(1)
Professor of Plastic Surgery, Director of Diabetic Wound Center, Director of Cell Therapy Laboratory, Korea University College of Medicine and Korea University Guro Hospital, Seoul, Republic of Korea (South Korea)
Abstract
The development of advanced wound healing technology has triggered the use of cells to overcome limitations of the conventional methods. Cell therapy has a potential to improve wound healing conditions without major surgical procedures and donor site morbidity. Cell therapy can be applied for both acute and chronic wounds. In the treatment of acute wounds, cell therapy can increase wound healing rate, reduce scar contracture, and minimize donor site morbidity. In the treatment of chronic wounds, by transplanting cells with an excellent profile of wound healing capacity to the wound, attempts are made to convert the wound bed into the environment where maximum wound healing can be achieved.
At present, fibroblasts, keratinocytes, adipose-derived stromal vascular fraction cells, and platelet concentrates are actively used in the clinical setting. In addition, mesenchymal stem cells have been drawing intense attention in the field of wound healing and may hold great promise in treating chronic wounds. The aim of this chapter is to provide information on cell-based treatment options in clinical setting for wound healing. In particular, healing of chronic diabetic wounds will be focused on.
Keywords
Cell therapyWoundDiabetic footThe art and science of wound healing are complex and intriguing. A variety of methods have been used for wound healing. These include healing by primary intention, secondary intention, tertiary intention, skin grafts, and flaps. Each conventional repair method has advantages and limitations. Recently, the development of advanced wound healing technology has triggered the use of cells to overcome limitations of the conventional methods. Cell therapy has a potential to improve wound healing conditions without major surgical procedures and donor site morbidity. Cell therapy can be applied for both acute and chronic wounds.
In the treatment of acute wounds, cell therapy can increase wound healing rate, reduce scar contracture, and minimize donor site morbidity. In particular, cell therapy can be useful for elderly patients in whom skin grafting or flap coverage can be a burden due to surgical procedures or patients who do not want to undergo major surgery. This method also provides a tissue that is similar to the surrounding skin, leaving minimal scars and color mismatch. Since the epidermal portion can be restored by epithelialization induced by the migration and proliferation of adjacent epidermal cells including melanocytes, the density and activity of melanocytes as well as precursor melanocytes of the epidermis of the graft become similar to those observed in the adjacent skin. In addition, this technique is simple and less time consuming and reduces the surgical burden for patients (Chap. 4).
In the treatment of chronic wounds, attempts are made to convert the wound bed into the environment where maximum wound healing can be achieved by transplanting cells with an excellent wound healing capacity to the wound bed. In cases of chronic wounds, wound healing cannot often be achieved successfully because of the involvement of multiple factors. One of the main contributing factors for the nonhealing or delayed healing wound is decreased function of the key cells for the wound healing including fibroblasts and keratinocytes. In chronic wound patients, these cells have notably decreased mitotic activity compared with normal healthy controls. Besides, there is an insufficient degree of synthesis of extracellular matrices (ECMs) and growth factors that are essential for wound healing. Conversely, secretion of matrix metalloproteinase which destroys tissue proteins becomes activated. The degree of cell migration is also decreased. Therefore, the wound remains open without being cured for long periods of time. This eventually leads to infections. It would therefore be mandatory to activate these cells during treatment. By transplanting cells with an excellent profile of wound healing capacity to the wound bed (the term may sound grand, but this method actually refers to the attachment or spraying the cells to the wound bed), attempts are made to convert the wound bed into the environment where maximum wound healing can be achieved.
Both autologous and allogenic cells can be used for wound healing. Cell therapy using autologous cells accelerates the wound healing process by reducing the time needed for the host cells to invade the wound tissue and by early synthesis of new skin. Fairly extensive previous research demonstrated that graft of autologous cells accelerates the rate of wound healing. Cell therapy using allogenic cells has also impressive therapeutic value in wound healing. Initially there was some confusion as to how the wound healing effects of allogenic cells were exerted. It became clear that allogenic cells do not attach and cover the wound permanently and are soon replaced by host cells. However, allogenic cells promote migration and proliferation of host cells from the wound beds and edges because allogenic cells release not only growth factors but also ECMs and basement membrane components. Eventually, the role of allogenic cells in the wound healing process is to accelerate epithelialization from the wound edges and promote granulation formation from wound beds.
At present, fibroblasts, keratinocytes, adipose-derived stromal vascular fraction (SVF) cells, and platelet concentrates are actively used in the clinical setting. The aim of this chapter is to provide information on cell-based treatment options in clinical setting for wound healing. In particular, healing of chronic diabetic wounds will be focused on.
Fibroblasts
Fresh Fibroblast Autograft
A cell therapy product comprising of autologous cultured fibroblasts and a scaffold made from the benzyl ester of hyaluronic acid (Hyalograft 3D; ChaBio & Diostech, Seoul, Korea) has been developed for the treatment of diabetic foot ulcers.
Hyaluronic acid is a naturally occurring biopolymer whose molecular structure is highly conserved between mammalian species. First described in 1934, it has since been used across a wide variety of medical fields as diverse as neurosurgery and cutaneous wound healing. The benzyl ester of hyaluronic acid is an ideal material for wound healing, as it is biocompatible and resorbable and integrates with ulcer tissues.
Fibroblasts transplanted onto the wound secrete various cytokines and growth factors that control cell proliferation, induce angiogenesis, and modify the inflammatory process. They also produce three-dimensional extracellular matrices comprising collagens, proteoglycans, and other proteins.
Application Method
A skin biopsy (1–2 cm2) is performed on a patient. Autologous dermal fibroblasts are isolated and cultured. Once the fibroblast number reaches 5 × 106, the cells are then seeded on a distinct biodegradable scaffold composed entirely of a benzylic ester of hyaluronic acid, which is a three-dimensional nonwoven scaffold. Starting on day 8 from seeding, the fibroblast–hyaluronic acid complex is ready for transplantation. The autologous fibroblast–hyaluronic acid complex is grafted onto the debrided and cleansed wound and covered with a polyurethane foam dressing (Fig. 10.1). The graft and the polyurethane foam are left on the wound for 7 days.


