Species
LD50/60 (rad)
Pig
250
Dog
275
Human
350
Monkey
475
Mouse
620
Rat
710
Turtle
1,500
Armadillo
2,000
Alternatives to Cellular Therapeutics
Supportive measures include proper fluid and electrolytes replacement, thyroid protection with potassium iodide, control of seizures, management of nausea, vomiting or diarrhea, and judicious use of sedatives, anxiolytics, and analgesics.
Antibiotics are used as a prophylaxis in neutropenic patients. In non-neutropenic patients, the use of antibiotics is usually reserved for focal infections secondary to burns, penetrating wounds, or visceral trauma. In several animal studies, it has been shown that antibiotics alter the anaerobic gut flora and increase mortality in irradiated animals [8]. Thus, antimicrobial gut prophylaxis is usually administered only to patients with abdominal trauma, Clostridum difficile enterocolitis, or those critically ill in intensive care units [6, 7, 13]. With exposure exceeding two Gy, hematologic support with the use of cytokines and growth factors is often required. Studies on victims of radiation accidents and cancer patients have demonstrated the beneficial effect of these agents on white blood cell recovery in humans [23, 29, 41]. Experimental studies using cytokines in mice, dogs, and non-human primates have also shown improved outcome after sublethal irradiation [15–17, 34, 36, 42]. However, cytokines may accelerate BM recovery only in the case of sublethal irradiation with reversible hematopoietic failure and are most beneficial in patients with an absorbed dose not exceeding five Gy [13]. None of the currently available growth factors or cytokines have formal FDA approval for the treatment of radiation induced BM failure.
Blood Products
Blood products are usually given when a patient presents with severe cytopenia secondary to radiation induced BM aplasia and for those with anemia resulting from gastrointestinal bleeding. Mature red blood cell and platelets do not contain cell nuclei, thus they are relatively resistant to radiation. Severe anemia and thrombocytopenia do not typically occur within the first week following radiation exposure and finding a matched blood donor is usually not a problem. Ideally, all blood products should be irradiated and leukoreduced to prevent transfusion-associated graft-versus-host disease (TA-GVHD). TA-GVHD is a severe form of GVHD that results from transplanting viable allogeneic lymphocytes to the immunocompromised host. It is almost uniformly fatal; thus, caregivers should be aware of potential risks and all available preventive measure should be taken. Leukoreduction also decreases the risk of febrile nonhemolytic reactions and the immunosuppressive effect of transfused allogeneic blood products [5, 22]. It also has some preventive properties against platelet alloimunization and cytomegalovirus infection [32]. Guidelines for transfusion of red blood cells and platelets in patients presenting acute radiation syndrome usually follow those recommended for patients receiving chemotherapy [2, 9, 40, 46].
Hematopoietic Stem Cell Transplantation
Rapid proliferation of hematopoietic stem cells (HSC) and progenitor cells increases their susceptibility to ARS induced damage. Transplantation of human- leukocyte-antigen (HLA) -matched BM or autologous BM could be an ideal solution to mitigate the suppression of irradiated patient immune system. Transplantation of the whole BM containing both hematopoietic and mesenchymal stem cells (MSC) could effectively facilitate not only the reconstitution of patient immune system, but also other systematic damages caused by radiation exposure. Traditionally used sources of HSC and progenitor cells include BM, mobilized peripheral blood, and cord blood. Experimental studies performed in the field of cellular therapies and transplantation are introducing other sources of stem cells such as adipose tissue derived stem cells (ASC) [4, 10, 19]. In the future, induced pluripotent stem cells (iPSC) could also become one of the sources of supportive therapy for ARS victims [14, 44]. Application of these cell sources in clinical scenario is unlikely at the present due to the time requirement for cell culturing and conditioning. With the technological advances and further discoveries in this field, both ASC and iPSC may become feasible sources of stem cells in the future. The information describing treatment procedures for ARS patients is obscure and based mostly on data gathered from leukemia patients, as well as ARS victims of industrial or military incidents who received different amounts of radiation, presented various severity of ARS, and obtained different types of supportive treatment, as well as HSC transplant from different sources. Acquiring autologous or HLA-match HSC for transplantation is very difficult due to the unexpected nature of irradiation exposure. In these situations, allogenic (not-fully matched) HSC from BM or cord blood of unrelated donor is used. Transplantation of allogenic HSC remains a controversial treatment option and is recommended only for patients with “documented lack of spontaneous recovery and/or lack of response following 2–3 weeks of cytokine treatment” and without “aplasia and significant injury to a nonhemopoietic organ system” [13]. In the past, the reported outcomes among patients who received HSC transplant were poor [13]. The risk associated with development of graft-versus-host disease (GVHD) significantly increases with application of allogenic HSC. GVHD, as well as conditioning regimens necessary for engraftment of allogenic HSC, introduces additional inflammation-induced damage to non-hematopoietic tissues and also jeopardizes any chance for ARS victim organism recovery. New methods for reducing the incidence and severity of GVDH are being studied. Since the discovery of mesenchymal stem cells (MSC) and their immunomodulatory properties, such as expression of transforming growth factor-beta (TGF-β), prostaglandin E2 and indoleamine 2, 3-dioxygenase, MSC has become an object of interest [3, 33]. Preclinical and clinical studies are currently testing the effect of MSC in leukemia patients [26, 28] as well as reduction of GVHD following BM transplant [39, 47]. Clinical trials using MSCs for treatment of severe, steroid-resistant GVHD have shown promising results [27, 38].
