25 Congenital hand I: Embryology, classification, and principles
Synopsis
Consistency of terminology is necessary for optimal communication.
Limb outgrowth and patterning are controlled by specific signaling centers within the developing limb bud via the activation and interaction of molecular messengers.
Anomalies of limb development result from:
The anomaly may be isolated or syndromic.
The Swanson/International Society for Surgery of the Hand (IFSSH) classification is unable to incorporate satisfactorily alterations consequent on the increasing knowledge of the molecular basis of limb development, but continues to be the classification relied upon by most surgeons. An alternative approach incorporates an understanding of the mechanism of insult and the site within the developing limb which is primarily affected.
Assessment and treatment are specific for each child and family but are based on a detailed understanding of the processes of limb development, the psychological and physical effects of limb anomalies, and simple surgical principles.
Introduction
Classification
Swanson’s classification was adapted as the standard system by which congenital hand anomalies are described by the IFSSH in 1976.1 It is derived from ideas existing in the 1970s regarding limb embryology and is largely based on morphological appearance. Knight and Kay2 have presented an extended version, attempting to incorporate a list of all congenital anomalies. Regrettably, this classification, based as it is on appearance and relying on knowledge available in the 1960s and 1970s, is intrinsically unsuited to alterations based on causation and etiology at a molecular level. It is perhaps time to consider alternatives.
Limb development (embryology)
Overview of upper limb morphogenesis
Around day 26 (4 weeks) after fertilization (Carnegie stage 12), the upper limb bud appears as an oblong ventrolateral bulge on the body wall between somites 9–12 (C5–8) (Fig. 25.1).3 The emerging limb bud is composed of somatic lateral plate mesoderm covered by ectoderm. Subsequent limb bud growth and differentiation are described in terms of three coordinate axes: proximodistal, dorsoventral (dorsovolar), and anterioposterior (or radioulnar) (Fig. 25.2), each controlled by distinctive regions – signaling centers.
The ectoderm overlying the distal edge of the limb bud at the dorsovolar boundary thickens and forms a distinct ridge of stratified ectoderm, the AER.4 The thickened AER is critical for proximodistal outgrowth and also adds mechanical rigidity to the distal rim of the limb bud, flattening it along the dorsovolar axis. Excision of the AER in chicken embryo limb buds prevents further proximodistal outgrowth and results in limb truncation.5 Underlying the AER, the distal mesoderm exhibits robust proliferation and has been termed the progress zone (PZ). Cells in the PZ ultimately differentiate into specific cell types and are directed to specific positions within the limb.
The dorsal ectoderm is a critical signaling center for directing formation of dorsal and volar characteristics of the limb. Excision and rotation of dorsal ectoderm cause the formation of dorsal structures on the limb ventral surface.6 Another collection of mesodermal cells at the distal ulnar (posterior) margin is called the zone of polarizing activity (ZPA). Although not morphologically distinct, these cells direct radioulnar patterning and coordinate asymmetric limb patterning with the other signaling centers during development. Removal of the ZPA in animal models leads to loss of the ulna and the ulnar digits.7 Conversely, transplantation of these cells to the anterior (radial) aspect of limb buds of chicken embryos will result in formation of a mirrored complement of ulnar digits radially.8
Over the next week, the limb bud expands and elongates, particularly along the proximodistal axis (Table 25.1). By day 33 of development (Carnegie stage 14), differential growth and programmed cell death transform the distal portion into a paddle-shaped hand plate. There is also progressive mesodermal condensation along the proximodistal axis, forming a tripartite skeleton composed of a proximal section, the stylopod (shoulder girdle and humerus), a middle section, the zeugopod (radius and ulna), and a distal section, the autopod (hand). The joints become fully evident about day 51 (Carnegie stage 20) when the elbow and wrist joints flex and by day 56 (end of eighth week, Carnegie stage 23), the major morphologic features of the limb are complete (Fig. 25.1).