Fig. 10.1
(A) A skin biopsy is taken from a patient. (B) The autologous cultured dermal fibroblast–hyaluronic acid complex is ready for transplantation. (C) The autologous fibroblast–hyaluronic acid complex is tailed according to the wound size and shape. (D) The fibroblast complex is grafted onto the debrided and cleansed wound bed
Clinical Study
Fifty-four patients with diabetic foot ulcers were screened and included in the author’s clinical trial. Of these 54 patients, 28 were assigned to the treatment group, and 26 were assigned to the control group. Patients in the control group were treated with a polyurethane foam dressing without the fibroblast–hyaluronic acid complex. Control group visits and dressing changes were scheduled as in the treatment group. There were no statistically significant differences with respect to the clinical characteristics between the two groups. All patients attended weekly visits for 12 weeks or until the ulcer healed. The primary efficacy criterion was the percentage of patients who achieved complete wound closure within the 12-week study period. The secondary efficacy criterion was the mean time required for complete closure in patients who achieved complete healing within 12 weeks. Complete closure was defined as the completely epithelialized state in the absence of any discharge which allowed the patient to shower.
After 12 weeks, complete wound healing occurred in 88 % in the autologous fibroblast–hyaluronic acid complex-treated group and in 36 % in the control group (p < 0.01). The time required for complete healing ranged from 2 to 10 weeks (mean, 4.91 ± 2.20 weeks) in the autologous fibroblast–hyaluronic acid complex-treated group and from 4 to 11 weeks (mean, 6.63 ± 2.20 weeks) in the control group (p = 0.063). The Kaplan–Meier mean healing time to complete ulcer healing was 6 weeks for the treatment group and 10 weeks for the control group.
The safety and tolerability were also excellent; there were no adverse effects related to the autologous fibroblast–hyaluronic acid complex treatment. No clinically meaningful changes occurred in the baseline values of clinical laboratory parameters, including serum chemistry, hematology, and urinalysis, or vital signs for any of the patients (Figs. 10.2, 10.3 and 10.4).




Fig. 10.2
A Kaplan–Meier diagram showing the results of time to wound closure

Fig. 10.3
A case of the control group. (A) Initial view. (B–F) Two, 4, 6, 8, and 10 weeks after foam dressing. (G) At twelve weeks after foam dressing, the ulcer did not heal