The effect of MSC on the recipient is not only restricted to facilitation of cytokine environment targeting T-cell proliferation and activation, but it can also restore BM stroma, which when severely damaged in ARS patients impedes HSC engraftment and proliferation [3, 18, 21]. Generalized inflammatory response and cytokine release following radiation exposure may additionally stimulate transplanted MSC to migration and restoration of minor tissue injuries created by irradiation. Several in vivo studies confirmed the ability of MSC to engraft into the radiation damage sites such as brain, heart, BM, and muscles [20, 31]. The migratory and regenerative effects of MSC are even more obvious when localized irradiation of the abdomen and leg was applied and enhanced engraftment of MSC was administered to the exposed areas [20, 31, 43]. To utilize the immunomodulatory and regenerative properties of MSC [25] co-transplantation of MSC as a supportive therapy to improve engraftment of HSCs has been proposed. Studies performed in NOD/SCID mice presented improved engraftment of human HSC following co-infusion [30, 35, 37]. This promising result was also confirmed in acute radiation syndrome (ARS) model [43]. Co-transplantation of MSC and HSC could be successfully introduced to clinical settings in the future to treat radiation-induced multi-organ failure syndrome [11, 45].
Ex Vivo Created Fused Chimeric Cells
Situations such as nuclear power plant accidents or hostile nuclear attacks are rare; however under these circumstances thousands of ARS victims will require immediate medical attention. The system used currently (close relative HLA – matched transplantation or unrelated donor from Bone Marrow Registry) will not be viable because family members may also be affected by irradiation and unrelated donor stem cell registries have a limited number of stem cell donor candidates. The development of new therapies that could be applied to treat mass casualties suffering from detrimental effects of radiation exposure is of great importance.
Due to the disadvantages of the currently used HSC transplantation procedures such as matching difficulty, the risk of GVHD, and toxic immunosuppression, Siemionow’s team introduced the use of a novel donor-recipient chimeric cell (DRCC) therapy for the custom treatment of individuals suffering from ARS [24]. This approach is based on the phenomenon of chimerism, which is defined as the presence of donor derived cells in the recipient. The development of the concept of mixed chimerism, studies supporting it, and the involvement of Siemionow’s team in the research testing application of hematopoietic cells for mixed chimerism for tolerance induction in vascularized composite allotransplantion (VCA) are presented in detail in Chap. 71 of this book entitled: In Vivo Chimera Model – Creation of Primary and Secondary Chimera. Briefly, Siemionow’s group observed spontaneous creation of donor-recipient chimeric cells (DRCC) following BM transplantation in allogenic or semi-allogenic models under a seven days immunosuppressive protocol. Detected DRCC presented on their surface MHC antigens characteristic for both transplant donor and recipient. BM transplantation in combination with