A mastery of advancement flap design, selection, and execution greatly aids the surgeon in solving reconstructive dilemmas. Advancement flaps involve carefully planned incisions to most efficiently close a primary defect in a linear vector. Advancement flaps are subcategorized as unipedicle, bipedicle, V-to-Y, and Y-to-V flaps, each with their own advantages and disadvantages. When selecting and designing an advancement flap, the surgeon must account for primary and secondary movement to prevent distortion of important facial structural units and boundaries.
Key points
- •
A mastery of advancement flap design, selection, and execution greatly aids the surgeon in solving reconstructive dilemmas.
- •
Advancement flaps involve carefully planned incisions to most efficiently close a primary defect in a linear vector.
- •
Advancement flaps are subcategorized as unipedicle, bipedicle, V-to-Y, and Y-to-V flaps, each with their own advantages and disadvantages.
- •
When selecting and designing an advancement flap, the surgeon must account for primary and secondary movement to avoid distortion of important facial structural units and boundaries.
Definitions and core concepts
Local flaps, including advancement flaps, are useful for closing a wide range of skin defects in the head and neck. Although most wounds and primary defects in the head and neck region are a result of using micrographic surgical techniques (Mohs), removal of benign lesions and traumatic injuries can also result in defects that are too large to close primarily. The surgeon must consider secondary intention healing, skin grafting, or local flaps, to address the wound in these situations. The advantages of local flaps over secondary intention healing or skin grafting include superior match of skin color and texture along with minimal wound contraction. Many of the local cutaneous flaps in the head and neck include an advancement component. The simplest “advancement flap” is the linear closure of an incisional wound ( Fig. 1 ). Adjacent tissue is advanced from both sides to close the defect primarily. A wide range of advancement flaps that are variations on this basic technique are useful for closing defects throughout the head and neck. A mastery of advancement flap design, selection, and execution greatly aids the surgeon in solving reconstructive dilemmas.
Advancement flap design, like all local flaps, involves carefully planned incisions to most efficiently close a primary defect. Advancement flaps are defined as having a linear vector. The flap is incised, undermined, and “slides” forward to cover the primary defect. Because of this design, advancement flaps rely heavily on skin elasticity and its ability to stretch into the defect. By stretching and advancing local tissue in a linear vector into the defect, maximum wound tension exists along that linear vector at the distal border of the flap. The vector of wound tension is taken into account during flap design with attention to surrounding facial structures susceptible to distortion. Facial subunits and relaxed skin tension lines (RSTLs) are additional considerations.
When designing advancement flaps, one must anticipate 2 types of movement to achieve an optimal outcome that minimizes distortion of surrounding facial aesthetic structures. Primary movement refers to the “sliding” and/or advancement of tissue into the defect (see Fig. 1 ; Fig. 2 ). Secondary movement refers to displacement of surrounding skin directed toward the central defect from wound closure tension (see Figs. 1 and 2 ). Secondary movement can result in unfavorable distortion of mobile facial structures, such as the vermillion border, nostril, brow, eyelid, and ear lobule. Conversely, minimal secondary movement is seen along facial boundaries with strong underlying attachments. Undermining and selecting an appropriate site where maximum tension occurs help the surgeon favorably control secondary movement. Broad undermining around both the flap and the defect minimizes and distributes circumferential secondary movement. Locating maximum tension vectors next to tissue with a strong underlying bony attachment or the use of an “anchoring stitch” to secure the flap to periosteum adjacent to the distal cutaneous defect minimizes secondary movement.
Advancement flaps are subcategorized as unipedicle, bipedicle, V-to-Y, and Y-to-V flaps. Bipedical flaps can be further divided into T-plasty (O-T, A-T plasty) and H-plasty. Common to all these advancement flaps is that the advancing border of the flap represents the margin of the cutaneous defect. When using advancement flaps, surgeons must be conscious of the wound tension vector and place incision lines to lie along RSTLs whenever possible.
Definitions and core concepts
Local flaps, including advancement flaps, are useful for closing a wide range of skin defects in the head and neck. Although most wounds and primary defects in the head and neck region are a result of using micrographic surgical techniques (Mohs), removal of benign lesions and traumatic injuries can also result in defects that are too large to close primarily. The surgeon must consider secondary intention healing, skin grafting, or local flaps, to address the wound in these situations. The advantages of local flaps over secondary intention healing or skin grafting include superior match of skin color and texture along with minimal wound contraction. Many of the local cutaneous flaps in the head and neck include an advancement component. The simplest “advancement flap” is the linear closure of an incisional wound ( Fig. 1 ). Adjacent tissue is advanced from both sides to close the defect primarily. A wide range of advancement flaps that are variations on this basic technique are useful for closing defects throughout the head and neck. A mastery of advancement flap design, selection, and execution greatly aids the surgeon in solving reconstructive dilemmas.
