Chapter 42 Chemical Modification of Tendon Gliding Surface
This chapter does not appear in the print edition.
Tendon Lubricants
The flexor digitorum profundus (FDP) tendon glides within tendon sheath, which consists of annular ligaments or pulleys, during the movement of fingers. This tendon-pulley system has a low coefficient of friction (0.04), which is similar to that of articular cartilage (0.014).1
Lubrication mechanism of articular cartilage has been widely investigated.2,3 Hyaluronic acid (HA), lubricin, and phospholipids are thought to be involved in the lubrication of the cartilage–cartilage interface, acting alone or in combination. HA is a polyanionic polysaccharide, present in the intercellular matrix of most vertebrate connective tissues, especially in synovial fluid. The unique properties of HA result in a molecular network that, in highly hydrated conditions, is extremely viscoelastic and pseudoplastic, favorable rheological properties for a potential lubricant. Lubricin, also known as superficial zone protein and proteoglycan 4, is a glycoprotein which has been identified in synovial fluid4,5 and the superficial zone of articular cartilage.6 It has been noted to lubricate articular cartilage with the same lubricating properties as normal synovial fluid. Lubricin is widely considered to be the principal lubricant in joints.3,7 Furthermore, phospholipids, the major component of all biological membranes, are known to contribute to joint lubrication and to lubricate other gliding surfaces as well.8,9
HA and lubricin have been identified on the surface of the FDP tendon.10–12 While phospholipids have not been sought in tendon, it is reasonable to assume that some are present, as phospholipids are found in all living tissues. To assess the effect of HA, lubricin, and phospholipids on tendon lubrication, the enzymatic and chemical treatments were applied to remove them from the surface of canine FDP tendons.13
Hyaluronidase can effectively digest HA. The effect of the hyaluronidase digestion on the friction between FDP tendon and its associated pulley was measured. The friction between the FDP tendon and its pulley increases after the FDP tendon is treated with hyaluronidase (Figure 42-1).10,13 Therefore, HA has been assumed to play a role in lubricating the surface of the FDP tendon.
Phospholipase C selectively removes certain types of phospholipids, such as phosphatidylcholine, without destroying the extracellular matrix network.14 The increase in friction after treatment with phospholipase C suggests that phospholipids are present on the surface of the FDP tendon, and that phospholipids help to lubricate FDP tendon (see Figure 42-1). This conclusion was further confirmed by the increase in friction after treatment with lipid solvent. The increased effect of lipid solvent compared to phospholipase C suggests that phospholipids other than phosphatidylcholine may also affect tendon lubrication.
Trypsin can digest lubricin as well as other extracellular matrix proteins except collagen, the principal protein in tendon. Trypsin digestion resulted in a significant increase of friction of the FDP tendon (see Figure 42-1). Although the increase of friction could contribute to phospholipids, which could be carried by lubricin and/or other extracellular matrix proteins, it was found that the friction of the FDP tendons could be further increased with trypsin after the elimination of phospholipids with lipid solvent (see Figure 42-1). Hence, the effect on friction could be due to a removal of protein components, especially lubricin, a glycoprotein both known to have lubricating ability, although it cannot exclude an effect from other proteins on the tendon surface.
Chemical Surface Modification of Tendon in Vitro
Tendons attach muscle to bone and transfer the power of the muscle to bone over a distance. The structure of tendon is directly related its physical and physiological function. The surface of tendons well adapts to their mechanical environments.15 Tendons are characterized based on their anatomic location as either intrasynovial or extrasynovial.16–18 The intrasynovial tendons, such as the FDP tendon, have parietal and visceral synovial sheaths that form a closed compartment that contains synovial fluid for lubrication and nutrition. In extrasynovial tendons, such as the peroneus longus (PL) tendon, there is a peritendinous sheet of loose fibrillar tissue, the paratenon, which functions as an elastic sleeve, permitting limited movement of the tendon against the surrounding tissue. The extrasynovial autograft, intrasynovial allograft, and the repaired flexor tendon are the accepted procedures in flexor tendon surgery. The chemical surface modification of these tendons is described later.
Extrasynovial Autograft
Tendon graft plays an important role in reconstruction to restore finger function in the cases of severe flexor tendon damage, adhesion formation, and primary tenorrhaphy rupture. As the potential sources of intrasynovial tendons available for use as tendon autografts are limited, the most donor tendons, such as palmaris longus tendon, plantaris tendon, and long extensor tendon, come from extrasynovial tendon sources in clinical settings. Extrasynovial tendons have rougher surfaces than intrasynovial tendons.19,20 The coating of paratenon on the surface of extrasynovial tendon is extremely susceptible to damage as the tendon repetitively glides against the pulley. Extrasynovial tendons create higher friction against a pulley compared to intrasynovial tendons, particularly after multiple repetitive motion.20–22 Animal models have shown that extrasynovial tendon grafts are associated with more adhesions to the surrounding tissue than intrasynovial tendon grafts.19,23,24 Therefore, outcomes of extrasynovial grafts may be improved by keeping paratenon intact as well as low friction of the grafts.
