© Springer-Verlag Berlin Heidelberg 2015
Dédée F. Murrell (ed.)Blistering Diseases10.1007/978-3-662-45698-9_7676. Future Therapies for Epidermolysis Bullosa
(1)
Laboratory for Investigative Dermatology, The Rockefeller University, New York, NY, USA
(2)
Department of Dermatology, St George Hospital, University of New South Wales, Sydney, NSW, Australia
76.1 Introduction
76.2 Cell Therapy
76.2.1 Fibroblast Cell Therapy
76.4 Gene Therapy
76.6 Conclusion
76.1 Introduction
Epidermolysis bullosa (EB) constitutes a group of heritable blistering disorders manifesting with fragility of skin and mucous membranes with considerable morbidity and mortality [1]. In the past two decades, gene mutation analysis has revolutionized our understanding of the EB family of disease. A number of mutations in as many as 15 distinct genes encoding the protein components of the structural attachment complexes at the epidermal-dermal basement membrane zone have been demonstrated in EB [2]. Unfortunately, despite the great strides in genetic studies for EB, no definitive treatment for EB is currently available. However, very recently, a number of novel molecular strategies have been made toward EB treatment [3].
Mutations in COL7A1 encoding type VII collagen (which form anchoring fibrils) give rise to dystrophic EB (DEB), with the nature and position of the mutation in COL7A1 determining if the DEB is recessive or dominant [4–8]. Therefore, the therapeutic goal in DEB is to increase type VII collagen expression at the dermal-epidermal junction (DEJ) to enable the skin to withstand trauma-induced blistering [3]. To achieve this goal, various strategies are being pursued using cell-, stem cell-, gene- and protein-based approaches [2].
76.2 Cell Therapy
76.2.1 Fibroblast Cell Therapy
Keratinocytes and dermal fibroblasts both express collagen VII, but keratinocytes are the primary source of collagen VII in the developing skin [9, 10]. Many investigators have used keratinocytes and fibroblasts as the target cells of recessive dystrophic epidermolysis bullosa (RDEB) treatment [11]. Of note, intradermal injection of normal human fibroblasts or gene-corrected RDEB fibroblasts alone was found capable of synthesizing and secreting stable deposition of type VII collagen in mouse DEJ, thus contributing to the formation of anchoring fibrils [12, 13]. Also, fibroblasts are more robust and easier to propagate than keratinocytes, are less susceptible to growth arrest and differentiation, and can be frozen, packaged, and stored [14]. Therefore, the target cells for cell therapy have changed from keratinocytes to fibroblasts [11].
Compared with gene therapy, cell therapy has many advantages. The most important feature is that fibroblast cell therapy is safe and easy to work with [11]. In the experiments which have been performed with fibroblasts in mouse models and RDEB patients [12, 15–22], only minor side effects, such as temporary erythema, pruritus, mild hypertrophic scarring at the injection sites, and local skin inflammation, have been found in some cases. All of these side effects resolved spontaneously [15]. Fibroblasts can be used by intradermal injection into skin, and no special subsequent wound care is required. Furthermore, unlike stem cell transplantation, in which human leukocyte antigen (HLA) typing is important because the degree of HLA compatibility between donor and recipient will influence the outcome of the transplant, the donor of fibroblasts can be unrelated to the patient. Graft-versus-host disease did not occur when allogeneic fibroblasts were injected in the pilot study of five patients [15].
76.2.2 Clinical Trials of Fibroblast Cell Therapy
Among the current cell therapies for RDEB using skin fibroblasts are the use of allogeneic fibroblasts (cultured from the patients’ parents or an unrelated individual) or autologous fibroblasts (cultured from the patients themselves). Specifically, investigators evaluated the clinical benefits that may accrue from a single intradermal injection of these cells in subjects with RDEB. To assess potential clinical benefits in humans, Wong and colleagues [15] gave single intradermal injections of allogeneic fibroblasts to nonwounded skin on the backs of five subjects with collagen VII-positive RDEB. These investigators noted a 1.5- to 2-fold increase of type VII collagen at the DEJ at 2 weeks and at 3 months following injection and a 1.5-fold increase of anchoring fibrils. No significant immune reactions were observed in skin biopsies, and none of these patients developed autoantibodies to collagen VII.
Given the prolonged increase in type VII collagen following allogeneic fibroblast injection, a key question was whether the new collagen at the DEJ is derived directly from donor cells (“direct release”) or indirectly through enhanced synthesis of mutant type VII collagen by the recipient’s own keratinocytes and/or fibroblasts (“indirect release”) [3]. Molecular analysis was consistent with “indirect release” suggesting that the major effect of allogeneic fibroblasts is to increase the recipients’ own COL7A1 mRNA levels, with greater deposition of mutant type VII collagen at the DEJ and formation of additional rudimentary anchoring fibrils. Moreover, recent studies in one patient with RDEB identified heparin-binding EGF-like growth factor as a novel putative growth factor induced by fibroblast injections with the capacity to increase type VII collagen expression (predominately from the patient’s keratinocytes and therefore from mutant alleles) [23, 24].