Advancement flap design, like all local flaps, involves carefully planned incisions to most efficiently close a primary defect. Advancement flaps are defined as having a linear vector. The flap is incised, undermined, and “slides” forward to cover the primary defect. Because of this design, advancement flaps rely heavily on skin elasticity and its ability to stretch into the defect. By stretching and advancing local tissue in a linear vector into the defect, maximum wound tension exists along that linear vector at the distal border of the flap. The vector of wound tension is taken into account during flap design with attention to surrounding facial structures susceptible to distortion. Facial subunits and relaxed skin tension lines (RSTLs) are additional considerations.
When designing advancement flaps, one must anticipate 2 types of movement to achieve an optimal outcome that minimizes distortion of surrounding facial aesthetic structures. Primary movement refers to the “sliding” and/or advancement of tissue into the defect (see Fig. 1 ; Fig. 2 ). Secondary movement refers to displacement of surrounding skin directed toward the central defect from wound closure tension (see Figs. 1 and 2 ). Secondary movement can result in unfavorable distortion of mobile facial structures, such as the vermillion border, nostril, brow, eyelid, and ear lobule. Conversely, minimal secondary movement is seen along facial boundaries with strong underlying attachments. Undermining and selecting an appropriate site where maximum tension occurs help the surgeon favorably control secondary movement. Broad undermining around both the flap and the defect minimizes and distributes circumferential secondary movement. Locating maximum tension vectors next to tissue with a strong underlying bony attachment or the use of an “anchoring stitch” to secure the flap to periosteum adjacent to the distal cutaneous defect minimizes secondary movement.
Advancement flaps are subcategorized as unipedicle, bipedicle, V-to-Y, and Y-to-V flaps. Bipedical flaps can be further divided into T-plasty (O-T, A-T plasty) and H-plasty. Common to all these advancement flaps is that the advancing border of the flap represents the margin of the cutaneous defect. When using advancement flaps, surgeons must be conscious of the wound tension vector and place incision lines to lie along RSTLs whenever possible.
Anatomy and physiology: vascular supply
Understanding both the vascular supply and the process of neovascularization is essential for successful local cutaneous flap design. The vascular supply to the cutaneous skin serves 2 primary functions, nutrition and thermoregulation. The nutrition role is most pertinent to local flap design and survival. The 2 main sources of cutaneous circulation are the musculocutaneous and septocutaneous arteries. First, the musculocutaneous arteries pass through muscle into the cutaneous dermis to supply smaller skin regions. Second, septocutaneous arteries, also known as direct cutaneous arteries, follow a path through fascial septa that separate and divide muscle segments. These septocutaneous vascular networks typically run parallel to the cutaneous skin within the fascia below the subcutaneous fat and supply most of the nutritional and vascular support to the skin. Once the vascular supply reaches the cutaneous skin, these larger vessels empty into the complex system of interconnected dermal and subdermal plexuses.
Flaps are classified based on their arterial supply as arterial or random cutaneous flaps. Arterial cutaneous flaps incorporate a septocutaneous artery within the flap axis, offering greater survival and length compared with random cutaneous flaps. One example of an arterial cutaneous flap in the head and neck is the paramedian forehead flap. Conversely, advancement flaps are random cutaneous flaps. Random cutaneous flaps derive their vascular supply from the interconnecting dermal and subdermal plexuses originating from the flap base. Other local flaps, including rotation, transposition, and tubed flaps, also rely on this “random” but typically reliable blood supply.
Following local flap transfer, perfusion through the dermal and subdermal plexuses continues until neovascularization provides the blood supply and nourishment. Local flap perfusion from the base of the flap to its most distal tip is determined by the following: the intravascular resistance, or intrinsic properties of the supplying vessels, and the perfusion pressure. Distal perfusion pressure is the most important factor for flap viability on days 0 to 3 following flap transfer. Perfusion pressure decreases with increasing distance from the base of the flap secondary to distance traveled and stretch applied through advancement. Distally, there is ultimately a critical juncture where closing pressure of the arterioles within the subdermal plexus exceeds the perfusion pressure. At this juncture, the arterioles will collapse and perfusion ceases. A flap will be ischemic beyond this juncture. Arterial closing pressure is typically between 5 and 10 mm Hg. Early hypotheses of flap survival mandated that specific length-to-width ratios would ensure flap perfusion and viability. This thinking is flawed. Although wider-based flaps will capture more of the subdermal plexus that feeds the flap, the wider base will not increase distal perfusion pressure. Therefore, even designing a very widely based flap will not maintain distal perfusion pressure and viability in flaps of excessive length.
Although distal perfusion pressure is important at the beginning, neovascularization is essential for continued flap viability and flap integration with the tissue surrounding a defect. A complex interaction between vascular endothelial cells and stimulating angiogenic factors drives neovascularization that is typically complete by day 3 to 7 following flap transfer. During the first 48 hours after flap transfer, a fibrin layer forms between the adjacent tissues to provide a pathway for communication. The interaction of stimulating angiogenesis growth factors and vascular endothelial cells results in vessel dilation, increased permeability of the basement membrane and cell junctions, and finally, endothelial proliferation and sprouting. With continued growth, these capillary outgrowths form capillary loops that undergo rearrangement to form new capillary beds. Neovascularization then occurs through inosculation, the joining of capillaries with preexisting flap vessels, and direct ingrowth into recipient vessels. Both animal and human models have shown that neovascularization is complete by about 7 days and can typically stimulate growth from 2 to 5 mm in length.
