Chapter 9 Fluid resuscitation and early management
Access the complete reference list online at http://www.expertconsult.com
Introduction
Proper fluid management is critical to the survival of the victim of a major thermal injury. In the 1940s, hypovolemic shock or shock-induced renal failure was the leading cause of death after burn injury. Today, with our current knowledge of the massive fluid shifts and vascular changes that occur during burn shock, mortality related to burn-induced volume loss has decreased considerably. Although a vigorous approach to fluid therapy has ensued in the last 20 years and fewer deaths are occurring in the first 24–48 h post-burn, the fact remains that approximately 50% of the deaths occur within the first 10 days following burn injury from a multitude of causes, one of the most significant being inadequate fluid resuscitation therapy.1 Knowledge of fluid management following burn shock resuscitation is also important and is often overlooked in burn education.
Burn shock resuscitation
The history of burn resuscitation began over a century ago; however, complete appreciation of the severity of fluid loss in burns was not apparent until the enlightening studies of Frank P. Underhill,2 who studied the victims of the Rialto Theater fire in 1921. His concept that burn shock was due to intravascular fluid loss was further elucidated by Cope and Moore,3 who conducted studies on patients from the Coconut Grove disaster in 1942. They developed the concept of burn edema and introduced the body-weight burn budget formula for fluid resuscitation of burn patients. In 1952, Evans developed a burn surface area–weight formula for computing fluid replacement in burns which became the first simplified formula for fluid resuscitation for burn patients.1 Surgeons at the Brooke Army Medical Center modified the original Evans formula and this became the standard for the next 15 years.
A number of methods for accomplishing adequate volume replacement therapy have been advocated in the more than 40 years since the introduction of the Evans’ formula in 1952. This chapter will review the various methods advocated and present the rationale of each. Importantly, properly utilized, each resuscitation formula can be effective in the resuscitation of the burn patient in the immediate post-burn period, provided that close attention is paid to the individual’s clinical response to therapy and that fluid replacement therapy is modified according to this response. The fact that patients respond to a wide variety of resuscitative efforts is testimony to the fact that burn patients are very resilient and can be overwhelmed only under the most unfavorable circumstances.4
Pathophysiology of burn injury
Multiple mediators have been proposed to explain the changes in vascular permeability seen post-burn. The mediators can produce either an increase in vascular permeability or an increase in microvascular hydrostatic pressure.5,6 Most mediators act to increase permeability by altering membrane integrity in the venules. The early phase of burn edema formation, lasting for minutes to an hour, has been thought by some investigators to be the result of mediators, particularly histamine and bradykinin. Other mediators implicated in the changes in vascular permeability seen post-burn include vasoactive amines, products of platelet activation and the complement cascade, hormones, prostaglandins, and leukotrienes. Vasoactive substances are also released which may act primarily by increasing microvascular blood flow or vascular pressures, further accentuating the burn edema.7,8 Histamine is released in large quantities from mast cells in burned skin immediately after injury.9 Histamine has been clearly demonstrated to increase the leakage of fluid and protein from systemic micro vessels, its major effect being on venules in which an increase in the intracellular junction space is characteristically seen.10 However, the increase in serum histamine levels after burn is transient, peaking in the first several hours post-injury, indicating that histamine is only involved in the very early increase in permeability. The use of H1 receptor inhibitors, e.g. diphenhydramine, has only limited success in decreasing edema. Recently, the use of an H2 receptor antagonist has been reported to decrease burn edema in an animal model.11
Serotonin is released immediately post-burn as a result of platelet aggregation and acts directly to increase the pulmonary vascular resistance and indirectly to amplify the vasoconstrictive effect of norepinephrine, histamine, angiotensin II, and prostaglandin.12 The use of ketanserin, a specific serotonin antagonist, in a porcine burn shock model, improved cardiac index, decreased pulmonary pressure, and reduced arteriovenous oxygen content differences compared to a control group in the early post-burn period. Serotonin antagonists should be investigated further as possible adjuvant therapeutic agents during burn shock resuscitation.13 Prostaglandins, vasoactive products of arachidonic acid metabolism, have been reported to be released in burn tissue and to be at least in part responsible for burn edema. Although these substances do not directly alter vasopermeability, increased levels of vasodilator prostaglandins such as prostaglandin E2 (PGE2) and prostacyclin (PGI2) result in arterial dilatation in burned tissue, increased blood flow and intravascular hydrostatic pressure in the injured microcirculation, and thus accentuate the edema process. Concentrations of PGI2 and the vasoconstrictor thromboxane A2 (TXA2) have been demonstrated in burned tissue, burn blister fluid, lymph, and wound secretion.14,15 However, the use of prostaglandin inhibitors has produced variable results in animal studies. Arturson16 reported a decrease in burn lymph and protein flow with the use of prostaglandin inhibitor, indomethacin. Those results have not been corroborated by other investigators and the role of thromboxane and the prostaglandins still needs to be elucidated.