Table 25.1 Timing of hand formation
Time after fertilization | Hand development |
---|---|
27 days | Development of arm bud |
28–30 days | Further development of arm bud |
34–36 days | Elongation of arm bud |
34–38 days | Formation of hand paddle |
38–40 days | Early separation of digits |
44–46 days | Digits separated |
Week 9–10 | Formation of fingernails begins |
(Reproduced from: Gupta A, Kay SPJ, Scheker LR, editors. The growing hand: diagnosis and management of the upper extremity in children. London: CV Mosby; 2000, p. 25.)
The molecular control of limb outgrowth and patterning
During early embryonic development, Hox transcription factors set up a segmental body plan along the cranial–caudal axis.9 By the fourth week of development the presumptive upper limb fields are established, triggering the expression of Tbx5, Wnt3, and Fgf10 which initiate limb formation.10 Tbx5 determines forelimb identity, while Wnt3 and Fgf10 induce mesodermal proliferation and limb outgrowth. Mesodermal Fgf10, in conjunction with ectodermal radical fringe (R-fng) at the apical dorsovolar boundary, induces ectodermal thickening to form the AER.11–13 Formation of the AER initiates the expression of AER-related Wnt and Fgf proteins (including Fgf2/4/8/9/17)14,15 which, in turn, act on the mesoderm just underneath the AER to sustain Wnt3 and Fgf10 expression in the PZ. This reciprocal loop of ectodermal and mesodermal Fgf/Wnt proteins promotes progressive proximodistal outgrowth16,17 (Fig. 25.3). In the absence of Wnt3 or Fgf10 limbs fail to develop and tetramelia results.18,19 Application of Fgfs to the distal chick limb bud after AER removal will return proximodistal limb outgrowth.15 Within the PZ, mesodermal cells persist in an undifferentiated or receptive state, allowing the signaling centers to direct their fates.
The ZPA in the posterior (ulnar) limb mesoderm secretes a potent morphogen, sonic hedgehog (Shh), that regulates radioulnar patterning20 (Fig. 25.3). Shh induces posterior (ulnar) proliferation of the limb bud expanding its width.21,22 Moreover, Shh posteriorizes (ulnarizes) the developing forearm and defines the identity of the ulnar four digits. In a naturally occurring chicken mutant, limb-specific Shh expression is lacking and the upper limbs develop without ulnas and without digits.23 Application of exogenous Shh to the posterior (ulnar) aspect of the limb bud can fully recover normal limb morphology. Furthermore, application of Shh to the anterior margin produces mirrored duplication of ulnar digits radially.20
The AER and the ZPA are also closely linked by a reciprocal feedback loop that maintains Shh expression at the distal posterior (ulnar) border of the limb bud adjacent to the AER during progressive outgrowth.24–26 Removal of the AER causes regression of Shh expression and ablation of the ZPA induces a loss of Fgf signaling.25 Secretion of Wnt7a from the dorsal ectoderm induces the homeodomain transcription factor Lmx1b in the underlying mesoderm and asymmetrically dorsalizes the developing limb13,27,28 (Fig. 25.3). Wnt7a also contributes to the maintenance of Shh secretion from the ZPA29 linking the dorsovolar and radioulnar axes. Removal of dorsal ectoderm reduces Shh expression and disrupts posterior (ulnar) patterning.30 Thus, Shh plays a pivotal role during limb development linking dorsovolar, proximodistal, and radioulnar axes during outgrowth.30
Around the end of the fifth week of development the hand plate becomes visible. Interplay between Hox transcription factors (particularly Hoxd9-13 and Hoxa9-13) and Shh establishes digit number and identity31–34 (Fig. 25.4). Shh also induces an ulnar to radial (posterior to anterior) gradient that appears to involve Bmps in at least two roles in the formation and differentiation of digits. First, Bmps induce programmed cell death or apoptosis via discrete interdigital signaling centers, in part by repressing Fgf expression in the overlying AER.35–37 In addition, Bmps play a role in completing digital identity via the phalanx-forming region, a region