Fig. 10.4
A case of the fibroblast group which had similar baseline characteristics of the wound to Fig. 10.3. (A) Initial view. (B, C) Two and four weeks after grafting of autologous fibroblasts. (D) Six weeks after grafting, complete wound closure was achieved
Fresh Fibroblast Allograft
Fibroblasts isolated from diabetic foot ulcers exhibit a marked decrease in cellular activity. More specifically, fibroblasts from diabetic foot ulcers have been shown to display lower proliferative potential and attenuated growth factor production than fibroblasts derived from healthy tissues. In addition, there is an excess of metalloproteinases and a decrease in the tissue inhibitors of metalloproteinases. These conditions can lead to a breakdown of growth factors and ECM components, such as fibronectin.
There is considerable interest in the treatment of diabetic ulcers with grafts of cultured keratinocytes and/or fibroblasts. These technologies, some of which are commercially available, are able to adjust a wound’s environment and provide desired growth factors and other substances that may be lacking in a diabetic wound, therefore representing an exciting development and a major advance. However, the effect has generally shown to be not very dramatic. One of the possible reasons for the limited effect of these products is that most cell therapy products are composed of cryopreserved allogenic cells. It is difficult for grafted frozen cells to recover, colonize, and persist in chronic wound beds which are deficient in oxygen and nutrients. Moreover, cell activities are impaired due to cryopreservation. Generally, cells in cryopreserved cultures show about 50 % viability compared to non-frozen cells, and protein synthesis is inhibited by 70–98 %. In terms of growth factor expression and angiogenesis, there is an even lower rate of recovery.
To overcome the limitations of such cryopreserved cell allograft techniques, the author has developed a non-cryopreserved fresh fibroblast allograft method and reported promising results. Cultured fibroblast allografts can improve the overall environment of problematic wounds. Fibroblasts transplanted onto the wounds secrete various cytokines and growth factors that control cell proliferation, induce angiogenesis, and modify the inflammatory process. They also produce three-dimensional extracellular matrices comprised of collagen, proteoglycans, and other proteins.
Application Method
Allogenic Dermal Fibroblast Culture
Freshly discarded, healthy skin tissues from healthy donors are deepithelialized and minced into pieces of 2 × 1 mm in size. Blood samples from all donors are tested for infectious diseases, including hepatitis, HIV, and syphilis. The tissues are spread evenly over the surface of 100 mm tissue culture plates and coated with 3 ml of Dulbecco’s modified Eagle medium/Ham’s F-12 (DMEM/F-12) containing 50 % autologous serum. The plates are incubated at 37°C for 4 h to facilitate tissue adhesion to the plates. After incubation, 12 ml of DMEM/F-12 containing 10 % autologous serum and 25 μg/ml gentamicin is added, and the plates are returned to the incubator. All cultures are grown at 37°C in a 5 % CO2/95 % air atmosphere. The cultured fibroblasts are trypsinized and then filtered through a nylon mesh. The dissociated cells are diluted 2.7-fold with Dulbecco’s phosphate buffered saline without Mg2+ and Ca2+ (DPBS) and collected by centrifugation at 450x g for 17 min. The cells are washed twice in 40 ml of DPBS. Cell density is determined using a hematocytometer, and viability is assessed by trypan blue exclusion. Second or third passage cultures are used for allografts. Approximately 3 weeks are required to prepare the fibroblast allograft material from the source skin graft material.
Technique
Patients are screened for eligibility before applying the fibroblast allograft. During the screening period, patients undergo debridement as necessary to remove nonviable tissue. The area of the debrided ulcer is determined by the Digital Wound Measurement System. After the fresh cultured fibroblasts are suspended in 0.3–0.7 ml of commercially available fibrinogen in a 50 ml centrifuge tube, they are transferred to a plastic syringe and dispersed onto the debrided wound. The liquid fibroblast allograft is then sealed using 0.2–1.0 ml of commercially available thrombin according to the wound size (Fig. 10.5). Film dressing is applied to the graft site and then changed 5 days later.


