinically significant coagulopathic complications are uncommon. For example, clinically significant DIC occurs in only 0.09% of burn patients.176 However, the development of a coagulopathy, especially DIC, is associated with a poor outcome.177,178 Unexpected spontaneous aggregation of platelets in the early period following a burn injury may be due to hyperfibrinogenemia and the interaction between fibrinogen and platelets179 and can lead to the accumulation of thrombi in the microcirculation, and may contribute to the development of end-organ failure and death.167,170,180 Elevated levels of fibrinogen are often present immediately following burn injury owing to the shift in hepatic protein synthesis to acute-phase proteins, including complement, interleukins, etc. in addition to fibrinogen.
Owing to the increase in these proteins and their consequences, a significant number of studies have looked at different treatments aimed at offsetting this hypercoagulable state or replacing depleted elements of the natural anticoagulant proteins. The use of drotrecogin-α/human recombinant protein C181 and antithrombin infusions175,182,183 has been studied and reported to be safe and beneficial. Antithrombin III (ATIII) is a serine protease inhibitor that regulates hemostasis and has been shown to have anti-inflammatory properties.184 ATIII is depleted after burn injuries, as both coagulation and fibrinolysis are activated simultaneously.183 Administration of exogenous antithrombin III to burn patients has been shown to be beneficial in reversing thrombogenicity and reducing mortality, and animal models have suggested a role for this agent in attenuating pulmonary inflammation.174,175,183,184 Still, the indication for their use has not been established in the burn population.
Thrombus formation may also be due to decreases in the anticoagulant proteins antithrombin, protein C, and protein S.166 When anticoagulant proteins, including antithrombin, do not return to normal levels, patients may have a longer hospital stay and worse mortality.185 During recovery from the burn injury, coagulopathies are less related to the burn injury and more likely due to critical care issues, including sepsis. In fact, there is rapid recovery of coagulation factors in the postoperative period of burn wound management, making frequent excision and grafting safe from a hemostatic standpoint.186
Fibrinolytic pathways are activated in burn injuries owing to the increased levels of tissue plasminogen activator protein.166 Consumption of antithrombotic proteins by activation of fibrinolytic activities predisposes burn patients to thrombosis. Contributing to the thrombotic state in severely injured burn patients are the release of tissue phospholipids and tissue factor, activation of complement cascade, tissue ischemia, and the presence of sepsis.166,168,172,187 As a result, patients are at increased risk for developing disseminated intravascular coagulation (DIC). DIC is characterized by activation of coagulation, intravascular fibrin formation and vascular thrombosis, resulting in organ hypoperfusion and failure. The consumption of coagulation and anticoagulation factors leads to small vessel thrombosis and simultaneous uncontrolled bleeding.170 Because of the high morbidity and mortality associated with episodes of DIC, a high index of suspicion needs to be maintained in this patient population when sepsis complicates the clinical course. Signs of impending DIC may include refractory shock out of proportion to apparent blood loss, bleeding from venepuncture and catheter sites, and urinary and gastrointestinal bleeding. Standard laboratory values in patients with suspected DIC include prolonged prothrombin time (PT) and activated partial thromboplastin time (APTT), increased levels of fibrinogen degradation products, decreased levels of antithrombin III, and decreased platelet counts and fibrinogen levels. Vigilance must be maintained for other thromboembolic complications in this population, such as deep vein thrombosis and pulmonary embolism. The treatment modalities recommended for patients who develop DIC are primarily supportive and geared toward maintaining hemostatic parameters with replacement therapy. In addition, patients receiving broad-spectrum antibiotic therapy or showing signs of hepatic failure should be carefully monitored for vitamin K-dependent coagulopathy. This must be corrected with vitamin K replacement, along with the necessary coagulation factor component therapy. Future efforts in the treatment of coagulopathies will focus on re-establishing the balance of coagulation and fibrinolysis.170
Growth factors and transcription factors
As described above, the hematopoietic hierarchy and lineage commitment patterns are a complex but well-preserved processes. The maintenance of stem and progenitor populations and the expansion of terminally differentiated cells are under the control of the growth factors and cytokines present in the bone marrow. Shifts in the concentration of growth factors and cytokines due to illness and injury allow hematopoietic lineage commitment patterns to change, resulting in increases or decreases in terminally differentiated cell populations (Table 23.1). Control of cell fate is most likely due to growth factor- and cytokine-induced transcription factors, as expression and blocking of these transcription factors control lineage commitment in the stem and progenitor cell populations.