overlying the distal digital anlagen that regulates Sox9 expression and chondrogenesis.38 Applying a Bmp-laden bead or transplanting Bmp expressing interdigital tissue from the third interdigital space to the second transforms the second digit into a third digit.35 The phalanx-forming region also maintains digit-associated Fgf expression in the overlying AER for continued digital outgrowth.38 However, it is still unclear how the various members of the Bmp family (Bmp2/4/5/6/7 and Gdf5/6) and their receptors (BmpR1a, BmpR1b) that are expressed in the digital and interdigital mesenchyme establish periodic thresholds that alternate between chondrogenesis and apoptosis. With regression of the phalanx-forming region and loss of overlying Fgf, the terminal phalanges form at the distal tip of each digit, invoking a unique poorly characterized mechanism that includes membranous ossification in addition to anlagen formation.39,40
The development/differentiation of specific tissues
Limb vasculature
As the limb bud grows, nutrients and oxygen are needed to maintain rapid cell proliferation and the secretory activity of the signaling centers. Induction of a primitive vascular system in the limb begins with transformation of mesoderm into angioblasts, cells that express the basic helix-loop-helix transcription factor Tal-1 and the vascular endothelial growth factor (VEGF) receptor Flk1.41 A dense meshwork of primitive vascular channels forms de novo from angioblasts in the limb mesoderm.42,43 Angioblasts formed in nearby somites also migrate into the limb bud and contribute to the formation of new limb vessels through continued vasculogenesis.44 As angioblasts aggregate, differentiate into endothelium, and form primitive vascular tubes, new vascular markers emerge. Flk1 persists to allow further remodeling by VEGF, Tal-1 diminishes and VE-cadherin, a cell–cell adhesion molecule, is up-regulated.
The primitive vascular network undergoes significant remodeling as the limb develops. By stage 13, the vascular channels coalesce proximally to form a central artery (subclavian) that connects to the dorsal aorta via the seventh intersegmental artery45 and two peripheral veins form that drain into the posterior cardinal system.43 In addition to vasculogenesis, angiogenesis, i.e., vessel sprouting from a preformed vessel, also contributes to the definitive architecture of the limb vasculature.
Construction of the vascular pattern is under the direction of the coordinate signaling centers and involves the regulation of specific VEGF family members and VEGFR3 receptors.45–47 The final vascular pattern progresses from proximal to distal (Fig. 25.5) – with formation of the axillary artery by stage 17 and the brachial and major forelimb branches by stage 19. The median artery is prominent in the forearm.48 Distally the capillary plexus persists. Ulnar differentiation precedes radial differentiation and is evident distally by the radial artery merging with an extensive primitive capillary plexus radially, while the ulnar side of the axis is already forming the palmar arch which communicates with the ulnar associated capillary plexus.49 The median and interosseous arteries decrease in size. The median artery degenerates, providing blood supply to the median nerve only.48
The vascular network must include arteries, capillaries, and veins. The diameter of each is dictated by blood flow, blood pressure, shear stress, and the accumulation of vascular smooth muscle around the vessel.42 Formation of vascular smooth muscle around limb arteries lags behind vessel formation by about 2 days. It is unclear whether these muscle cells are derived from the endothelial cells or are condensations of the surrounding mesoderm. Furthermore, the mechanism underlying differential smooth-muscle accumulation in arteries and veins is unknown. Arteries are also differentiated from veins by the expression of Ephrin B2, a membrane-bound ligand, while the Eph-B4 receptor highlights veins. Presumptive capillaries will be negative to both Ephrin B2 and the Eph-B4 receptor.