The activation of the proteolytic cascades, including those of coagulation, fibrinolysis, the kinins, and the complement system, has been demonstrated to occur immediately following thermal injury. Kinins, specifically bradykinins, are known to increase vascular permeability, primarily in the venule. Rocha and co-workers17 report increased kinin levels in burn edema fluid in the rat. The release of other mediators and the generalized inflammatory response after burns favors the activation of the kallikrein–kinin system, with the release of bradykinin into the circulation.18 Elevation of proteolytic activity has been demonstrated in both animals and in burn patients.19 Pretreatment with protease inhibitors significantly decreases free kinin levels but appears to have little effect on the edema process.
In addition to a loss of capillary integrity, thermal injury also causes changes at the cellular level. Baxter20 has demonstrated that in burns of >30% total body surface area (TBSA), there is a systemic decrease in cell transmembrane potential, involving non-thermally injured cells as well. This decrease in cell transmitting potential, as defined by the Nernst equation, results from an increase in intracellular sodium concentration. The cause of this is thought to be a decrease in sodium ATPase activity responsible for maintaining the intracellular–extracellular ionic gradient. Baxter further demonstrated that resuscitation only partially restores the membrane potential and intracellular sodium concentrations to normal levels, demonstrating that hypovolemia with its attendant ischemia is not totally responsible for the cellular swelling seen in burn shock. In fact, the membrane potential may not return to normal for many days post-burn despite adequate resuscitation. If resuscitation is inadequate, cell membrane potential progressively decreases, resulting ultimately in cellular death. This may be the final common denominator in burn shock during the resuscitation period.
Although the etiology of burn shock is not totally understood, many authors have studied the fluid volume shifts and hemodynamic changes that accompany burn shock. Early work by Moyer et al.,21 and Baxter and Shires22 established the definitive role of crystalloid solutions in burn resuscitation and delineated the fluid volume changes in the early post-burn period. Moyer’s original studies in 196521 demonstrated that burn edema sequestered enormous amounts of fluid, resulting in the hypovolemia of burn shock. In addition, he described the first crystalloid-only resuscitation formula used to treat burn shock. He noted that burn shock recovery occurred in the majority of patients studied, although hemoconcentration remained unchanged and the hematocrit was unresponsive to fluid administration despite adequate resuscitation. This became the first objective evidence that burn shock is not simply due to hypovolemia but is also influenced by extracellular sodium depletion. Baxter and Shires,22 in 1968, using radioisotope dilution techniques, defined the fluid volume changes of the post-burn period in relation to cardiac output. They first demonstrated that edema fluid in the burn wound is isotonic with respect to plasma fluid and contains protein in the same proportions as that found in blood. This confirmed Arturson’s earlier findings that in major burns there is complete disruption of the normal capillary barrier, with free exchange between plasma and extravascular extracellular compartments. They measured changes in fluid compartment volumes in burned primates and dogs and demonstrated that in untreated (unresuscitated) animals, a 30–50% extracellular fluid (ECF) defect persisted at 18 h post-burn. Plasma volume decreased 23%, to 27% below controls, although red cell mass changed only about 10% over the same 18-hour period. Thus, the greatest volume loss was functional intravascular extracellular fluid. Cardiac output was initially depressed very soon after injury to a level of about 25% of controls at 4 h after a 30% TBSA burn. By 18 h, however, the cardiac output had stabilized at around 40% of control, despite persistent defects in plasma and ECF volumes. On the basis of studies using different volumes of resuscitation fluids, they arrived at an optimal response in terms of cardiac output and restoration of ECF at the end of 24 h in a canine model. Clinical studies using similar sodium and fluid loads immediately followed, confirming efficacy in restoring ECF to within 10% of controls within 24 h. This became the basis for the Baxter or Parkland formula.20 Mortality was comparable to that obtained with a colloid-containing resuscitation formula.
