Fig. 72.1
Types of cells derived as a result of chemical (polyethylene glycol/dimethyl sulfoxide – PEG/DMSO) ex vivo fusion of two different cell lineages. Fusion of cells derived from the same lineages creates syncytium with multiple nuclei N ≥ 2 (1) or with single nucleus – homotypic synkaryon (2). Fusion of cells derived from different lineages creates heterotypic synkaryon (3) or heterokaryon (4). If the fusion of cells derived from different lineages is not complete, hemi-fused cells are created (5). Toxicity of fusion can cause cell death (6). Cells can also not undergo fusion due to lack of other cells in proximity or inappropriate fusion conditions (7)
Most of the knowledge explaining the molecular mechanism of in vivo spontaneous CF, as well as fused cell properties, comes from studies of cancer cell lines [5] and bone marrow transplantation [6]. In 1961 the first in vitro spontaneous CF conditions between mammalian cells were established [7]. The discovery of spontaneous fusion between pluripotent embryonic stem cells and mouse bone marrow cells [8] or brain progenitor cells [9] created an interest in CF as a process that could be applied for tissue regeneration.
CF is considered to be one of the forces altering a cell’s fate by modifying phenotype and function. Multiple in vitro studies demonstrated that hybrids created as a result of fusion are presenting mixed/intermediate phenotype and gene expression patterns derived from both fusion donors in migratory activity [10, 11], proliferation capability [11, 12], cell surface protein expression [8, 9], or drug resistance [13, 14]. The work of pioneers such as Terada [8] and Ying [9] revealed that cells created by spontaneous CF could express phenotype characteristics of undifferentiated cells or properties of both types of cells undergoing fusion. The possibility of formation of stable multinucleated heterokaryons as a result of spontaneous fusion of bone marrow derived cells with several types of fusion friendly cells such as: skeletal muscle, cardiac muscle, liver, monocytes, mesenchymal stem cells, hematopoietic stem cells/progenitor cells, macrophages, B and T lymphocytes, intestine cells, and Purkinje neurons was confirmed by multiple in vivo studies [6, 15–25]. In these experiments fused cells not only presented mixed phenotype, but also overtook the function of the injured recipient cells and helped facilitate the process of tissue regeneration. The interest in application of bone marrow derived cells in various medical fields such as tissue regeneration and transplantation is increasing due to their potential therapeutic effects.
In Vitro Cell Fusion
In the early 1960s, in vitro CF was performed to describe the effect of viruses such as hemagglutinating virus of Japan (HVJ) on murine cell cultures [26–28]. Currently, there are three major CF methods: chemical, electrical, and viral. Advantages and disadvantages of each method were compiled and are presented in Table 72.1. New methods of CF applying different agents such as cephalin, bispecific nanoparticles, fusogenic cell lines, or v-fusion are investigated [29–32]. Combining different fusion methods and introducing additional modifications of conventional techniques have been studied to improve the fusion efficacy, either by increasing the cell-to-cell contact capability or permeabilization area. The selection of proper fusion method and protocol optimization depends on the cell type, cell number, culture conditions (monolayer vs. cell suspension), their sensitivity to unfavorable conditions, and equipment availability. The efficacy of polykaryons creation can be controlled by factors such as time and/or fusing agent concentration. Higher numbers of polykaryons can be also generated by repeating the fusion procedure [33]. Additionally, the application of supporting agents such as dimethyl sulfoxide (DMSO), in case of polyethylene glycol (PEG) mediated fusion, was confirmed to be more efficient in creating higher number of fused cells than using PEG alone [34, 35]. PEG, due to its hydrophobic properties, decreases the distance between cells by removing water (thermodynamically unfavourable environment) and causing their aggregation. Dehydration leads to asymmetry in the lipid membrane bilayers leading to formation of a single bilayer septum at a point of close apposition of two cell membranes and facilitates formation of the pore following septum decay (Fig. 72.2).
