Dermal atrophy, manifesting as skin contraction, telangiectasia, and necrosis may be considered a consequence of decreased vascularization with loss of endothelial cells and reduction in capillary network. For the same reason the wound-healing ability may be severely compromised, which is of considerable clinical importance in the surgical field.
The pathogenesis of damage in irradiated tissue is not completely clear, but it is known that it primarily involves the cell as target and water, as a major constituent of the cell, plays a basic role in these events. The ionization of a molecule of water produces ROS (H+ and OH−) that have short lives and are very reactive [3]. Nowadays it is believed that indirect action of ROS in the context of tissues is the most important cause of injury than the direct action of ionizing radiation (e.g., on chromosomes). This action on the DNA causes tissue injury with endothelial cell damage resulting in inflammatory response, fibrin accumulation in extracellular matrix, and macrophage activation. The activated macrophages release several cytokines and ROS that perpetuate tissue damage. All these mechanisms contribute to the development of tissue ischemia which further stimulates production of ROS and cytokines [4].
Several groups have demonstrated that cytokines that are more prevalent in irradiated tissue are fibrogenic and proangiogenetic factors. In particular IL1 and TGF-β/Smad3 pathways have been implicated as mediators of fibrosis, and angiogenesis is stimulated via vascular endothelial growth factor (VEGF) production. Moreover to respond to proliferation induced by VEGF, endothelial cells die because of the accumulated radiation damage [5].
These changes are a common feature of response to a tissue injury, and inflammatory cells and activated macrophages are required to stimulate epidermal basal cells to proliferate and repair the damage. Conversely irradiated tissues show an intrinsic alteration in local stem cells that follow the principle of Bergonié and Tribondeau, previously described, and that is related to poor wound healing specific of radiodermatitis.
52.3 Preclinical Research
In recent years, autologous fat graft harvested with a syringe and processed according to the Coleman technique proved to be effective in treating skin alterations due to radiation. Several studies have demonstrated clinical improvement of chronic fibrosis adopting lipoaspirate transplantation, but the mechanism behind the improvement is not clear and number theories have been proposed [6]. Rigotti et al. [7] provided data supporting the hypothesis that the reported clinical results could be related with the observed presence of multipotent mesenchymal stem cells within the stromal vascular fraction.
The adipose-derived stem cells have shown to have multilineage potential and to differentiate in vitro into endothelial cells improving neovascularization [8]. These results have been confirmed in animal models. In mice autologous transplantation of stem cells in ischemic lesions determines tissue revascularization through neoangiogenesis, and using a murine model of radiation skin damage has demonstrated the clinical benefits and histologic effects of fat grafting on the skin and its vascular system [9].
Alternative mechanisms have been suggested to explain the clinical observation that fat grafting improves the quality of irradiated skin such as the adipocytes are able to secrete growth factors and cytokines, as vascular endothelial growth factor (VEGF), hepatocyte growth factor (HGF), insulin growth factor (IGF), and transforming-growth factor (TGF-β) with proangiogenetic effects, tissue remodeling, and antiapoptotic properties [8].
To better understand the molecular and biological mechanisms underlying this process and to translate these into clinical applications, further in vitro and in vivo studies are required.
52.4 Clinical Experience
Dystrophy of skin and subcutaneous tissues is a common clinical event in several congenital, genetic, and acquired diseases. Radiodermatitis is also found in patients affected by cancer since radiotherapy is essential for sterilizing any residual neoplastic site after surgery and in metastatic disease. Over 50 % of all cancer patients receive radiotherapy at one stage in their treatment process. Inevitably the skin is one of the most frequently damaged tissues due to its localization and constant turnover. The most affected cases are due to radiotherapy post mastectomy, radiation to treat arthritis of the joint, and adjuvant therapy in skin cancer and lymphomas.
Despite the lack of preclinical studies, the adoption of lipofilling in the sequelae of radiotherapy is an approach used for almost 10 years because clinical and experimental studies have demonstrated that grafting adipose cells in irradiated tissues improves the quality of the skin and enhances the thickness of the subcutaneous tissue [10].
Breast reconstruction after radiotherapy is an ongoing challenge for the plastic surgeon, and different approaches have been used. Autologous reconstruction with a pedicled or free flap, after adequate debridement of atrophic tissues, which carries distant nonirradiated skin to area of reconstruction allows good cosmetic results and lowers the complication rates better than implant reconstruction [11–13].