Table 23.1 The roles of growth factors and cytokines in hematopoiesis
Growth factor or cytokine | Role in hematopoiesis |
---|---|
Stem cell factor | Essential for hematopoietic stem cell proliferation and differentiation Activates the c-kit receptor |
Flt-3 ligand | Enhances multipotent progenitor, early lymphoid, myeloid, natural killer and dendritic cell proliferation Activates the Flt-3/flk-2/CD135 receptor |
IL-3 | Plays a role in hematopoietic stem cell, myeloid and erythroid cell line expansion |
IL-6 | Increased production following burn injury and infection Essential for expansion of hematopoietic stem and progenitor cells |
G-CSF | Stimulates granulocyte proliferation in the bone marrow and augments immune activity of cells in blood Increased immediately following burn injury and in response to infection RhG-CSF use is not indicated for use in burn patients |
CSF-1 or M-CSF | Essential for monocyte and macrophage differentiation Increases the survival of monocytes and macrophages |
GM-CSF | Regulates the proliferation and differentiation of hematopoietic progenitors Enhances antigen presentation by DC and macrophages Prophylactic administration accelerates bacterial clearance and killing |
IL-7 | Necessary for both engagement into lymphoid lineage and maintenance and expansion of lymphoid cells |
Erythropoietin | Stimulates erythroid proliferation and prevents apoptosis to increase erythrocyte production May have a role in tissue protection via a related receptor rhEPO has not been shown to benefit burn patients |
Thrombopoietin | Enhances megakaryocyte proliferation and reduces apoptosis to increase platelet production May increase following burn injury and contribute to thrombocytosis Evolving role in stem and progenitor cell proliferation |
Stem and progenitor cell growth factors
Hematopoietic stem cell maintenance and expansion are controlled by growth factors/cytokines both unique to these cells and also having roles in other hematopoietic and non-hematopoietic processes. For example, stem cell factor/steel factor, produced by fibroblasts and endothelial cells,188 is a growth factor essential for hematopoietic stem cell self-renewal and differentiation by activation of the c-kit (CD117) receptor.189 Mutations or deletions of stem cell factor or the c-kit receptor in embryogenesis can lead to in utero death from impaired hematopoiesis.190,191 Additionally, stem cell factor plays a role in melanocyte development,192 spermatogenesis193 and gut motility.189,194 Other growth factors that play a dual role are thrombopoietin, which is essential to megakaryocyte development and granulocyte–macrophage colony-stimulating factor, and IL-3 which contributes to both myeloid and erythroid cell proliferation. IL-6, which is significantly increased immediately following burn and in a variety of inflammatory states, is a proinflammatory cytokine that also plays a role in the expansion of hematopoietic stem cells.