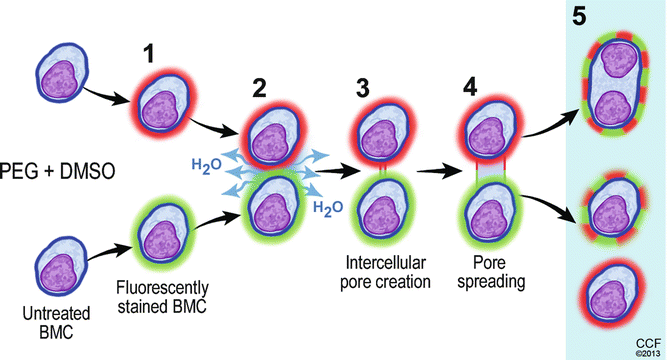
Table 72.1
Comparison of three of the most popular in vitro fusion methods: chemical (polyethylene glycol, PEG), electrical and virus induced fusion applied for creating cell hybrids
Types of fusion | Chemical fusion – PEG | Electrofusion | Virus induced fusion (biological fusion) |
---|---|---|---|
Mechanism | Cells are brought closer by removal of water between the cells and further dehydration changes symmetry of lipid membrane | Application of pulsed electric field: Alternating current aligns cells and direct current forms temporary pores in cell membranes | Applies noninfectious or inactivated viruses (Sendai virus-most common use, HVJ, SV5, coronavirus, rhabdovirus) expressing viral envelope glycoproteins |
Efficacy | Low | High | Low |
Number of cell types that can be fused during single fusion procedure | Multiple cell types can be fused at the same time | Not more than two cell types during fusion procedure | Not more than two cell types during fusion procedure |
Equipment | No additional equipment | Fusion chamber | Requires facility to work with viruses |
Advantages | Fast, cheap and easy | Fast and easy | Less toxic |
Disadvantages | Cell-type dependent toxicity, depends on shape, size, and intensity of shaking | Cell-type dependent toxicity, similar size cell can be fused, cost | Length of procedure depends on cell type, productions/purchase of inactivated virus, continuous to fuse for a period of time, immunologic reaction, cost |
Scaling up | Possible | Limited | Possible |
Clinical application | Possible | Possible | Limited (potential for viral infection) |
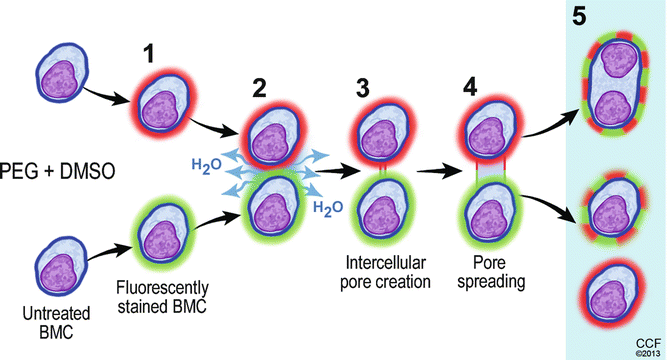
Fig. 72.2
The mechanism of polyethylene glycol/dimethyl sulfoxide (PEG/DMSO) induced donor-recipient chimeric cells creation via ex vivo cell fusion (CF). PEG mediated CF is a three-step process requiring the following: (1) aggregation or “close” (the intercellular distance may vary for different cells and fusion models) approach of membrane lipid bilayers due to hydrophobic properties of PEG that causes membrane dehydration; (2) removal of the water between adjacent cells; (3) the intermediate membrane destabilization (facilitated by PEG) is followed by creation of pores (facilitated by DMSO) in the membranes of cells undergoing fusion; (4) positive osmotic pressure created by PEG improves stabilization of fusion intermediates and leads to expansion of the pores, cell swelling and cell-to-cell fusion. The products of PEG/DMSO solution induced cell fusion may include (5) heterokaryon and synkaryon cells as well as cells that did not undergo fusion process. More detailed descriptions of cell fusion mechanism can be found in articles by Lentz [62, 63]
Application of Cell Fusion as a Research Tool
Ex vivo CF can be a crucial tool in creating new cell types or improving research models used in vitro in the basic mechanistic studies (e.g. cancer research) or for more efficient drug/treatment development. Recently ex vivo CF was applied to create human insulin-releasing 1.1B4 cells, which are able to form pseudoislets [36]. Most of the studies describing interactions and insulin secretion were performed using B cells routinely grown in the monolayers, which can impact their secreting properties. The use of fused cells in this model may improve the knowledge of regulatory mechanism underlying insulin secretion and survival of human pancreatic B cells. Additionally, it can help develop better types of insulin secreting cell therapies against diabetes [36]. CF is a