short-term immunosuppression treatment created an environment facilitating the creation of DRCC, which improved chimerism induction and prolonged VCA survival [12]. Siemionow’s group hypothesized that it could be created as a result of two processes: trogocytosis (cell surface membrane transfer) or cell fusion. Next, ex vivo method of creating DRCC by cell fusion of BM from fully MHC-mismatched donor and recipient was successfully developed and tested in vivo with positive results (described in Chap. 72 entitled: Cellular therapy models: Ex vivo chimera model by cell fusion). Based on the collected data, Siemionow’s group hypothesized that the use of DRCC therapy for the reconstitution of BM compartments will effectively replace the damaged hematopoietic stem cell lineage and will create a bridging therapy for ARS patients. DRCC will propagate and replace damaged BM after radiation exposure without the need for toxic immunosuppression. To test this hypothesis, 10–12 × 106 of ex-vivo created DRCC were used to reconstitute rat BM compartment exposed to sublethal dose of total body γ-irradiation of seven Gy (Fig. 75.1). DRCC therapy was delivered into the femoral bone at six hours following TBI. DRCC were created by ex-vivo fusion of BM cells derived from fully MHC mismatched LEW (RT1l) and ACI (RT1a) donors. All animals received supportive treatment with prophylactic antibiotics and fluids during the follow-up. Preliminary results of this experiment showed that transplantation of DRCC after non-fractionated, single high dose radiation exposure proved to be more effective rescue therapy against ARS