Fig. 57.1
Schematic depiction of epineural sleeve end-to-end anastomosis
Epineural tube is a naturally occurring conduit, which can be easily harvestable in large amounts from allogenic donor nerves in clinical setting. It has low immunogenic potential as it lacks Schwann cells, which are primarily responsible for nerve graft rejection [26, 27]. BMSC may provide an alternative source of easily accessible and expandable neural support cells as well as express neurotrophic factors supporting axon growth. In previous studies BMSCs were applied as a supportive therapy for tissue regeneration because of their plasticity. BMSCs comprise a multipotential heterogenous population of cells, which contribute to the regeneration of different types of tissues such as bone, cartilage, fat and muscle [28]. Recently, Chen et al. proved that BMSCs are capable of production of many trophic and supporting substances including neurotrophic factor NGF and laminin 2 [29]. BMSC are also immunotolerogenic properties that may ameliorate host inflammation [30]. On the contrary, Schwann cells have been shown to be highly immunoreactive and can only be used in isogenic transplantation [7, 26, 27]. They are also difficult to isolate and culture ex vivo to attain the correct phenotype and adequate amounts of cells. Therefore, BMSCs may provide a novel bio-responsive therapy free of the current restraints on Schwann cells.
Surgical Technique
Initial work was performed on well-established 20 mm rat sciatic nerve defect model. All studies were reviewed and approved by Cleveland Clinic Animal Research Committee and were carried in accordance to Guide for the Care and Use Laboratory Animals published by National Institutes of Health. In the isogenic model Lewis strain was both donor and recipient of epineural tube and BMSC [31]. In the allogenic model ACI strain was used as a donor of epineural tube and BMSC, while Lewis strain served as recipient. Additionally in mixed allogenic/isogenic construct Lewis BMSC were used in conjunction with allogenic conduit. In all presented studies autograft repair served as a control groups as it is widely accepted current gold standard when nerve grafting is required.
After adequate anesthesia and analgesia is obtained sciatic nerve is dissected circumferentially to isolate 20 mm segment. If longer epineural tube is needed the dissection can be extended proximally up to sciatic notch and distally to popliteal fossa obtaining 35–40 mm nerve segment. Then the nerve is transected proximally and distally and the free fragment is suspended on a straight 30G irrigator (Fig. 57.2a). Nerve fascicles are gently removed with the aid of a fine surgical forceps from proximal and distal end (Fig. 57.2b). This creates an empty epineural tube conduit ready for grafting (Fig. 57.2c).
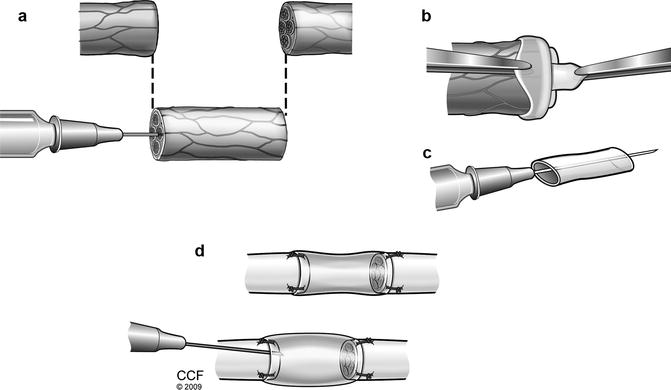
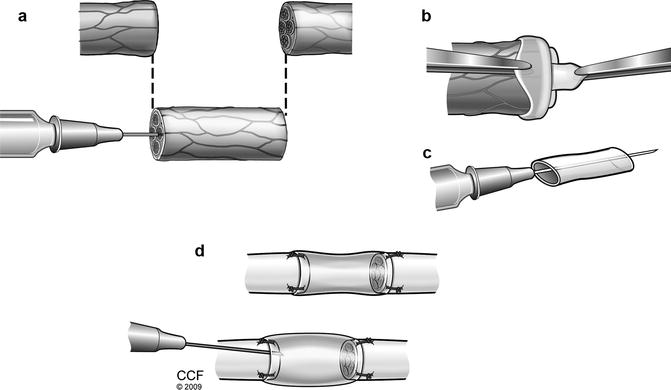
Fig. 57.2
Suspension of donor sciatic nerve on straight irrigator. (a) Removal of nerve fascicles from donor epineural tube. (b) Epineural tube conduit ready for coaptation. (c) Peripheral nerve repair with epineural conduit and injection of BMSC/saline (d)
The sciatic nerve of the recipient animal is exposed and defect is created. The resulting nerve gap is bridged by coaptating the epineural tube conduit to the proximal and distal nerve stumps of the recipient rat with standard epineural suture technique with 10–0 nylon under microscope. Then the conduit is filled with saline, BMSC suspension [31], fibrin gel with/without BMSC at the proximal end using a 27 g ½ (0.4 × 13 mm) tuberculin syringe. Approximately 0.1 ml of solution is delivered into 20 mm conduit, filling the entire conduit (Fig. 57.2d). In our protocol we typically deliver 2.5–3.0 × 106 isogenic or allogenic BMSCs into 20 mm conduit.