well-established procedure in the process of producing monoclonal antibodies, which are widely used in research application such as protein or cell type detection, blocking cell activity, studying cross-reactivity, and antigen purification. Monoclonal antibodies are produced by immortalized hybridoma cells, which are created by ex vivo CF between antibody secreting B cells and myeloma cells. The first report describing the creation of hybridoma was published in 1973 by Schwaber and Cohen [37] and was soon followed by a procedure of fusion between myeloma cells and B lymphocytes isolated from spleen of immunized animal, in 1975 by Kohler and Milstein [12]. Generated cells were characterized by both lymphocyte properties to produce specific antibodies and the immortal character of the myeloma cells. CF played a critical role in a variety of studies exploring processes such as cancer pathogenesis, explaining trans-differentiation of committed somatic cells through cell reprogramming, or even assessing the effects of nuclear transfer of nucleus of one cell transferred to the cytoplasm of enucleated oocyte [38]. Examples of application of CF in research are presented in Table 72.2.
Table 72.2
Examples of research studies in which ex vivo CF was applied as a research tool
# | Study problems utilizing CF as a research tool | Reference |
---|---|---|
1 | Epigenetic reprogramming | [39] |
2 | Genomic instability | [40] |
3 | Cancer progression | [41] |
4 | Determination of dominance or recessiveness of genes | |
5 | Dynamics of intracellular components | |
6 | Results of polyploidy | [46] |
7 | Hybridomas for monoclonal antibodies production | [12] |
8 | Radiation hybrids to map genomes | [47] |
9 | Aneuploidy | [48] |
Application of Cell Fusion as a Treatment Option
Clinical application of ex vivo CF has been limited to the scarcity of knowledge of the fusion mechanism, as well as fused cell function and safety. However, CF has a potential to become a useful tool in the armamentarium of highly anticipated methods focused on organ and tissue function regeneration. Currently, CF is utilized either indirectly during production of monoclonal antibodies or directly by creating cellular therapeutics. Monoclonal antibodies are used to treat a variety of diseases and conditions such as cancer, autoimmune disease, inflammation, and transplant rejection. Creation of immortalized hybridomas is an essential part of monoclonal antibody production. Antibodies such as Muromonab-CD3 (anti-CD3) are directly produced by mouse hybridomas [49]. However, basiliximab (anti-CD25), which is a chimeric antibody, is primarily created by mouse hybridoma and further modified by utilizing recombinant DNA technology to replace the mouse immunogenetic component of the antibody with human protein [50].
The concept of direct application of ex vivo CF in creation of cellular therapies was initiated by observations of opposite sex hematopoietic cell transplantation. The ability of transplanted stem cells to fuse spontaneously with defected cells and restore their function originated the research focused on creating cellular therapies. These experimental therapies are oriented towards approaches such as fusion of differentiated cells with stem cells or reprogramming of specialized cells to pluripotent state via CF of embryonic stem cells or embryonic germ cells. In theory, ex vivo CF could be applied as a cell-based gene-delivery system for treating genetic and non-genetic diseases such as muscular dystrophy, tyrosinemia, hemophilia, or diabetes type 1 and 2.