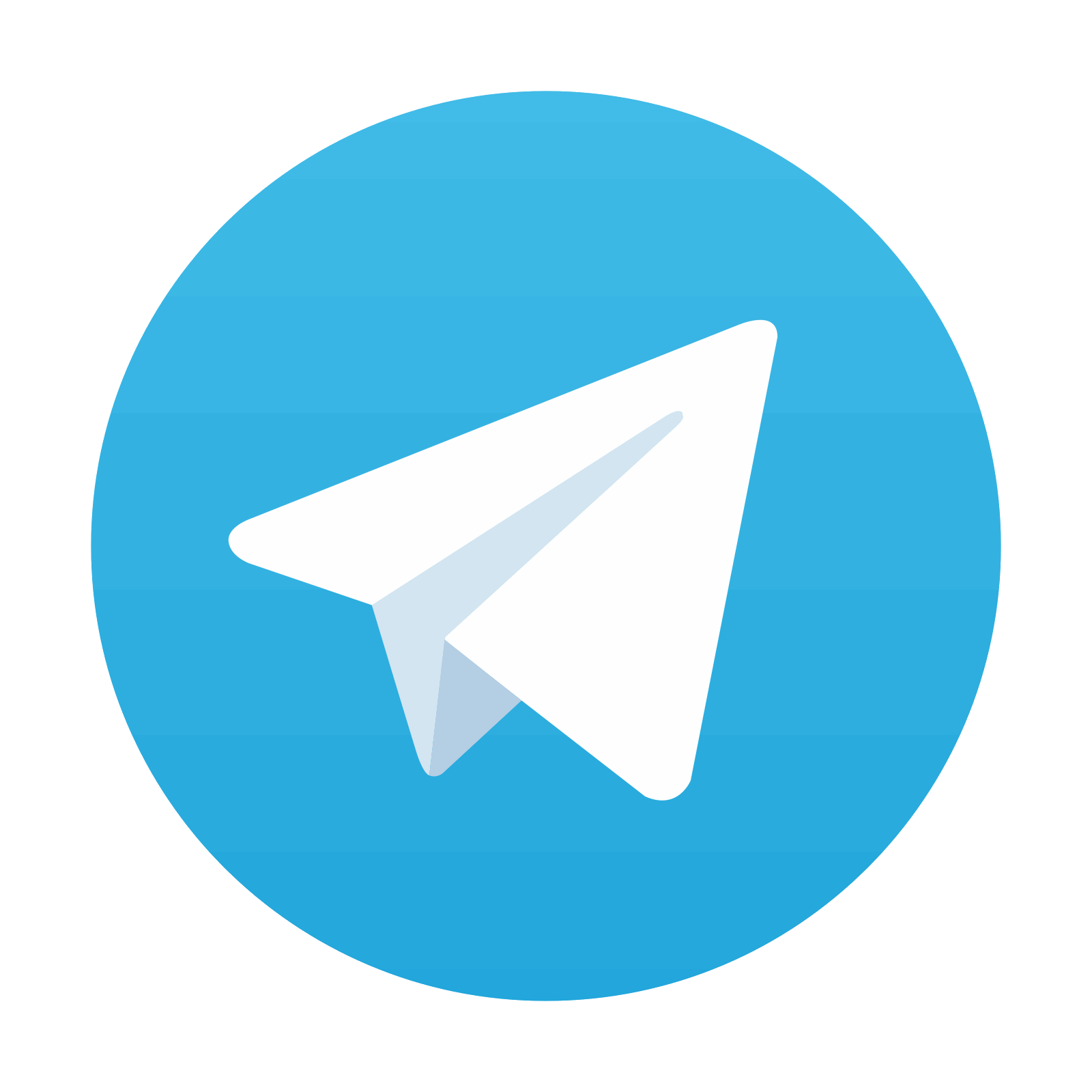
Stay updated, free articles. Join our Telegram channel

Full access? Get Clinical Tree
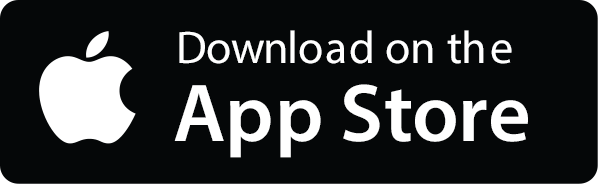
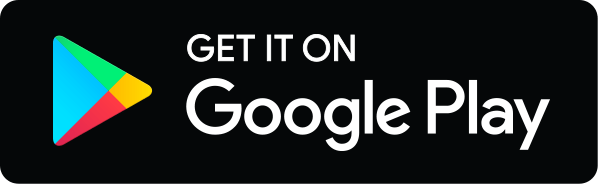