Baxter went on to demonstrate that during the first 24 h post-burn, plasma volume changes were independent of the type of infused fluid, whether crystalloid or colloid, but at approximately 24 h post-injury, an infused amount of colloid would increase the plasma volume by the same amount. His findings prove that colloid-containing solutions are an unnecessary component of fluid resuscitation in the first 24 h. He recommended their use only after capillary integrity was restored, to correct the persistent plasma volume deficit of about 20% as measured externally. While the fluid shifts were being defined by Baxter in terms of crystalloid resuscitation, Pruitt and co-workers4 worked to characterize the hemodynamic alterations that occur in burn shock with and without fluid resuscitation. Their efforts culminated in the Brooke formula modification which utilized 2 cc/kg/% burn during the first 24 h. Fluid needs were initially estimated according to the modified Brooke formula, but the actual volume for resuscitation was based on clinical response. In their study, resuscitation permitted an average decrease of about 20% in both extracellular fluid and plasma volume, but no further loss accrued in the first 24 h. In the second 24 h post-burn, plasma volume restoration occurred with the administration of colloid. Blood volume, however, was only partially restored and an ongoing loss of 9% of the red cell mass per day was found. Cardiac output, initially quite low, rose over the first 18 h post-burn, despite plasma volume and blood volume defects. These results were quite consistent with those demonstrated by Baxter in his animal studies. Peripheral vascular resistance during the initial 24 h was initially very high but decreased as cardiac output improved, and in fact the changes were reciprocal. Once plasma volume and blood volume loss ceased, cardiac output rose to supranormal levels where it remained until healing or grafting occurred.
Moylan and associates23 in 1973, using a canine model, defined the relationships between fluid volumes, sodium concentration, and colloid in restoring cardiac output during the first 12 h post-injury. No significant colloid effect on cardiac output was noted in the first 12 h post-injury. In addition, 1 mEq of sodium was found to exert an effect on cardiac output equal to 13 times that of 1 mL of salt-free volume. This experiment established the fact that any combination of sodium and volume within the broad limits of the study would effectively resuscitate a thermally injured patient.
Arturson’s landmark studies in 197916 on vascular permeability characterized the nature of the ‘leaky capillary’ in the post-burn period. He demonstrated in a canine model that increased capillary permeability is found both locally and in non-burned tissue at distant sites when the TBSA burn exceeded 25%. He proposed that the burn wound is characterized by rapid edema formation due to dilatation of the resistance vessels (precapillary arterioles); increased extravascular osmotic activity, due to the products of thermal injury; and increased microvascular permeability to macromolecules. The increased permeability permits molecules of up to 350 000 molecular weight to escape from the microvasculature, a size which allows essentially all elements of the vascular space except red blood cells to escape from it. Further studies by Demling and co-workers24 have demonstrated that in 50% TBSA burns, one-half of the initial fluid resuscitation requirement may end up in non-thermally injured tissues.
Resuscitation from burn shock
It is quite clear that the edema process is accentuated by the resuscitation fluid. The magnitude of edema will be affected by the amount and type of fluid administered.25 The National Institutes of Health consensus summary on fluid resuscitation in 1978 was not in agreement in regard to a specific formula; however, there was consensus in regard to two major issues – the guidelines used during the resuscitation process and the type of fluid used. In regard to the guidelines, the consensus was to give the least amount of fluid necessary to maintain adequate organ perfusion. The volume infused should be continually titrated so as to avoid both under-resuscitation and over-resuscitation.26,27 As for the optimum type of fluid, there is no question that replacement of the extracellular salt lost into the burned tissue and into the cell is essential for successful resuscitation.19,21
Crystalloid resuscitation
Crystalloid, in particular lactated Ringer’s solution with a sodium concentration of 130 mEq/L, is the most popular resuscitation fluid currently utilized. Proponents of the use of crystalloid solution alone for resuscitation report that other solutions, specifically colloids, are no better and are certainly more expensive than crystalloid for maintaining intravascular volume following thermal injury.4 The most common reason given for not using colloids is that even large proteins leak from the capillary following thermal injury. However, capillaries in non-burned tissues do continue to sieve proteins, maintaining relatively normal protein permeability characteristics.