Other approaches, which utilize direct application of CF, can be found in cancer immunotherapy. Anti-cancer vaccines created by ex vivo CF between dendritic cells (DC), which are potent antigen presenting cells, and carcinoma cells are among the most promising strategies dedicated to introduce cancer specific antigens to DC. The aim of this approach is to enhance immune system response against cancer cells. Fusion between DC and tumor cells eliminates the limitation of low number of known tumor-associated antigens available for HLA molecules. Hybrid cells containing unidentified molecules and expressing them in combination with MHC Class I and II molecules in the presence of co-stimulatory signals may be an effective alternative for patients suffering from rare forms of cancer. The results of cancer immunotherapy using anti-cancer vaccines in vitro, as well as in mouse model, showed potent anti-tumor response against multiple tumor-associated antigens [51, 52]. Currently, this immunotherapy is being evaluated in the treatment of melanoma and breast cancer patients in phase II clinical trials.
Cell Fusion in the Field of Transplantation
The infusion of bone marrow-derived cells, presence of chimerism in peripheral blood, and following migration of donor-derived cell to lymphoid organs was associated with prolonged survival of the transplants or even tolerance induction [53–57]. Bonde et al. [58] reported that during co-culturing spontaneous fusion of bone marrow cells derived from two different mice strains occurred. In vivo study confirmed this result following allogenic and syngenic transplantation. Further, it has been shown that fused cells, on their surface, expressed both donor and recipient MHC antigens.
Siemionow’s group also performed experiments on CF of bone marrow derived cells [59]. Results of this preliminary study were in line with results of Bonde et al. [58] and showed that there is a possibility of creating in vivo donor-recipient fused cells that can facilitate face allograft survival (Article in press). Short immunomodulatory protocol of anti-αβ-TCR monoclonal antibody and cyclosporine A was used to facilitate engraftment of spontaneously created donor-recipient chimeric cells (DRCC).
Ex Vivo Cell Fusion as a New Approach for Tolerance Induction
The successful establishment of protocol creating in vivo DRCC either via the mechanism of trogocytosis or spontaneous CF is opening many possibilities of using bone marrow derived cells as a tool for development of novel therapeutic products. Although, further research on DRCC is necessary in order to fully understand the underlying mechanisms of their creation and action in vivo, the creation of tolerance-inducting cells could be a breakthrough modality in solid organ and CTA transplantation.