With respect to wound healing in RDEB, however, a double-blinded randomized controlled trial (RCT) has shown no differences in the extent or rate or reepithelialization of chronic erosions following injections of fibroblasts or saline [25]. A recent phase II double-blinded RCT also has shown that the injection of both allogeneic fibroblasts and suspension solution alone improved wound healing in chronic nonhealing RDEB wounds independently of collagen VII regeneration [26]. On the other hand, McGrath and colleagues [27] reported that a single intradermal injection of allogeneic fibroblasts increased the initial rate of erosion healing in subjects with RDEB even though the effects were statistically significant only within the first 7 days. For now, the data and cumulative clinical experience indicate that for a subgroup of individuals with RDEB, notable those with mild-to-moderate disease severity and some baseline expression of type VII collagen at the DEJ, allogeneic fibroblast cell therapy may be useful [3]. For patients with more generalized disease, associated with extensive scarring and contractures and with the absence of type VII collagen protein at the DEJ, alternative cell therapy or other strategic approaches may be more effective [3].
76.3 Stem Cell Therapy for Epidermolysis Bullosa
76.3.1 Bone Marrow Stem Cell Therapy
Bone marrow is the major source of adult hematopoietic stem cells, which are capable of reconstituting the entire circulating population of hematopoietic cells [28]. The bone marrow also contains mesenchymal stem cells (MSCs), which contribute to the regeneration of mesenchymal tissues including bone, cartilage, muscle, and adipose tissues [29].
The rationale for bone marrow stem cell therapies is based on the capacity of bone marrow-derived cells to differentiate into skin cells given the right microenvironment [30–42]. The hypothesis is that chronic skin injury in RDEB generates a microenvironment that promotes homing of bone marrow-derived stem cells, which can then differentiate into skin cells, produce type VII collagen, and restore the anchoring fibrils defective in RDEB [28]. Chronic wounding, such as in epidermolysis bullosa, has been shown to stimulate the engraftment of bone marrow-derived stem cells to the skin and their incorporation and differentiation into non-hematopoietic skin structures [3, 42–44]. The early observations also support the usefulness of bone marrow stem cell populations in correcting basement membrane defects in RDEB [45].
Bone marrow cell therapy provides several advantages compared with other genetic and cellular therapies. Transplantation of wild-type allogeneic stem cells obviates the need for exogenous viral gene delivery and the risks associated with it, including insertional mutagenesis [46] and the eventual loss of gene expression. Furthermore, because bone marrow cell therapy can be delivered systemically via bone marrow transplantation, the therapy enables targeting of extensively affected skin as well as internal organs in RDEB.
76.3.2 Clinical Trials of Bone Marrow Stem Cell Therapy
Conget and colleagues [47] used intradermal injection of MSCs to treat RDEB in humans. MSCs were isolated from the bone marrow of healthy donors unrelated to patients and intradermally injected to two RDEB patients at chronic ulcerated sites. Reepithelialization of chronic ulcerated skin was observed near MSC administration sites, and the observed clinical benefit lasted for 4 months. One week after intervention, type VII collagen was detected along the basement membrane zone and the dermal-epidermal junction in MSC-treated skin.
To investigate the role of bone marrow MSCs for treatment of RDEB, El Darouti and colleagues [48] enrolled 14 RDEB patients in a clinical trial [28]. Bone marrow donor cells were harvested and cultured in vitro to isolate adherent cells for the transplant. To investigate potential additional benefits of cyclosporine treatment, 7 of 14 patients received cyclosporine infusion in addition to the stem cell transplant. Preliminary results demonstrated that blister eruption was significantly decreased in both treatment groups and rate of blister healing was significantly increased. No differences were detected between stem cell transplantation only and cyclosporine combination therapy.