By stage 21 the major vessel architecture is complete. The majority of vascular anomalies arise between stage 17 and 21, i.e., between days 41 and 52 or largely within the seventh and early eighth week of development. Lymphatics follow a similar course, albeit less well delineated, and are also composed of angioblasts that migrate into the limb from somitic mesoderm.44 A defining molecular difference in lymphatic vessels is the coexpression of PROX-1 and LYVF-1.50
Skeletogenesis
Under the influence of the limb signaling centers, Sox9, a high-mobility group transcription factor, is up-regulated in a targeted population of limb mesoderm.51 Sox9 transforms these cells into chondrogenic precursors and induces condensation, the first step in limb skeletogenesis.52,53 Chondrogenesis occurs in a proximal to distal progression (Fig. 25.6). The expression of Sox5 and Sox6 is required for further differentiation of chondrogenic precursors into chondrocytes to form cartilage anlagen.54
At precise locations within the forming skeletal anlagen, synovial joints form. Hoxa transcription factors (Hoxa9-13) exhibit expression patterns along the proximodistal axis that correlate with skeletal segmentation.54 In addition, several molecules are known to participate in the process of joint formation, including Wnt14 and Gdf5. The first morphologic evidence of joint formation is the compact cellular condensation called the interzone that expresses Wnt14.55 In addition, Gdf5 is induced in the proximal portion of the interzone, covering the distal end of the proximal anlagen.56,57 The central region of the interzone begins to expand, accumulates hyaluronan, and becomes hypocellular in a process termed cavitation.58,59 The two cellular regions of the interzone begin to differentiate into the opposing articular cartilage surfaces. Patterning signals and movement work in concert to shape the joint into its definitive morphology57 (Fig. 25.7). Mesoderm surrounding the developing joint condenses to form the joint capsule.60
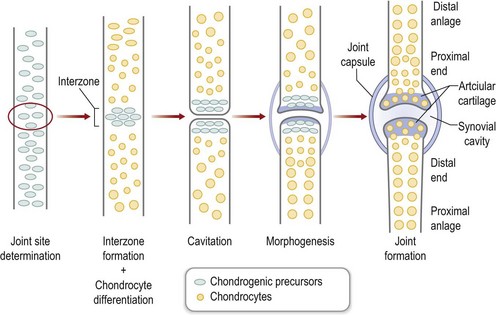
Fig. 25.7 Joint formation.
(Modified from Pacifici M, Koyama E, Iwamoto M. Mechanisms of synovial joint and articular cartilage formation: recent advances, but many lingering mysteries. Birth Defects Res C Embryo Today 2005;75:237–248.)
Endochondral ossification converts the cartilage anlagen into the skeletal framework of the growing limb. This process is under precise regulation and involves Runx2, Twist1, Fgfs, Indian hedgehog (Ihh), and vascular endothelial growth factors (Vegfs).53 Chondrocytes are induced to proliferate, undergo hypertrophy, and then die, leaving an extracellular cartilage matrix. This matrix is subsequently invaded by blood vessels, osteoclasts, and differentiating osteoblasts. Osteoblast differentiation is also under the control of Runx2 and Osterix (Osx), a bone-specific transcription factor.61 Ossification begins within the diaphysis of anlagen at the primary ossification center during early fetal development. Subsequently, vascular invasion of the proximal epiphysis occurs, followed by the distal epiphysis forming secondary ossification centers later in development. Each metacarpal and digital phalanx has two ossification centers, a primary center within the diaphysis and a single secondary center that develops postnatally.
Myogenesis
Muscular development of the upper limb is a coordinated effort between segment-specific tendon primordium, migrating myocytes, and migrating motor neurons.62,63 There are three phases to myogenesis.64 Embryonic myogenesis establishes the primary myotubes and the basic muscle layout. Later, a second wave of myogenesis occurs with secondary myofibers surrounding primary myofibers and contributing to the bulk of the muscle mass present at birth. Finally, satellite cells which take up residence in the basal lamina surrounding myofibers will contribute to postnatal growth and muscle regeneration.65
During early limb bud formation, limb mesoderm condenses to form the proximal tendon primordium (PTP), establishing a target and an initial scaffold for migration of myocytes.62 Myocyte precursors of the limb arise from the dorsolateral aspect of associated somites (the dermomyotome subdivision) and express the Pax3 transcription factor. Muscle precursors for the limb and body wall express c-Met, a surface receptor that is modulated by scatter factor initially emanating from the lateral plate mesoderm and then later from other sites, including the PZ. Scatter factor acts as a chemoattractant to promote myocyte precursor migration. A population of the myocyte precursors further differentiates into limb-specific precursors, demarcated by Lbx1 expression66 (Fig. 25.8).