BMSCs are obtained from isogenic (Lewis) and allogenic (ACI) rats and purified as previously described [32]. Bone marrow is harvested from tibias and femurs of donor rats by flushing with culture medium. BMSC are isolated by multiple passages until the culture reached confluence [31]. Before transplantation stromal cells are labeled with red membrane dye PKH-26 (Sigma-Aldrich, UK), for their post-transplant assessment.
Methods of Assessment
Functional evaluation of the transplanted epineural tube conduits is performed by techniques previously established and validated in our laboratory [23–25, 31]. Functional assessment consists of toe-spread (Fig. 57.3) and pin-prick tests (Fig. 57.4), somatosensory evoked potentials (SSEP) (Fig. 57.5), gastrocnemius muscle index (GMI). The regenerated nerve samples and contralateral controls are also evaluated with histomorphometry and immunostaining [31].
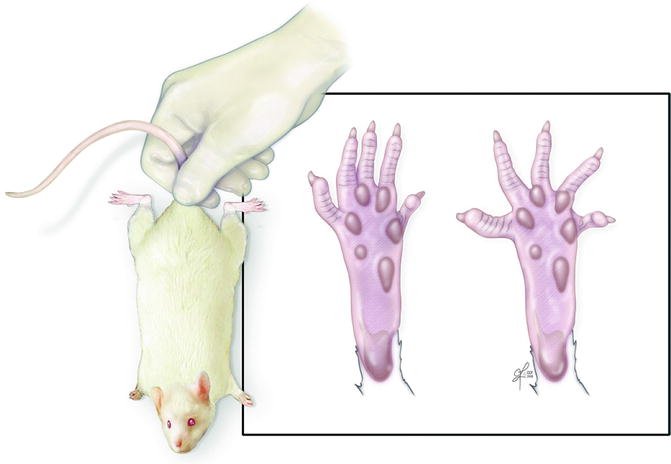
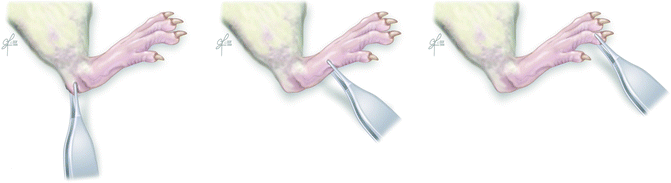
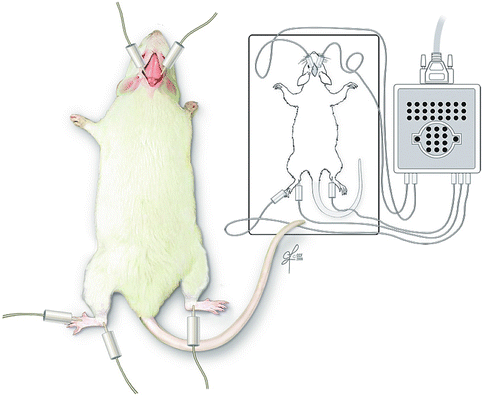
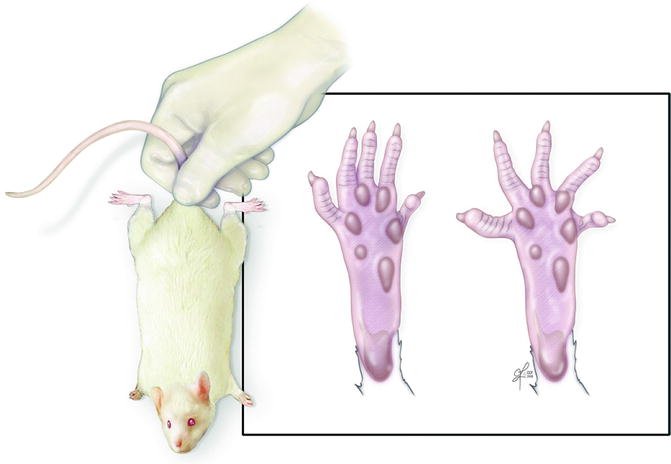
Fig. 57.3
Toe-spread test – rat is suspended by base of tail and degree of toe movement is graded from 0 (no movement) to 3 (abduction and extension)
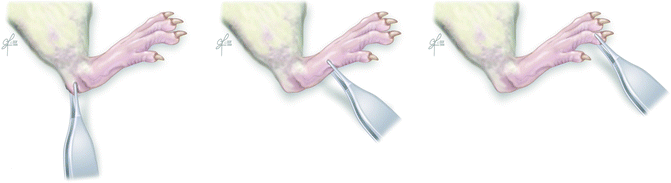
Fig. 57.4
Pinprick test – response to painful stimulus on the hindlimb of the rat. Demonstrated PP =1 withdrawal response at heel (left), PP =2 withdrawal response on dorsum of foot (middle), PP = 3 withdrawal response at toes (right)
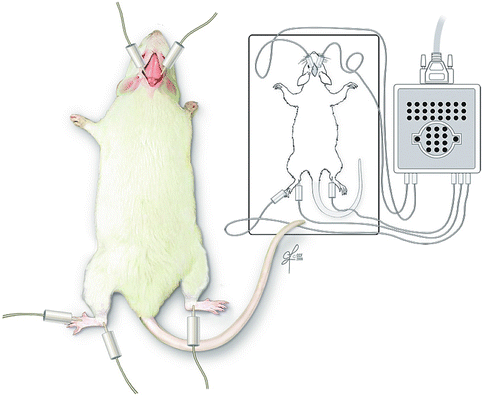
Fig. 57.5
Somatosensory evoked potentials recording. Stimulating electrodes placed in distal hindlimb. Signal is recorded from sensory cortex
Results of 20 mm nerve gap repair with isogenic epineural tube [31]. Pinprick and toe-spread scores did not differ between autograft repair and epineural tube groups (saline and BMSC filled) at 6 and 12 weeks. All animals had complete return of sensation at 12 weeks. SSEP evaluation did not reveal significant differences between autograft and epineural tube repair. However, there was a trend towards higher amplitudes at both time points and shorter latencies at 6 weeks when epineural tubes were filled with BMSC rather than saline alone. Histomorphometry did not reveal statistical differences between groups