The quantity of crystalloid needed is in part dependent upon the parameters used to monitor resuscitation. If a urinary output of 0.5 cc/kg of body weight/hour is considered to indicate adequate perfusion, approximately 3 cc/kg/% burn will be needed in the first 24 h. If 1 cc/kg of body weight/hour of urine is deemed necessary, then of course considerably more fluid will be needed and in turn more edema will result. The Parkland formula recommends 4 cc/kg/% burn in the first 24 h, with one-half of that amount administered in the first 8 h9 (Table 9.1). The modified Brooke formula recommends beginning burn shock resuscitation at 2 cc/kg/% burn in the first 24 h (Table 9.1). In major burns, severe hypoproteinemia usually develops with these resuscitation regimens. The hypoproteinemia and interstitial protein depletion may result in more edema formation.
Hypertonic saline
Hypertonic salt solutions have been known for many years to be effective in treating burn shock.28,29 Rapid infusion produces serum hyperosmolarity and hypernatremia with two potentially positive effects.28,30 The hypertonic serum reduces the shift into the extracellular space of intravascular water. Proposed benefits include decreased tissue edema and fewer attendant complications, including escharotomies for vascular compromise or endotracheal intubation to protect the airway. Monafo28 reported that the resuscitation of burn patients with salt solution of 240–300 mEq/L resulted in less edema because of the smaller total fluid requirements than with lactated Ringer’s solution. Urine output was the indicator used during resuscitation. Demling and colleagues31 in an animal model demonstrated that the net fluid intake was less if burned animals were resuscitated with hypertonic saline to the same cardiac output compared with lactated Ringer’s. Urine output was much higher with hypertonic solution. Interestingly, soft tissue interstitial edema in burned and non-burned tissue, as reflected by lymph flow, was increased with hypertonic saline similar to that of lactated Ringer’s (LR). This can be explained by a shift of intracellular water into extracellular space as the result of the hyperosmolar solution. Extracellular edema can therefore occur at the same time as intracellular fluid defect. This may give the external appearance of less edema. Although several studies to date have reported that this intracellular water depletion does not appear to be deleterious, the issue remains controversial. Shimazaki et al.32 resuscitated 46 patients with either LR or hypertonic saline. The sodium infusions were equivalent, but the free water load was greater with LR; 50% of the latter required endotracheal intubation. The hypertonic serum also delivers a more concentrated ultrafiltrate within the kidney. This increases urine volume and salt clearance without marked increases in the required volume of free water.
There is no consensus regarding the type of osmolarity of hypertonic resuscitation fluid. Caldwell and Bowser,30 in 1979, reported a series of 37 patients with greater than 30% burns treated with either LR or hypertonic lactated saline (HLS), but no colloid. Total sodium balance was the same but the HLS group received 30% less free water and the reduced weight gain was maintained for 7 days. Subsequent reports from this institution reported successful HLS resuscitation in the elderly and children but no improvement in late mortality.33–35
Bartolani et al.36 randomized 40 patients to receive LR or HLS. HLS patients received more sodium, but less total fluid than the LR group. The observed higher mortality with HLS was attributed to larger burns in this group.
Reserve colloid for the second 24 h in cases where the patient remains poorly perfused after large infusions of crystalloid. Griswold et al.37 reported resuscitation of 47 patients with HLS resuscitation. Of these, 29 were also given colloid as albumin or fresh frozen plasma based on burn severity, premorbid state, or poor response to HLS resuscitation. This group had larger burns, greater mean age, and higher incidence of inhalation injury, but required only 57% of the fluid volume predicted by the Parkland formula, compared to 75% of predicted volume in the HLS alone group. Both groups maintained urine volumes of 1 mL/kg/h with no significant difference in hematocrit or serum sodium levels. Jelenko et al.38 also reported in a small series that patients given HLS and albumin required fewer escharotomies, fewer days of mechanical ventilation, and less total fluid than patients resuscitated with LR or HLS alone. Gunn et al.39 in a series of 51 randomized patients found no difference in fluid requirements or weight gain if they were given LR or hypertonic saline, if fresh frozen plasma was administered to maintain serum albumin levels above 2 g/dL, but all patients received hypotonic enteral feedings during resuscitation.