Wagner and colleagues [49] reported the first clinical trial of allogeneic whole bone marrow. They enrolled seven children with RDEB on a trial of bone marrow transplantation consisting of myeloablative conditioning with busulfan, fludarabine, and cyclophosphamide, followed by the infusion of bone marrow. One of seven patients died of cardiomyopathy before transplantation. Of the remaining six patients, one had severe regimen-related cutaneous toxicity, with all having improved wound healing and a reduction in blister formation between 30 and 130 days after transplantation. New type VII collagen was noted at the DEJ in five of the six recipients, albeit without normalization of anchoring fibrils. The six recipients had substantial proportions of donor cells in the skin, and none had detectable anti-collagen VII antibodies. Clinical improvement was sustained for at least 1 year after bone marrow transplantation. A second clinical bone marrow transplantation trial has been initiated, using reduced-intensity chemotherapy before transplantation [28]. Along with demonstrating a potential curative therapy for RDEB, the study will examine a strategy of reduced-intensity conditioning prior to bone marrow transplantation, which may provide reduced risks and ethical concerns compared with myeloablative conditioning [50, 51].
76.4 Gene Therapy
76.4.1 Gene Therapy at the DNA Level
76.4.1.1 Ex Vivo Gene Transfer
The first successful gene transfer applied to RDEB was achieved by microinjecting a PAC vector into a COL7A1-deficient cell line [52]. This genomic approach has several advantages such as the presence of the endogenous regulatory elements for physiologic expression of the transgene and the maintenance of the genomic environment that can contain gene transcription [53]. However, gene therapy for RDEB has been hampered because of the inability of the large-sized COL7A1 cDNA (8.9 kb) to transfer. The recent progress in vectorology has permitted vectors to accommodate cDNAs as large as 10 kb, and several groups using different approaches have been able to correct primary human keratinocytes and/or fibroblasts derived from patients with RDEB.
The most efficient approach to achieve permanent transgene expression in epidermal stem cells is the ex vivo approach, where epidermal stem cells are harvested from the host by a skin biopsy and gene transfer is performed when the cells are growing in tissue culture. Genetically corrected cells are expanded, analyzed, and then grafted back onto the recipient. Several groups have reported successful COL7A1-cDNA transfer into keratinocyte cell lines, primary keratinocytes, or fibroblasts derived from a patient with RDEB using either nonviral gene transfer or virus-mediated gene transfer [12, 54–57]. In those studies, demonstration of functional correction was performed using grafting of the genetically corrected cells onto immunodeficient mice to show anchoring fibril formation in vivo in reconstructed human skin [53].
Mavilio and colleagues [58] reported successful treatment of junctional epidermolysis bullosa (JEB) with ex vivo gene therapy. Epidermal stem cells from an adult patient affected by LAM5- β[beta]3-deficient JEB were transduced with a retroviral vector expressing LAMB3 cDNA (encoding LAM5-β[beta]3) and used to prepare genetically corrected cultured epidermal grafts. Nine grafts were transplanted onto surgically prepared regions of the patient’s legs. Synthesis and proper assembly of normal levels of functional LAM5 were observed, together with the development of a firmly adherent epidermis that remained stable for 1 year.
76.4.1.2 In Vivo Gene Transfer
Woodley and colleagues [59] have shown functional correction after in vivo injection of a SIN-lentiviral vector expressing type VII collagen into RDEB skin equivalents grafted onto immunodeficient mice. This approach would avoid the need of culturing the cells, could permit treatment of areas not readily accessible, and theoretically would also offer a system treatment. However, in vivo injections of such vectors raise major safety concerns [53]. The viral vector that is injected in vivo in a well-vascularized tissue could disseminate into the body and affect other organs, where the effect of ectopic expression of type VII collagen is unknown, or could genetically modify germ line cells—an effect which is prohibited by the ethics committees [60].
76.4.2 Gene Therapy at the RNA Level
Classic gene therapy approaches for genetic disorders is based on the complementation of a functional deficiency via the transfer of the relevant wild-type nucleic acid sequence to cells and organs. A different methodological principle is to correct the endogenous genetic information itself to direct the biosynthesis of a functional protein product [53]. The exon skipping strategy is based on the capacity of small antisense sequences to mask signals recognized by the spliceosome machinery, thus leading to the excision of the target exon or exons carrying the mutation or mutations. If the target exon sequence is dispensable and the process maintains the open reading frame, it produces a shortened protein, which is partly or completely functional and which restores the phenotype.
Goto and colleagues [13] have shown the dispensability of exon 70 of COL7A1, by transducing RDEB keratinocytes and fibroblasts using a retroviral vector encoding a COL7A1 cDNA in which exon 70 was deleted. This group has also induced in vivo targeted skipping of exon 70 using antisense oligonucleotides in RDEB skin equivalents grafted onto nude mice, albeit with low efficacy. Although the efficacy of this approach for RDEB remains to be shown, it offers the opportunity of a noninvasive systemic in vivo treatment using easy-to-manufacture compounds (oligoribonucleotides). Drawbacks are that it can be used only on a subset of patients carrying mutations within exons that are dispensable for type VII collagen function, and it would require lifelong periodic administration.