although autograft trended towards thicker myelin and larger nerve fibers at 6 weeks. Interestingly at 12 weeks when isogenic tube was supported with BMSC the regenerating nerve fibers had the thicker myelin compared to saline filled tubes and autograft. Epineural tube repaired sciatic nerves had larger nerve fibers then autograft. Autograft and BMSC supported conduit had similar percentage of myelinated nerve fibers while saline filled tube was the least myelinated graft. Saline filled epineural tube supported reinnervation of the gastrocnemius muscle better then BMSC supported conduit and autograft at 6-week time point, however there were no differences at 12 weeks post repair. In our model neurotrophic factors NGF and GFAP, extracellular matrix protein Laminin B, and proangiogenic factor VEGF were assessed. Autograft group revealed stable staining for all factors throughout the whole length of the graft at 6 and 12 weeks. In saline filled epineural tubes NGF and Laminin B expression was comparable to autograft at 6 weeks and decreased at 12 weeks. GFAP expression was the weakest in central part at 6 weeks and uniformly expressed throughout the tube at 12 weeks. There were no differences in the VEGF expression between proximal, medial, and distal parts in saline-filled epineural tube group, with higher staining intensity compared with autograft at 6 weeks and lower intensity at 12 weeks. In the group receiving isogenic BMSC, immunofluorescence showed the presence of PKH-26 positive cells in the proximal and medial parts of the transplanted epineural tube at 6 weeks and in all segments at 12 weeks. BMSC expressed NGF and GFAP as proven by double positive fluorescence. In summary, functional and histological outcomes of nerve gap repair with isogenic epineural tube supported with BMSC were comparable to autograft repair – the gold standard. We also confirmed that addition of BMSC to the conduit has a great potential to improve outcomes, which warrants further studies. However, nerve gap repair with isogenic epineural tube would not be clinically feasibly in most situations therefore, in the next step, we investigated the use of allogenic epineural tube supported with isogenic and allogenic BMSC. The same experimental model was used with the addition of 24-week observation group to assess long-term outcomes. It is worth reiterating that no immunosuppression was used at any time after or before the repair.