During embryonic myogenesis, limb-specific myocytes migrate into the proximal limb bud, initially as dorsal and ventral masses (Fig. 25.8). Continued migration, however, is not haphazard; rather myocyte precursors are directed into muscle anlagen by the tendon primordium, e.g., the ventral mass migrates into the biceps and brachialis under the direction of the PTP (Fig. 25.9, Carnegie stage 15). With continued proliferation and differentiation, the myocytes up-regulate MyoD and Myogenin, declaring their commitment as myocytes. These myocytes will then coalesce to form fibers and begin to produce myosin filaments. Satellite cells, important for later growth and muscle regeneration, take up residence just under the basal lamina of the developing myofibers.65 Concurrently, the tendon primordia further define the shape of specific muscles with discrete tendinous attachments. For example, expression of Lmx1b within the dorsal tendons directs the unique pattern of extensor attachments67 and it is likely that each upper limb muscle is shaped by a unique combination of patterning factors.68
As in other aspects of upper limb development, there is progressive differentiation from proximal to distal. Thus, as the muscles of the upper arm take shape, migrating myocytes invade the forearm to associate with the PTP and a new mesodermal condensation forming at the presumptive wrist, the intermediate tendon primordium. In the forearm, the superficial muscles differentiate before the deep muscles. By Carnegie stage 17, the distal tendon primordia form and associate with migrating myocytes destined to be muscles of the hand (Fig. 25.9). The intrinsic muscles arise from five embryonic muscle layers, which differentiate and fuse in a complex but logical manner.69,70 Following embryonic myogenesis, a second wave of myocyte precursors migrate into the limb and coalesce around primary myofibers, forming secondary myofibers and adding bulk to the muscle masses as the fetus grows. It is during this secondary or fetal myogenesis that formation of motor endplates occurs and neuromuscular communication begins, further differentiating the forming muscle with slow and fast fiber types.71,72
Innervation
Outgrowth of nerves into the limb bud lags behind muscle migration (Fig. 25.10) and involves both motor and sensory neurons.73 Motor neurons are specified early during spinal cord development by exposure to Shh, initially from the notochord and later from the floor plate of the developing neural tube.74,75 Motor neurons begin to express a combination of transcription factors (e.g., Hb9/Mnx1, Lhx3/4) that promote motor neuron migration into discrete columns within the spinal cord and direct their axons to specific muscle groups.63 Within the limb fields (specified by Hox6 in the forelimb and Hox10 in the hindlimb), a Hox accessory factor, FoxP1, is expressed that assists in deciphering the subsequent Hox-specific code within the limb for appropriate axon targeting.76 As the axons of the motor neurons enter the limb, those expressing Lim1 (Lhx1) will project into the dorsal Lmx1b-expressing compartment of the limb to target dorsal extensor muscle groups. The remaining axons express Isl1 and will enter the ventral compartment to target flexor muscles of the limb.