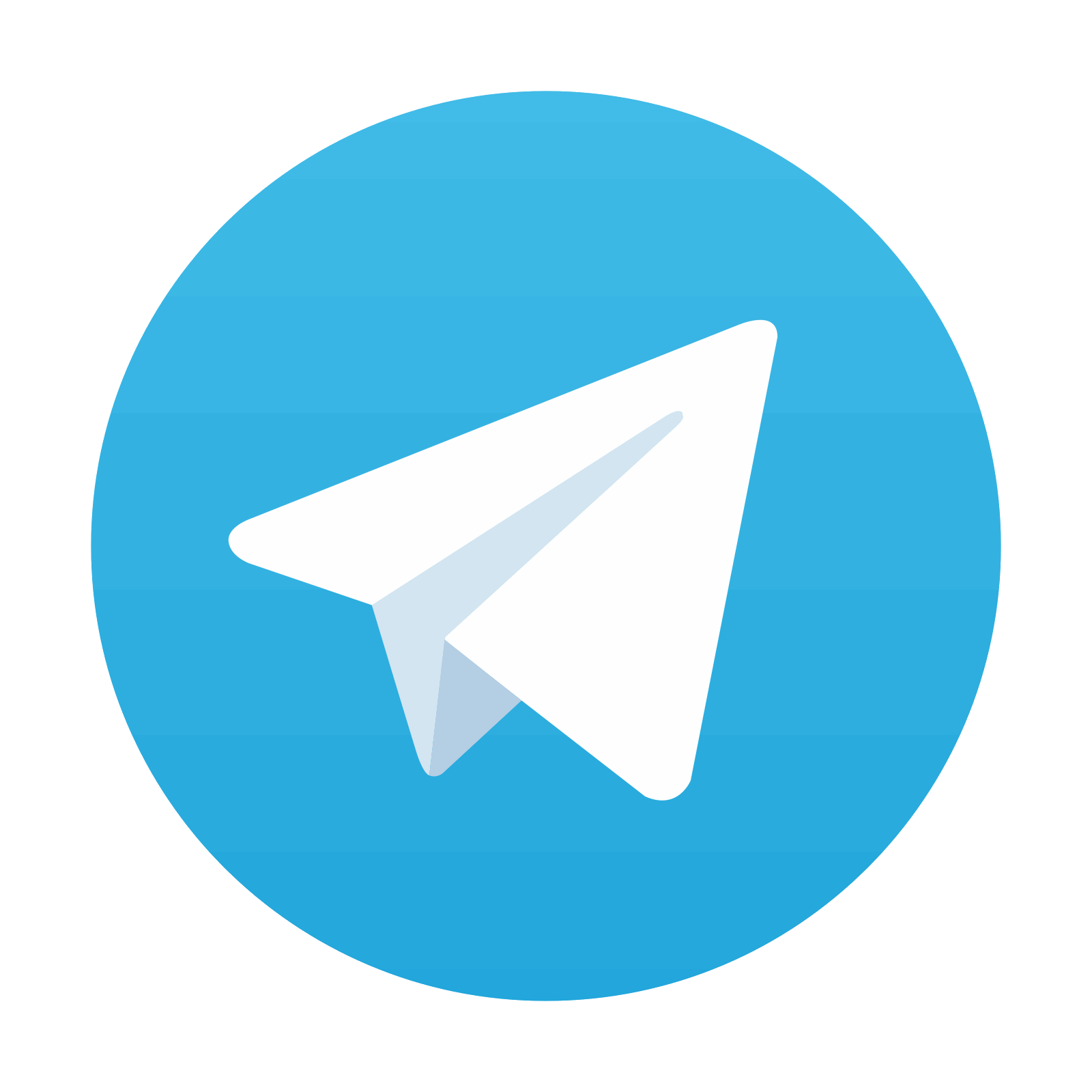
Stay updated, free articles. Join our Telegram channel

Full access? Get Clinical Tree
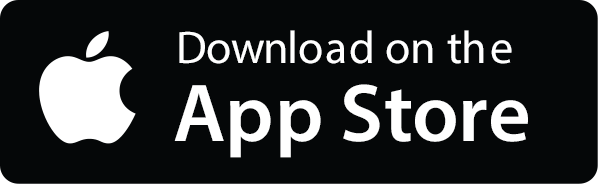
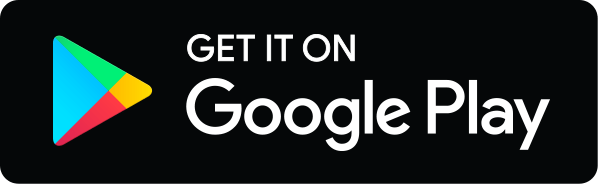