Results of 20 mm nerve gap repair with allogenic epineural tube.
This study comprised of control group – autograft repair, and allogenic epineural tube repair in three variants: saline, isogenic BMSC and allogenic BMSC filled conduit. In all animals the transplanted tube appeared to be intact and was bridging the nerve gap at the follow up. Macroscopically there was no evidence of rejection. We did not observe neuroma formation or axon growth outside the tube (Fig. 57.6). The pinprick test revealed no differences at 6 weeks and complete sensory recovery in all animal groups by 12 weeks. Although, animals that received saline filled conduit had worse motor exam at 6 weeks. BMSC supported constructs had comparable toe-spread scores to autograft repair at all time points (6, 12, 24 weeks). SSEP evaluation failed to reveal significant differences although fully allogenic constructed trended towards lowest amplitudes at 12 weeks and elongated latencies at 6 weeks. GMI failed to reveal any significant differences at the earliest time point. However 6 weeks later epineural tubes supported with BMSC, both allogenic and isogenic provided better muscle reinnervation than autograft and saline filled construct. At 24 weeks the mixed epineural conduit (allogenic tube/isogenic cells) had comparable GMI to autograft. Fully allogenic graft did not improve muscle reinnervation past 12 weeks. Laminin B2 expression was observed throughout the transplanted epineural construct up to 24 weeks post nerve repair. PKH-26 stained transplanted BMSC were observed inside the tube up to 24 weeks post nerve repair. Additionally BMSC expressed NGF as proven by double positive staining for PKH and NGF. Full histomorphometric evaluation is currently under way (Fig. 57.7). Presented results are superior to outcomes presented by Whitlock et al. in their study on collagen conduits and processed allografts in 14 mm sciatic nerve gap repair [33]. At 12 weeks post repair the Whitlock group reports wet muscle ratio 0.34 for processed allograft while Siemionow’s group reports ratio of ~0.57 for BMSC supported conduit in a 6 mm longer construct.