Ex Vivo Creation of the Donor-Recipient Chimeric Cells: Animal Model
There are several disadvantages for creating DRCC in vivo in the clinical scenario as described in the previous chapter (Chap. 71). The most challenging issue in clinical execution of the primary chimera creation protocol is the critical time frame following bone marrow infusion required for development of chimeric pro-tolerogenic environment in the transplant recipient. Pre-treatment of the transplant recipient in order to create in vivo DRCC will be possible only for the living organ donor. To overcome this hindrance and move forward to a more clinically applicable model, Siemionow’s group adopted a new approach to create DRCC via ex vivo CF. DRCC therapy was created by fusion of donor and recipient bone marrow derived cells by polyethylene glycol (PEG) technique. Briefly, bone marrow cells were harvested from the tibia and femur bones of two fully MHC mismatched ACI (RT1a) and Lewis (RT1l
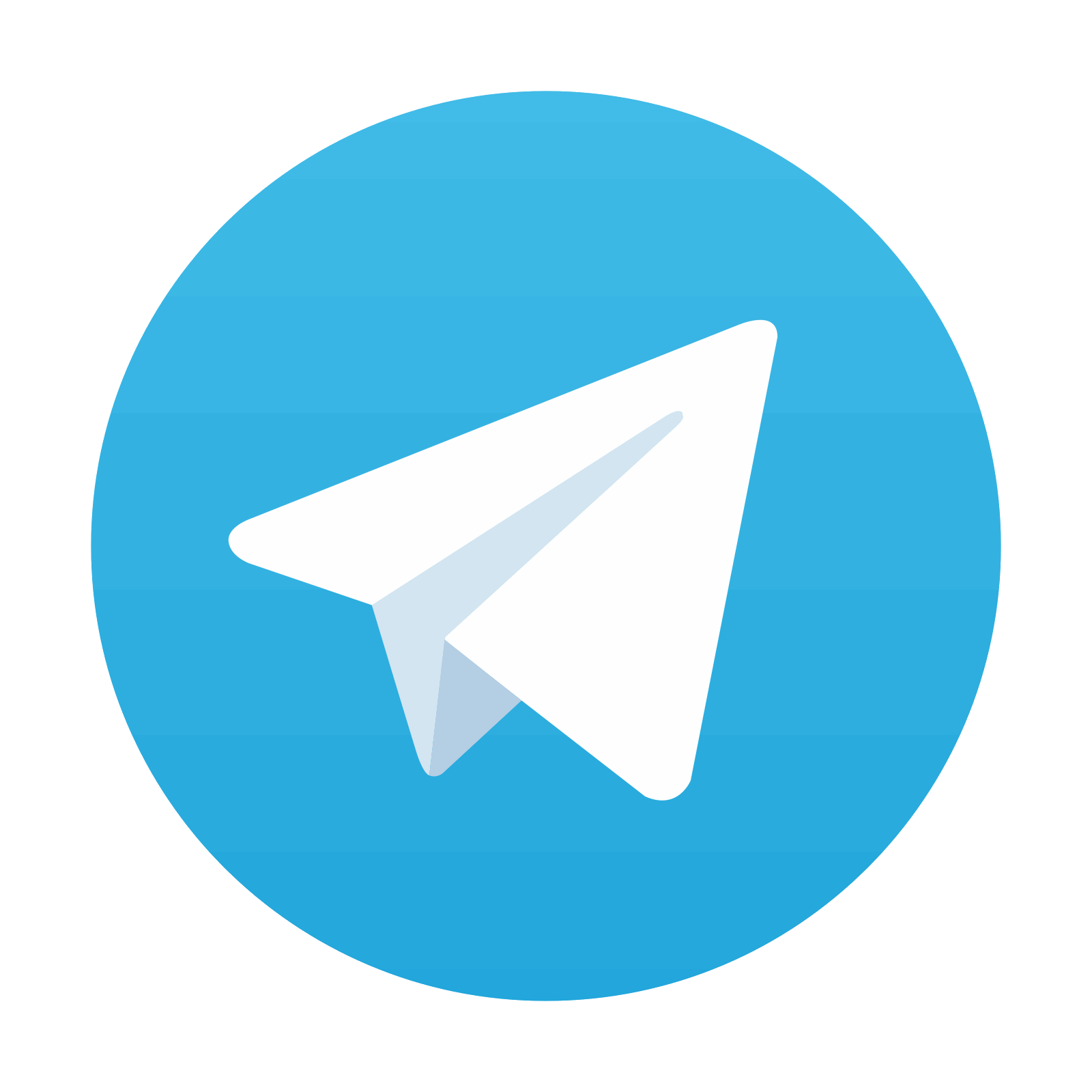
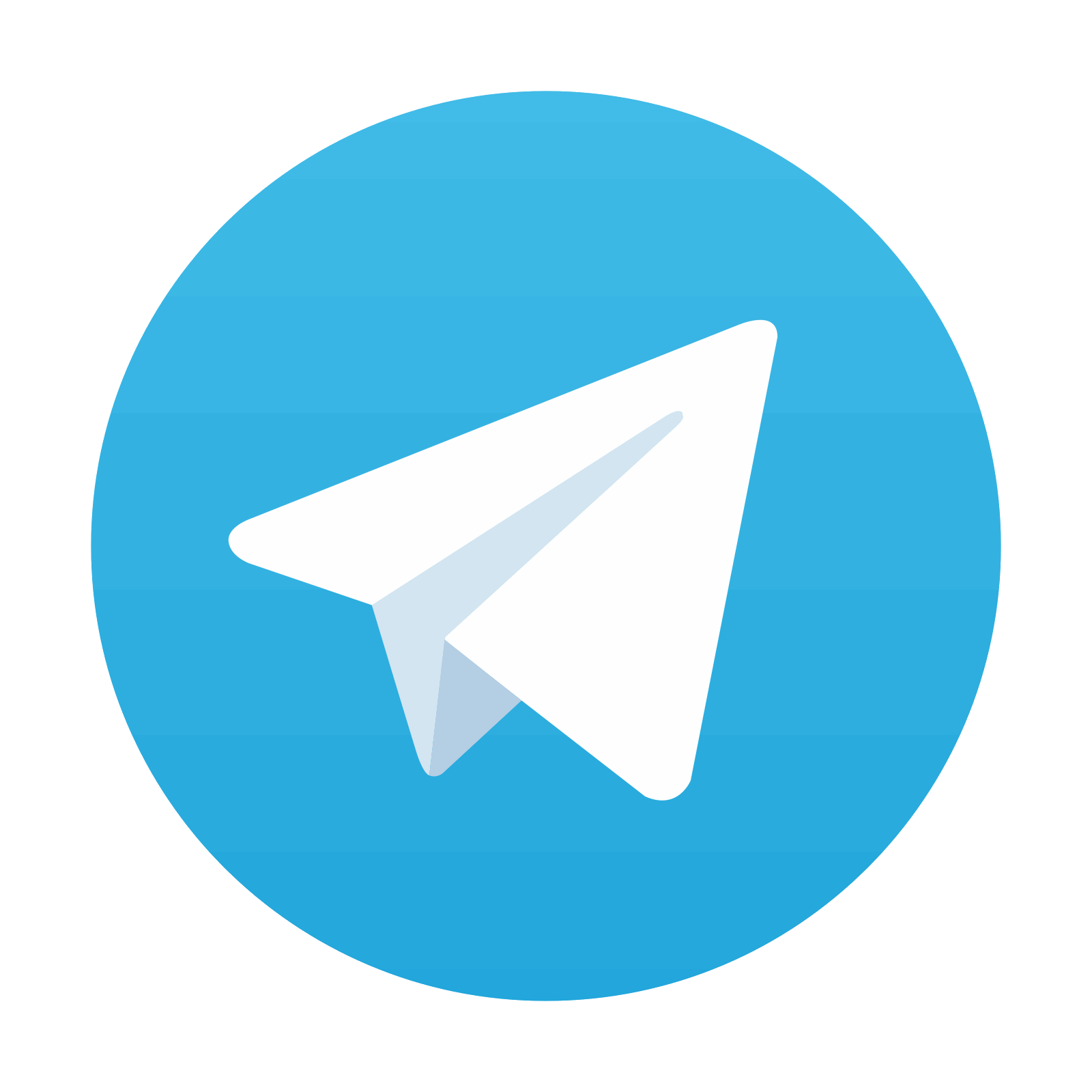
Stay updated, free articles. Join our Telegram channel

Full access? Get Clinical Tree
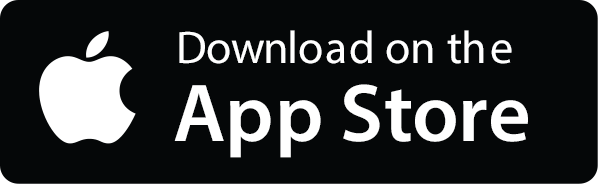
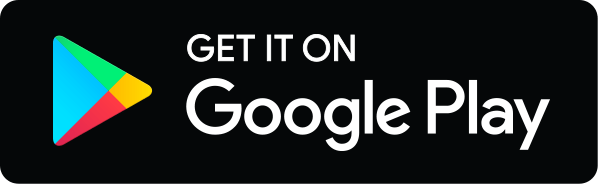