Fig. 10.5
Obtaining fresh allogenic fibroblasts. (A–C) Freshly discarded, healthy skin tissue from a healthy donor is deepithelialized and minced into pieces of 2 × 1 mm in size. Dermal tissue is spread evenly over the surface of 100 mm tissue culture plates. (D) Dermal fibroblasts on a culture plate (X100). (E) The collected dermal fibroblasts in a 50 ml centrifuge tube. (F) The fresh fibroblasts are spread over a wound bed
The patients return every 3–7 days for wound examination and dressing changes. The dressing is kept moist until complete wound closure is achieved. All patients with ulcers on weight-bearing sites, or sites otherwise receiving pressure when wearing shoes, have pressure off-loading by the use of foam dressings with a hole on the ulcer site and footwear with cushioning insoles. If clinically appropriate, the use of other weight off-loading devices such as crutches, half-shoes or half-sandals, and wheelchairs should be applied.
Clinical Study
A total of 55 patients with diabetic foot ulcers were included in the author’s research. Among them, 37 patients were treated with fibroblast allografts. The mean patient age was 63.9 ± 8.2 years, and the ulcer size ranged between 2.0 and 7.2 cm2 (mean area, 4.6 ± 1.7 cm2) with a duration of 6–30 weeks (mean, 13.2 ± 5.5 weeks). Twenty patients had ulcers with exposed bones. Nineteen patients had dorsal ulcers located on the forefoot (8), heel (2), or toe (9), and 18 patients had plantar ulcers on the forefoot (9), heel (5), or toe (4).
For control treatment, only fibrinogen and thrombin, without cells, were topically applied over the wound area of 18 patients. All treatment conditions, including wound management, were set up to be identical to those used for the fresh fibroblast treatment group. The mean age of the control group was 59.8 ± 5.8 years, and the wound size ranged from 1.5 to 7.0 cm2 (mean, 4.3 ± 1.9 cm2) with a duration of 6–24 weeks (mean, 12.4 ± 5.1 weeks). Eight patients had bone-exposed ulcers. Nine patients had dorsal ulcers located on the forefoot (5) or toe (4), and the other 9 patients had plantar ulcers on the forefoot (5), heel (1), or toe (3).
The efficacy, safety, and tolerability of fibroblast allograft treatment were excellent. After 8 weeks, complete wound closure was achieved in 31 patients (83.8 %) in the treatment group and 9 patients (50 %) in the control group (p < 0.05). The time required for complete healing ranged from 17 to 56 days following treatment (mean, 30.9 ± 10.1 days) in the allograft group and from 33 to 56 days (mean, 47.2 ± 7.8 days) in the control group (p < 0.05).
Patient satisfaction was also very positive. The patients showed better results for fresh fibroblast application than for the conventional method (mean scores of 8.0 ± 1.0 and 4.9 ± 1.4, respectively, p < 0.05).
The safety and tolerability were also excellent; there was no adverse effects related to fibroblast allograft treatment. The grafted areas did not exhibit any clinical signs of graft rejection, and no clinically meaningful changes occurred from the baseline values of clinical laboratory parameters (including serum chemistry, hematology, and urinalysis) or vital signs in any of the patients (Figs. 10.6, 10.7, 10.8 and 10.9).





Fig. 10.6
A woman (age 54) with a chronic nonhealing diabetic ulcer over 10 weeks old on the medial side of the left fourth toe. (A) Preoperative view of the bone-exposed, 2.5 cm2 sized wound. After debridement, freshly bleeding wound bed was prepared. (B) Application of fresh allogenic fibroblasts. (C) Ten days after treatment. (D) Seventeen days after treatment. Epithelialization was complete

Fig. 10.7
A woman (age 50) with 6-week-old chronic nonhealing diabetic ulcers on the left second and third toe tips. The second toe with the larger wound was treated with the fibroblast allograft, and the third toe with the smaller wound was conservatively treated. (A) Preoperative view. (B) Immediate after treatments. (C) Two weeks after treatments. (D) Twenty days after treatments, the second toe wound completely epithelialized, but the third toe wound (control) had a raw surface. (E) Three months after treatments, the second toe was good with a healthy texture, but the third toe had not yet completely epithelialized

Fig. 10.8
(A) A fibroblast–fibrinogen mixture and thrombin were spread on the debrided wound using a plastic syringe. (B) Twenty-two days after treatment, epithelialization was complete