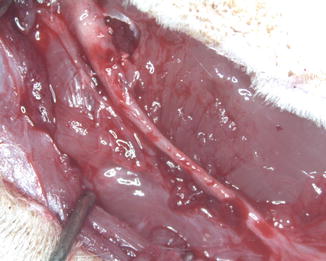
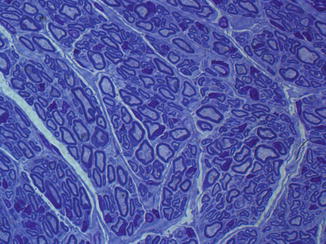
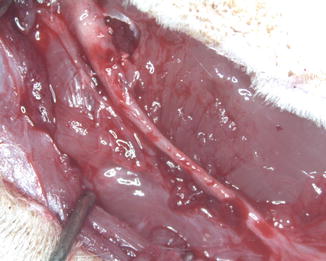
Fig. 57.6
Appearance of 20 mm sciatic nerve gap repair with allogenic epineural tube
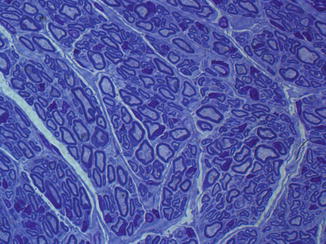
Fig. 57.7
Cross section through distal allogenic epineural tube filled with allogenic BMSC 12 weeks after transplantation. (Toluidine blue staining, 100×)
Large Animal Study
Currently Siemionow’s lab is investigating the concept of epineural tube repair on sheep model. Fully allogenic epineural tube repairs will be performed in standardized and previously used sheep 6 cm median nerve defect [34, 35]. Upon arrival to the animal facility, animals will be paired and will have their bone marrow harvested and expanded to obtain BMSC. Transplantation of epineural tubes will be performed between animals from the same pair as the harvested bone marrow to ensure same antigen load. Each animal will receive epineural tube in one forelimb median nerve defect whereas the other side will be repaired with autograft. Animals will be assessed (monthly) for nerve recovery with final endpoint at 6 months.
Preliminary Cadaveric Study
We also performed cadaveric study to prove the feasibility of obtaining epineural conduits from human nerves. Median and ulnar nerves were harvested. The first conduit was created by pulling out the fascicles one by one to achieve 1.5 cm of multi channel conduit. The next harvested fragment had the epineurium pulled inside out and fascicles were resected en-bloc, creating single channel tube. Both conduits were coaptated to the intact nerve fragments with four epineural sutures and filled with saline to test the mechanical properties of the conduit. Preparation of the conduits is fast (about 5 min for 3 cm single lumen conduit) and straightforward.
Discussion
Repair of major peripheral nerve injuries remains a great challenge despite recent developments in this field. It is important to remember that although the array of conduits, allogenic nerve grafts developed and researched is vast, the fact remains that to date, neither biologic nor synthetic graft material has been shown to be as efficacious as autologous nerve graft in nerve repair [36–40]. It became apparent that nerve diameter in addition to length of nerve defect is a critical determinant in regeneration success. Repair of larger nerves like ulnar nerve usually yields much poorer results than smaller nerves, which is likely related to the volume of the conduit that needs to be filled with cytokines, cells and extracellular matrix to promote axonal growth [41]. While nerve allografts showed to be efficacious in regeneration of large nerves, the need for immunosuppression (although temporary) and risk of rejection remains a significant concern [7]. Therefore, peripheral nerve allograft devoid of Schwann cells and antigenicity has been developed (Avance, AxoGen Inc.) and approved for clinical use in 2007. The manufacturing process removes all donor cells but retains the microstructure resembling native peripheral nerve, which provides scaffold for ingrowing axons.
Karabekmez et al. reported good outcomes after repair of sensory nerves in hands and fingers with Avance nerve graft in seven patients [42]. This study was followed by a larger multicenter trial, which included 76 nerve gaps (range 5–50 mm, average 22 ± 11 mm) repaired with this nerve graft [43]. Authors report an 87 % meaningful sensory and motor recovery based on the Mackinnon modification of the Medical Research Council grading system. However more recently Berrocal et al. published a case of failed 1.7 cm ulnar nerve gap repair with Avance graft [44]. Same disappointing results were reported previously when different type of conduits (PGA, collagen) were used to repair large diameter nerve gaps [41]. Most recently Squintani et al. reported good 2-year outcomes of 10 patients with 14 nerve gaps repaired with cryopreserved allografts without subsequent immunosuppression [45]. This technique was previously described on experimental models, which have proven that cryopreservation significantly decreases the antigenic load but also preserves the internal nerve microstructure [46, 47]. While the results of Squintani et al. study are encouraging, the relatively small sample size, retrospective design, variability in type of repair, timing of repair and site of injury would require larger well design study to completely evaluate this method. Therefore, currently for motor reconstruction, critical sensory reconstruction, and any large-diameter nerve reconstruction, autologous repair with graft or nerve transfer remains a treatment of choice [48].