Over 30 growth factors may play a role in stem and progenitor cell maintenance and expansion.195
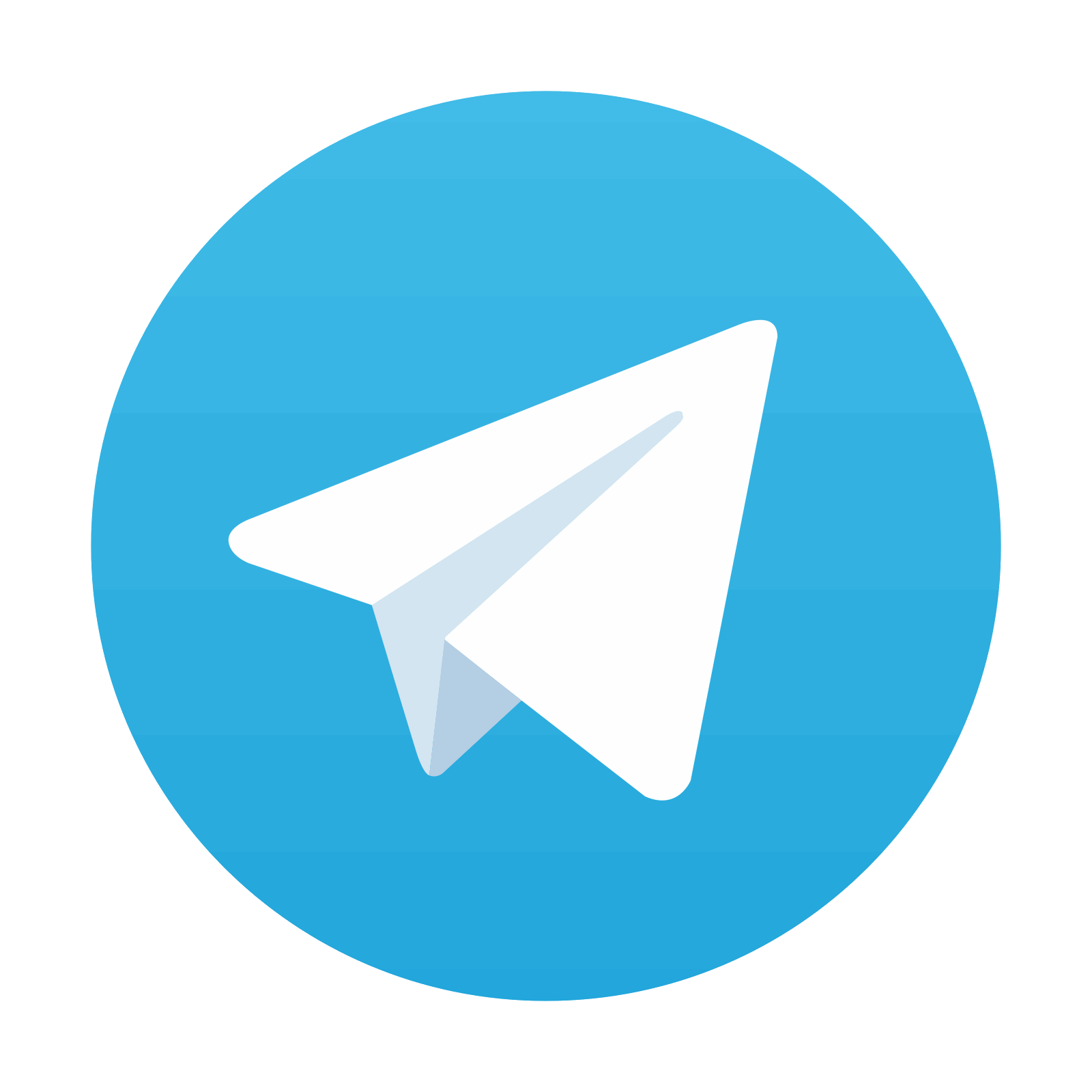
Stay updated, free articles. Join our Telegram channel

Full access? Get Clinical Tree
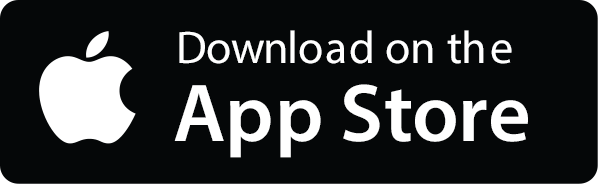
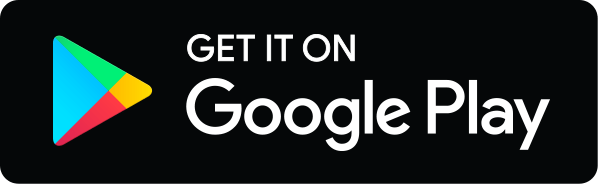