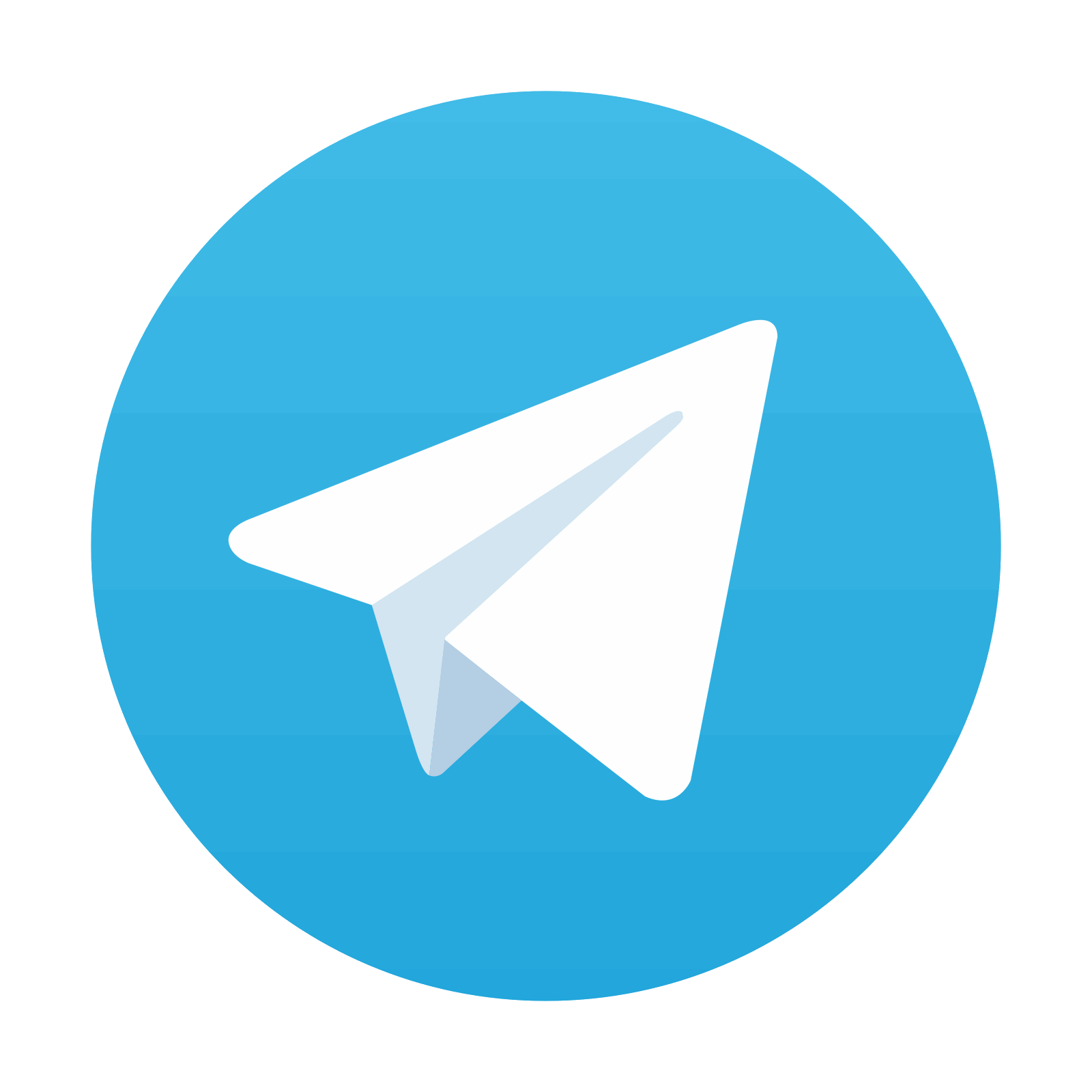
Stay updated, free articles. Join our Telegram channel

Full access? Get Clinical Tree
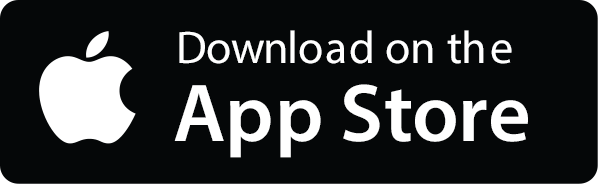
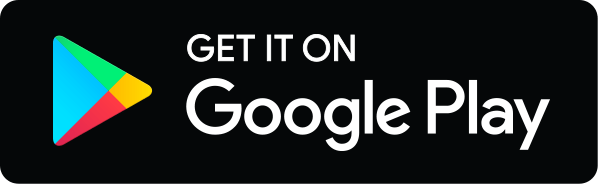