In summary, the functional measures, clinical and electrophysiological, as well as muscle ratios confirmed that the results of epineural tube repair, both isogenic and allogenic are comparable to autograft repair in observation up to 12 weeks (24 weeks for allogenic group). Moreover in some instances epineural tube repair yielded better results than autograft, like at 6 weeks when isogenic tube repair promotes faster motor recovery as observed by tendency for higher GMI and better toe-spread scores. Those findings were also confirmed by a tendency to higher axonal density in the epineural tube groups compared with autograft at 6 weeks, and by significantly higher GMI in saline group compared with autograft. The addition of BMSC to the conduit resulted in thicker myelin and more myelinated nerve fibers. It is hoped that addition of cellular therapy to the epineural tube will extend this positive effect over long nerve gap repair. In consideration of our cellular therapy choice, the ability of BMSCs to adopt the characteristic supportive features of the Schwann cell, such as myelinization, makes them an appealing choice for nerve regeneration strategies. While Schwann cells are the main player in nerve regeneration process its inherent immunogenic properties makes it very difficult to use in clinical setting. Application of donor Schwann cells would require immunosuppression and obtaining them from recipient would necessitate harvesting of nerve tissue. On the other hand BMSC isolation from bone marrow is relatively straightforward process and usually produces substantial amount of cells. Moreover BMSC lack of immunogenicity in nonimmunoprivileged sites, which creates a practically unlimited source of cells from donors that might be applied clinically without immunosuppression [30, 49]. Studies involving direct injection of BMSCs into injured peripheral nerves have demonstrated that the cells become incorporated into the nerve and preferentially differentiate into Schwann cells, as evidenced by expression of Schwann cell-specific markers GFAP, S-100 [50].
Furthermore, in other reports, nerve regeneration studies using bioengineered and artificial nerve conduits have shown that addition of BMSCs to the conduit may enhance the functional and structural recovery offered by conduit alone [51–63]. In our isogenic study epineural tube alone was able to support faster recovery of denervation atrophy compared to previously reported data of 30 % shorter silicone conduit repair on the similar experimental model [29]. On the other hand, allogenic epieneural tube supported with BMSC had better clinical motor scores than saline filled tubes at 6 weeks. Moreover these constructs supported faster reinnervation of gastrocnemius muscle compared to autograft at 12 weeks. At 24 weeks BMSC enabled allogenic epineural conduit to have comparable GMI to autograft.