Unfortunately sometimes implant reconstruction may be the only option for patients who are not suitable for autologous reconstruction or who rejected this option. However implant reconstruction after radiotherapy results in to a high risk of failure, complication, and capsular contraction, and this approach does not improve the atrophy and deterioration of the skin due to radiodermatitis. A solution is to precede the implant coverage with multiple fat grafts in the irradiated area, improving the quality of the skin and reducing the complication related to this technique [14].
In the postmastectomy radiated chest, fat transfer may be done either before implant insertion or for treating a complication, as an ulcer or a capsular contraction, due to exposition of the prosthesis (Fig. 52.1
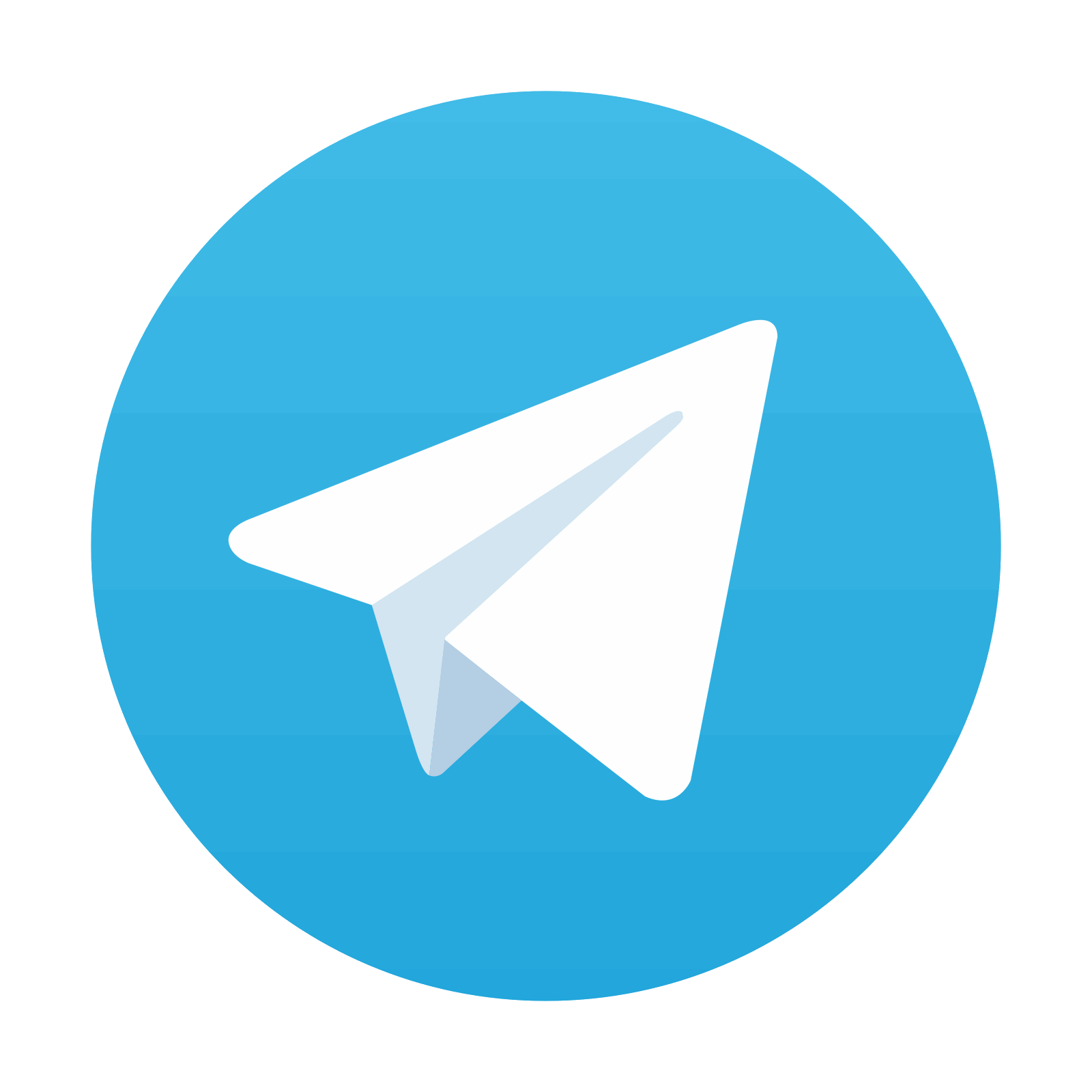
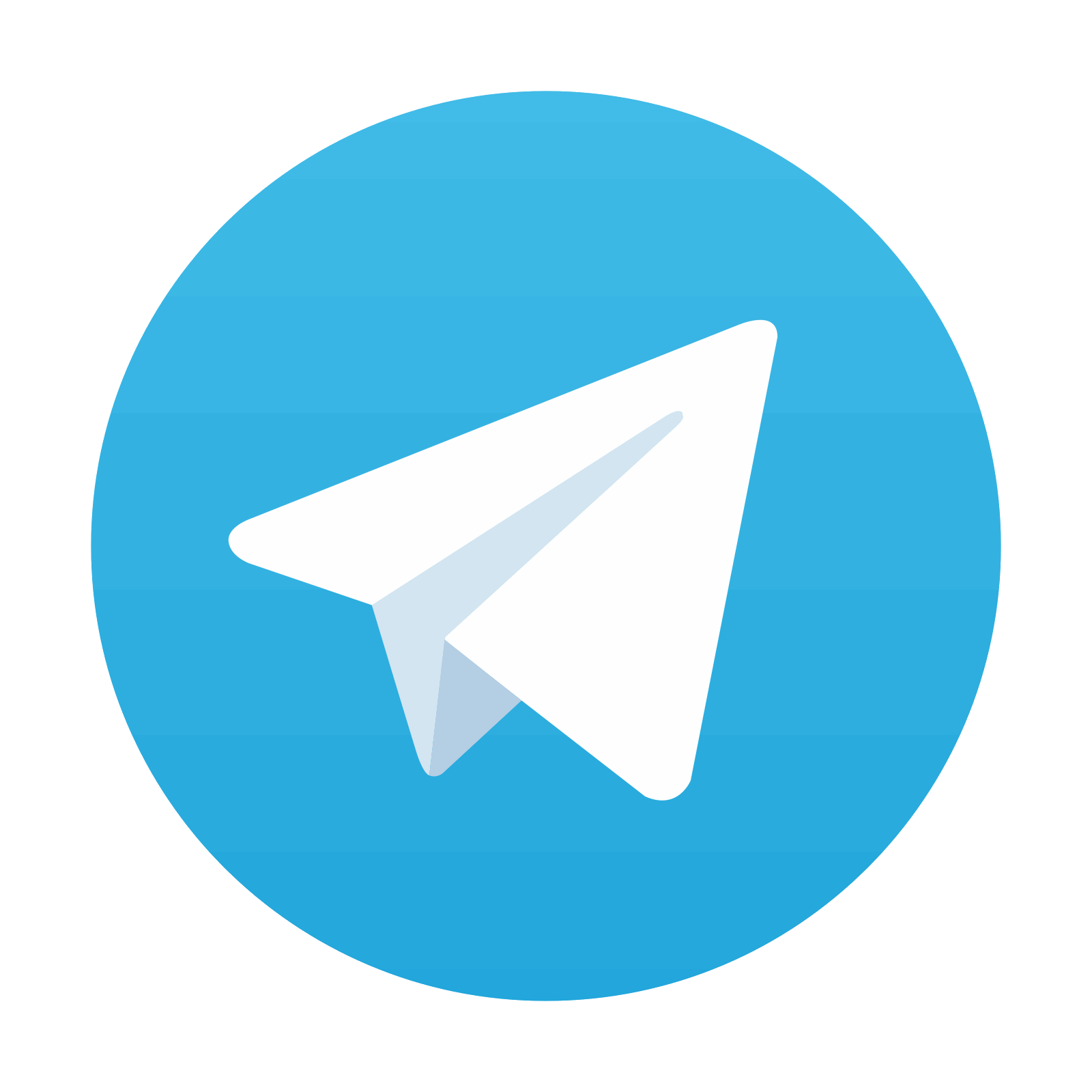
Stay updated, free articles. Join our Telegram channel

Full access? Get Clinical Tree
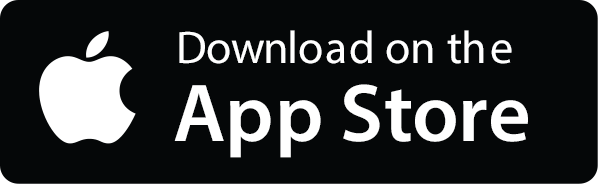
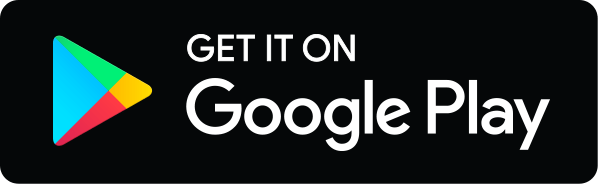