Yoshioka et al.40 reviewed 53 patients treated with greater than 30% burns resuscitated with LR, LR and colloid, or HLS. Fluid requirements were 4.8 mL/kg/% TBSA with LR, 3.3 mL/kg/% TBSA with LR and colloid, and 2.2 mL/kg/% TBSA with HLS. The total sodium requirements were increased 30% with LR compared to the other groups. Oxygen extraction, measured as A-VO2 difference, was improved with HLS, but reduced with LR–colloid, perhaps because of protein leak across the alveoli.
Vigorous administration of hypertonic saline solutions can produce a serum sodium above 160 mEq/dL or serum osmolarity greater than 340 mOsm/dL, followed by a rapid fall in urine output.41 Bowser-Wallace et al.33 and Crum et al.42 have reported 40–50% of patients treated with HLS developed hypernatremia with serum sodium greater than 160 mEq/L requiring switch to hypotonic fluids. Huang et al.43 reported a series of deaths associated with hypernatremia and hyperosmolarity following hypertonic saline resuscitation. Serial determinations of serum sodium and serum osmolarity are required to prevent complications including sudden anuria, brain shrinkage with tearing of intracranial vessels, or excessive brain swelling following rapid correction of serum hyperosmolarity. Current recommendations are that the serum sodium levels should not be allowed to exceed 160 mEq/dL during its use. Of interest, Gunn and associates,39 in a prospective randomized study of patients with 20% TBSA burns evaluating HSL versus LR solution, were not able to demonstrate decreased fluid requirements, improved nutritional tolerance, or decreased percent weight gain.