HA is a linear, unbranched polysaccharide with a high molecular weight. It is composed of a repeating disaccharide that consists of N-acetyl-d-glucosamine and d-glucuronic acid linked by a β1–4 glycosidic bond (Figure 42-2).25 The disaccharides are linked by β1–3 bonds to form the HA chain. Each disaccharide has one carboxyl group, which can be activated by carbodiimides at physiological condition to form an amine-reactive O-acylisourea intermediate that quickly reacts with an amino group to form an amide bond and release an isourea. As amino group universally exists in the protein components, such as collagen, the major extracellular matrix in tendon, with the activation of carbodiimides, HA could cross-link paratenon and tendon and keep paratenon resist abrasion. Furthermore, HA is a lubricant of tendon. The chemical reaction can tightly bind HA on the surface of tendon and play a role in lubrication (Figure 42-3).
We performed a study to evaluate HA and carbodiimide derivatized HA (cd-HA) on the gliding of canine PL tendon, an extrasynovial tendon.26 We found that the gliding resistance of normal PL increased with the increase of cycle of repetitive motion (Figure 42-4). The administration of exogenous HA (1%) resulted in the nonchange of gliding resistance in the first cycle of motion after the administration. However, the gliding resistance of PL treated with HA increased significantly over five cycles of repetitive motion than normal PL did. The quick discharge of the lubricating ability of exogenous HA indicates the weak attachment of HA on the surface of the tendon. cd-HA, which is composed of 1% HA with 1% 1-ethyl-3-(3-dimethylaminopropyl)carbodiimide hydrochloride (EDC) and 1% N-hydroxysuccinimide (NHS), significantly decreased the gliding resistance of the PL tendon compared with that of normal PL tendon or the PL tendon treated with HA only, at each corresponding cycle of repetitive motion. This finding indicates the chemical modification improves the attachment of HA and/or paratenon on the surface of the PL tendon.
Although cd-HA improves the gliding of the PL tendon, the gliding resistance decreased significantly only in the first cycle of motion after the administration and increased over 20 cycles of repetitive motion. Gelatin, which serves as a carrier of HA and a cross-linker and a cross-linker, was introduced to further improve the gliding of the PL tendon. It was found that all PL tendons treated with saline, 10% gelatin, or cd-HA (1% HA, 0.25% EDC, and 0.25% NHS) showed similar trends up to 500 cycles of repetitive motion22,27 (Figure 42-5). Over the first 200 cycles, the gliding resistance increased linearly and then reached a plateau. There was no significant difference in gliding resistance between the saline-, gelatin-, and cd-HA–treated PL tendons for any given number cycles of motion. For the PL tendon treated with cd-gelatin (10% gelatin, 0.25% EDC, and 0.25% NHS) and cd-HA–gelatin (1% HA, 10% gelatin, 0.25% EDC, and 0.25% NHS), the gliding resistance increased at a much more gradual rate over the 500 cycles. Beginning at 100 cycles of simulated flexion/extension, the gliding resistance of tendons treated with cd-HA–gelatin was significantly lower than that of the saline-treated tendons. Starting at 200 cycles, the gliding resistance of tendons treated with cd-gelatin was also significantly lower than that of the saline-, gelatin-, and cd-HA–treated PL tendons. Starting at 300 cycles, there was also a significant difference in the gliding resistance between the PL tendon treated with cd-gelatin and the PL tendon treated with cd-HA–gelatin (p < 0.05), with the latter group having a lower gliding resistance at comparable cycles of simulated flexion/extension. At the 500th cycle, the increase in gliding resistance of the cd-HA–gelatin treated PL tendons was roughly 4 times that of the FDP tendon, compared to a 20-fold difference for the saline treated PL tendon. The gliding of the PL tendon treated with cd-HA–gelatin almost approached that of the FDP tendon. The chemical modification of the surface of the PL tendon with cd-HA–gelatin also significantly improved its resistance to abrasion. After 500 flexion/extension cycles, the surface of untreated PL tendons was roughened, and the collagen fibers covering the PL tendon surface were twisted into large strands. In contrast, the surface of the PL tendon treated with cd-HA–gelatin was still smooth after 500 cycles of repetitive motion, and the normal collagen fibers on the PL tendon surface remained intact, similar to the FDP tendon.