compared to allogenic BM transplantation, as confirmed by 100 % recipient survival and expedited recovery of the hematopoietic system without GVHD development [24]. Differential counts performed by hematologic analysis confirmed regenerative effect of DRCC on both the lymphocyte and polymorphonuclear cell lineages [24]. Siemionow’s laboratory’s novel approach of DRCC therapy may act as a bridging therapy supporting hematopoietic recovery and ameliorating injuries in ARS patients, which do not require toxic immunosuppression. In addition, this DRCC therapy will be technically feasible and more economical than current treatments.
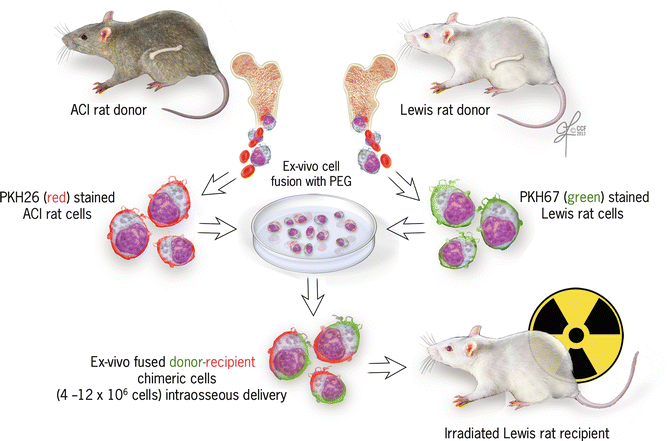
Get Clinical Tree app for offline access
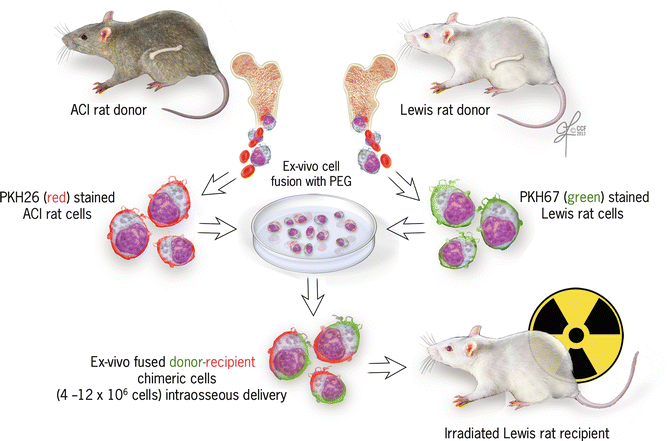
Fig. 75.1
Ex vivo creation of donor-recipient chimeric cells (DRCC) for the treatment of acute radiation syndrome. This novel experimental approach is currently tested on rat model and uses bone marrow derived DRCC; however in the future, stem cells from different sources such as cord blood or adipose tissue might be also utilized. DRCC were created ex vivo by the chemical polyethylene glycol (PEG) induced cell fusion of the BM cells harvested from the ACI (RT1a) and Lewis (RT1l) rat donors. Isolated BM cells were separately stained with two different (red/orange and green) fluorescent dyes. Next, the ex vivo
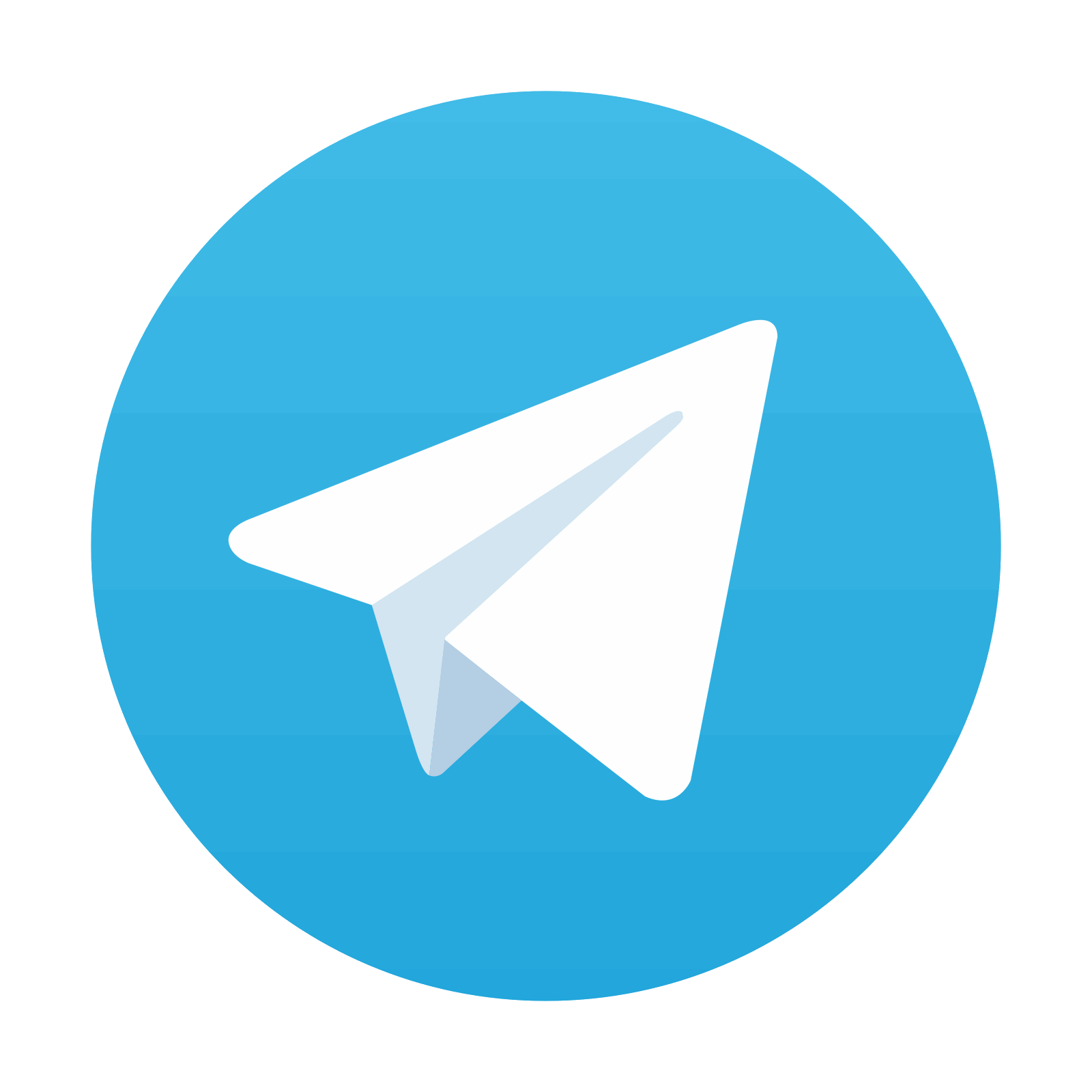
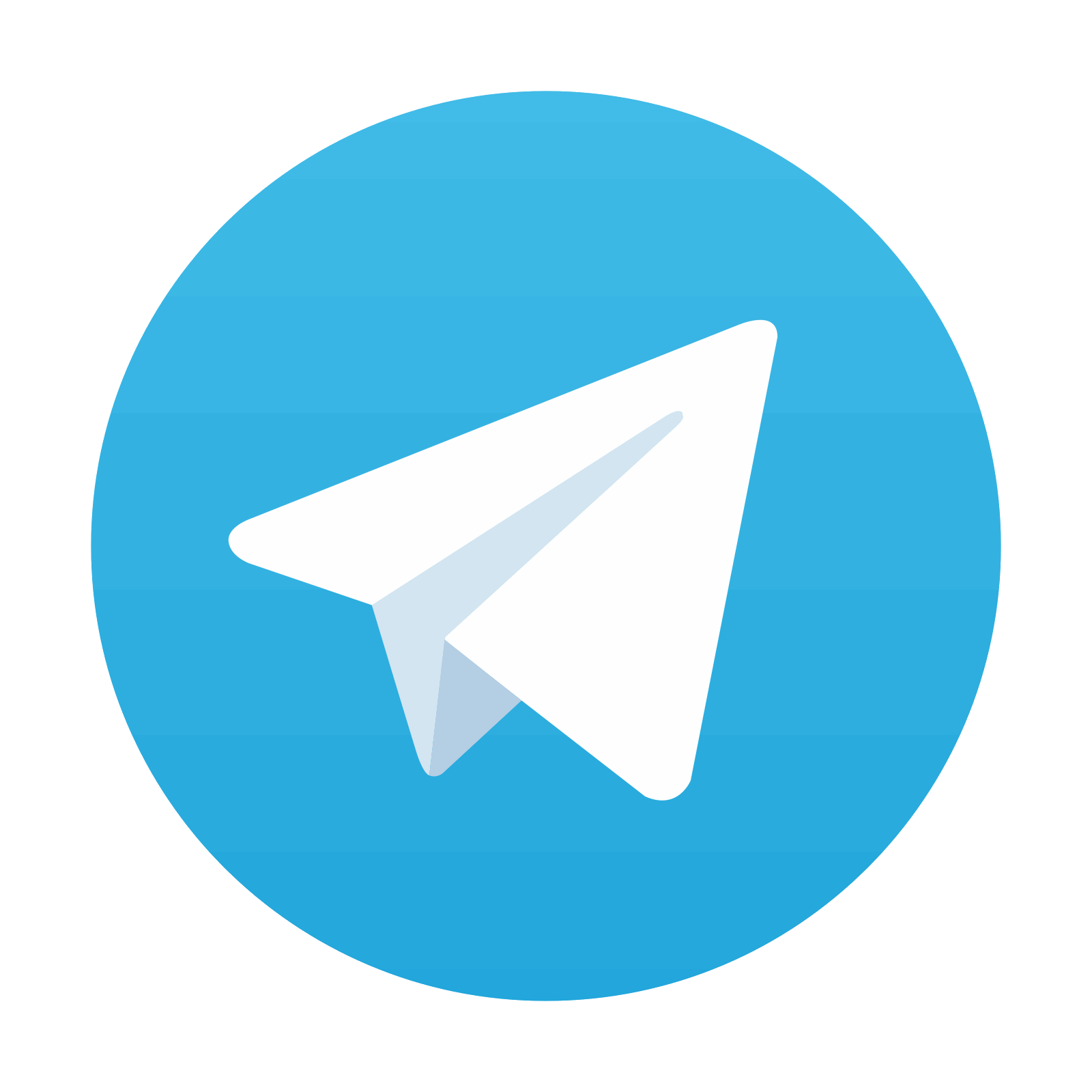
Stay updated, free articles. Join our Telegram channel

Full access? Get Clinical Tree
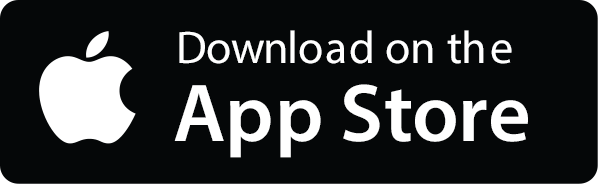
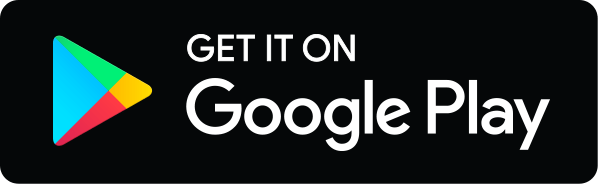