A modified hypertonic solution has been utilized in children with major thermal injuries >40% TBSA burn. The resuscitation fluid contains 180 mEq Na+ (lactated Ringer’s +50 mEq NaHCO3). The solution is utilized until the reversal of metabolic acidosis has occurred, usually by 8 h post-burn. The volume administered is begun at a rate calculated by the Parkland formula (4 cc/kg/% burn); however, volume is titrated to maintain urine output at 30–50 cc/h. After 8 h the resuscitation is completed utilizing LR to maintain urine output at 30–50 cc/h. This hypertonic formula can be used in infants and in the elderly without the accompanying risk of hypernatremia.44,45
Colloid resuscitation
Plasma proteins are extremely important in the circulation since they generate the inward oncotic force that counteracts the outward capillary hydrostatic force. Without protein, plasma volume could not be maintained and massive edema would result. Protein replacement was an important component of early formulas for burn management. The Evans formula, advocated in 1952, used 1 cc/kg of body weight/% burn each for colloid and LR over the first 24 h. The Brooke formula was clearly based on estimate rather than determined scientifically, but the formula used 0.5 /kg/% burn as colloid and 1.5 cc/kg/% burn as LR. The burn budget of Moore similarly used a substantial amount of colloids.3 Considerable confusion exists concerning the role of protein in a resuscitation formula. There are three schools of thought:
1 Protein solutions should not be given in the first 24 h because during this period they are no more effective than salt water in maintaining intravascular volume and they promote accumulation of lung water when edema fluid is being absorbed from the burn wound.46
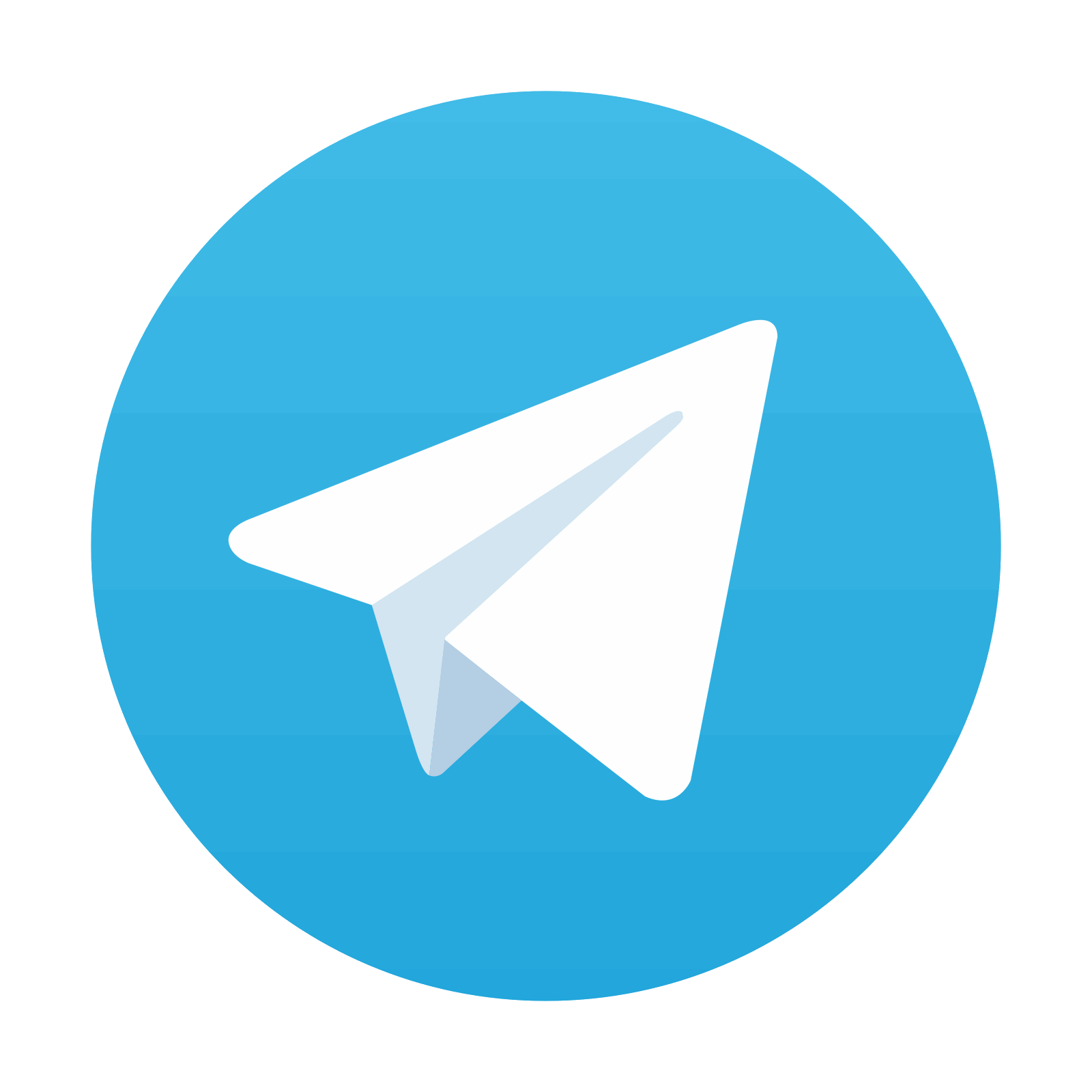
Stay updated, free articles. Join our Telegram channel

Full access? Get Clinical Tree
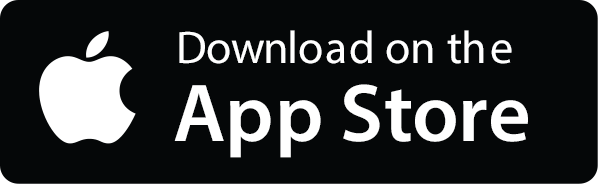
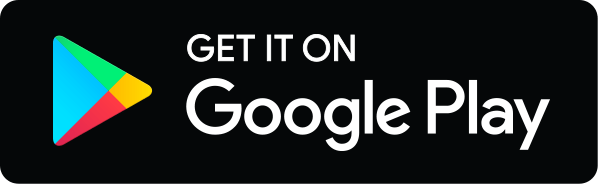