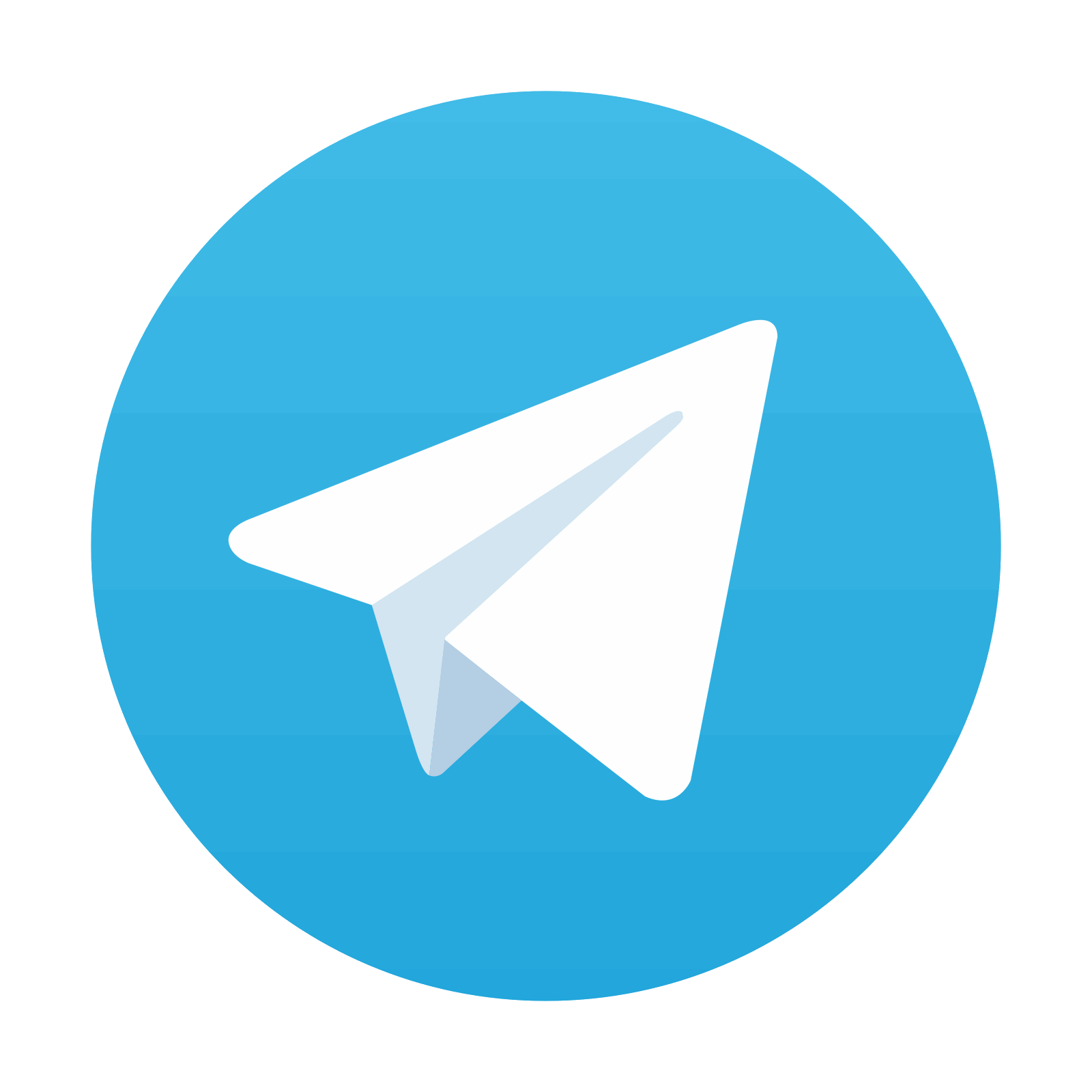
Stay updated, free articles. Join our Telegram channel

Full access? Get Clinical Tree
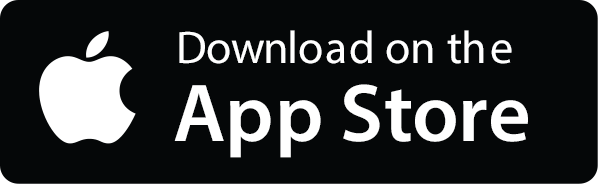
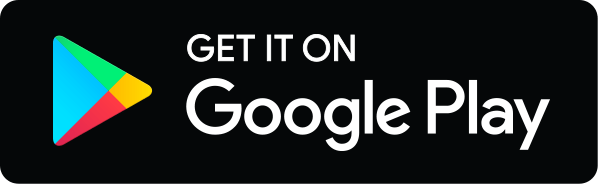