BMSC were present in the epineural conduits for up to 24 weeks after transplantation. The BMSC were shown to express S100 and other Schwann cell-related factors after transplantation, consistent with possible transdifferentiation in vivo [29, 62, 64]. Pereira Lopes et al. demonstrated in vitro expression of NGF by BMSC and presence of implanted BMSC in proximal nerve stump [58]. Similarly, we demonstrated in vivo expression of NGF, GFAP by BMSC in addition to increased laminin B and VEGF expression at 6 weeks. These findings suggest that transplanted isogenic BMSC undergo transformation in vivo into neural cell line phenotypes without the pretransplantation culture treatment with neural growth factors. Instead, epineural tube alone might provide the needed impulse for neural transdifferentiation as shown by increased NGF expression by BMSC when co-cultured with epineurium. In our models, we have observed up-regulation of both the proteins that contribute to nerve regeneration and myelinization neurotrophic factor NGF and extracellular matrix protein laminin, which expression is increased in remyelinating Schwann cells during axonal regeneration [65
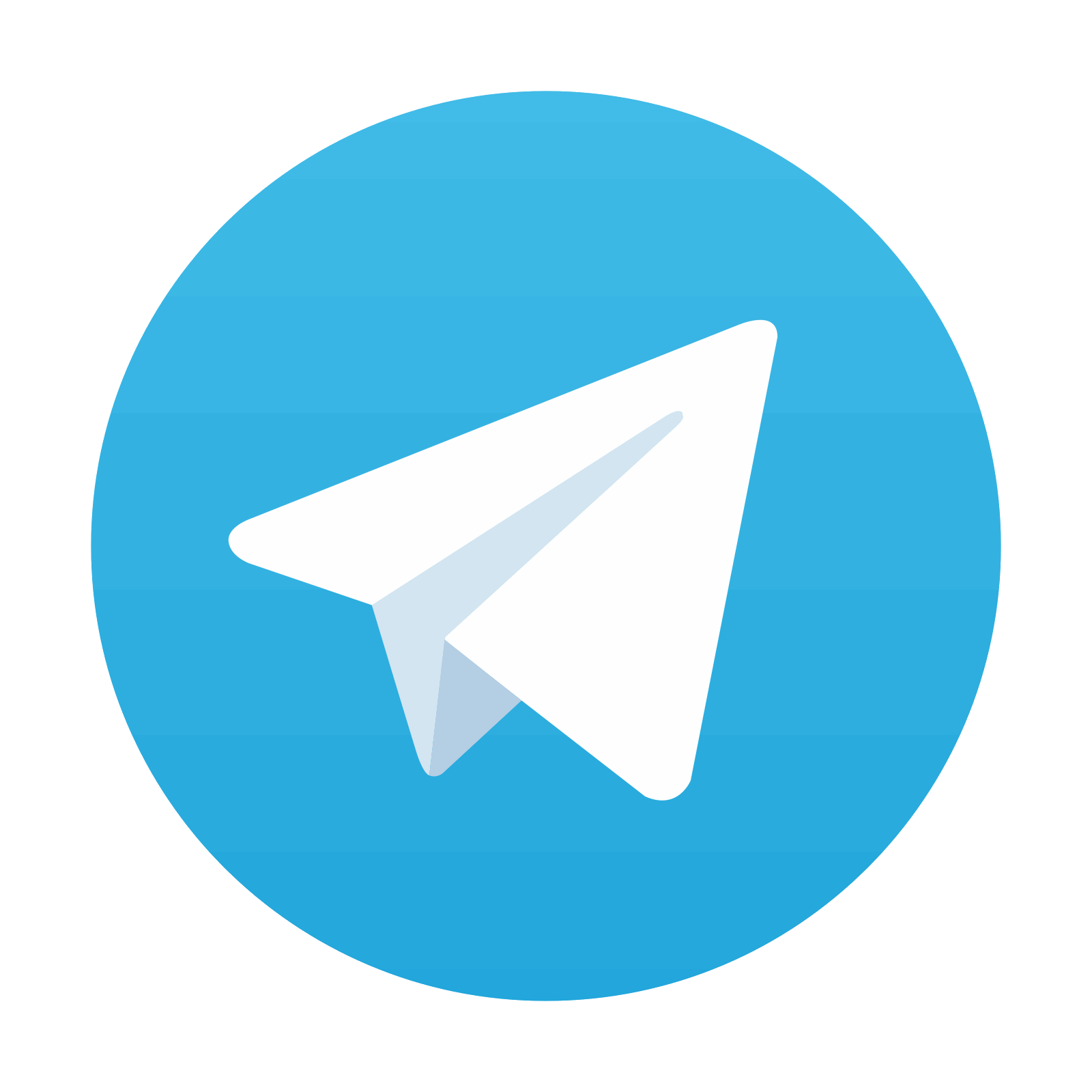
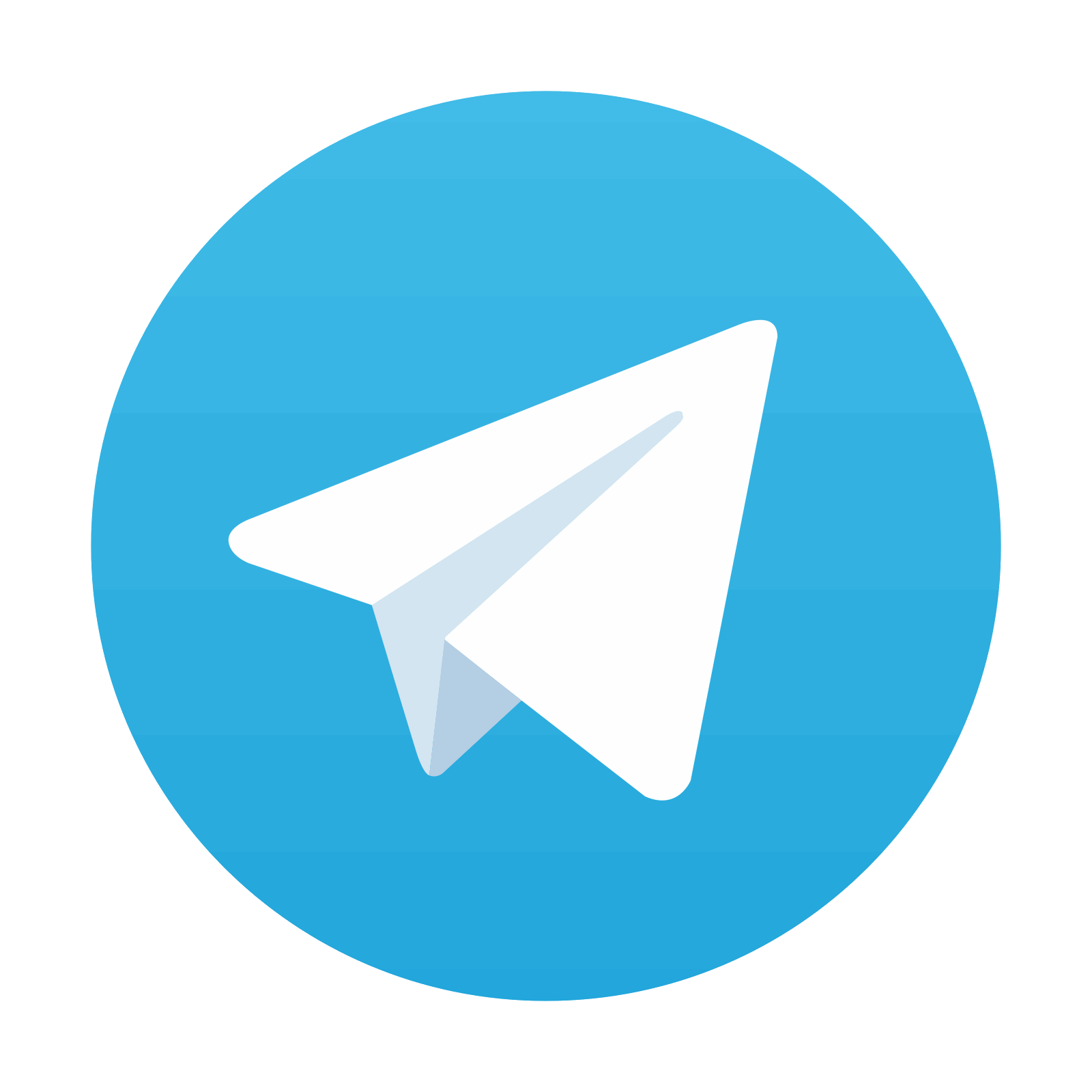
Stay updated, free articles. Join our Telegram channel

Full access? Get Clinical Tree
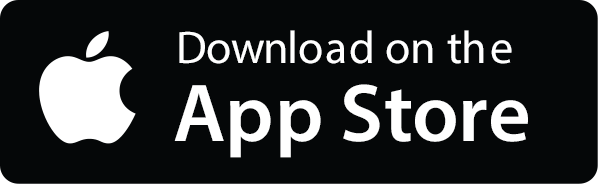
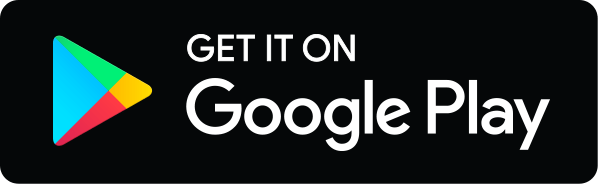