Fig. 10.9
(A) Preoperative view. (B) Immediate after debridement. (C) Immediate after application of the fresh fibroblast allograft. (D) Thirty days after treatment, complete wound closure was achieved. (E) At the 40-month follow-up, the affected site had a healthy texture
Keratinocytes
Keratinocyte Autograft
Green et al. succeeded in culturing keratinocytes in 1975. Treatment using cultured autologous keratinocytes was first applied to a burn patient in 1981. Over the past three decades, cultured keratinocytes have been used as autografts or allografts to cover various wounds.
Autologous keratinocytes should become an integral part of the management of patients with large burn wounds and patients with limited donor site availability. This treatment can minimize donor site morbidity when the patient needs a skin graft because it is possible to culture a large sheet of the patient’s epidermis from only a small piece of skin. For example, a commercialized product (Holoderm®; Tego Science, Seoul, Korea) is composed of approximately 1 billion keratinocytes. A skin biopsy as small as 1–3 cm2 can be expanded 10,000 times in about 14–18 days to cover an entire adult body.
However, skin biopsy is necessary from a patient, and patients need to wait for the cells to be processed for this strategy. Therefore, the keratinocyte allograft can be more useful for the treatment of chronic wounds.
Keratinocyte Allograft
Recently, a biologic dressing material derived from cultured keratinocytes of a Korean infant’s foreskin (Kaloderm®; Tego Science) has newly been developed. A sheet of the cultured allogenic keratinocytes contains >2 × 107 cells backed by Vaseline gauze.
Keratinocytes are known to enhance wound healing by secreting and synthesizing many growth factors and major components of the basement membrane. Allogenic keratinocytes do not attach and cover the ulcer permanently and are soon replaced by host keratinocytes. However, allogenic keratinocytes promote migration and proliferation of host keratinocytes from the wound edges or remaining hair follicles because allogenic keratinocytes release not only growth factors (TGF-α, PDGF, bFGF, VEGF, and TGF-β) and cytokines (IL-1, IL-6, IL-8, and IL-10) but also extracellular matrices (fibronectin and laminin) and basement membrane components. Eventually, the role of allogenic keratinocytes in the healing process is to accelerate epithelialization from the wound edges and promote granulation formation.
Using the cultured allogenic keratinocytes for the treatment of diabetic foot ulcers has the following advantages. First, cultured keratinocytes are derived from an unrelated allogenic donor and may be stockpiled. Cell Bank enables a large-scale production, and keratinocyte sheets can be stored at −70 °C and be stable for 24 months. This will make keratinocyte sheets available immediately and convenient to use for physicians and patients. Unlike autologous cells, biopsy is not necessary, and patients do not need to wait for the cells to be processed. These keratinocyte sheets can be nearly ready-made when the patient arrives at the clinic. Second, cultured human keratinocytes have a direct antimicrobial effect. Maier et al. reported that keratinocytes, usually considered sensitive to bacterial contamination, are able to inhibit bacterial colonization of freshly excised burn wounds and chronic ulcers. Finally, the cultured allogenic keratinocytes do not elicit a significant immunologic response from the host. This lack of rejection may be due to the loss of immunologic properties of cultured keratinocytes. Experimentally, cultured allogenic keratinocytes have been shown to inhibit the response to alloantigen for both native T cells and anti-CD3-activated T cells. This inhibition is thought to be due to soluble factors secreted by keratinocytes.
Improvement in the understanding of wound healing mechanisms and the field of bioengineering have led to the development of various advanced products, such as growth factors and living skin equivalents. The cultured allogenic keratinocyte sheet, which is one such new treatment, may be a safe and effective option for the treatment of nonischemic diabetic foot ulcers without infection.
In this chapter, the author’s experiences with the new cultured allogenic keratinocyte sheet in the management of chronic diabetic foot ulcers are reported.
Application Methods
According to the information provided by the manufacturer, human epidermal keratinocytes are isolated from the foreskin of a Korean newborn and cultured according to the Green and Rheinwald method. Cells at an early passage are cultured to form a sheet for 10 days in a 3:1 mixture of DMEM/F-12 with fetal bovine serum and supplements described elsewhere. The epidermal sheets are chemically detached from the culture vessel, backed with Vaseline gauze, and stored frozen at −70°C according to the manufacturer’s undisclosed protocols.
The ulcer is cleaned with 3 % hydrogen peroxide and saline solution to remove dirt and other debris before applying the keratinocyte sheet. The keratinocyte sheet, which is stored at −70 °C, is thawed at room temperature for 5–10 min and cut to the wound size and applied directly to the wound bed as a primary dressing and then covered with a secondary dressing such as a polyurethane foam (Fig. 10.10).


Fig. 10.10
(A) View of the cultured allogenic keratinocyte sheet. (B) Treatment of a diabetic ulcer using the keratinocyte sheet. (C) A polyurethane foam dressing is applied to the treated wounds. (D) Microscopic view of cultured keratinocytes. (E) Close-up view of the keratinocyte sheet on the wound bed
Dressing changes and application of the keratinocyte sheet are usually scheduled at weekly intervals. If found necessary, the patient returns to the hospital two to three times a week for wound examination and secondary dressing changes. All patients with ulcers on weight-bearing sites or sites otherwise subjected to pressure when wearing shoes have pressure off-loadings with the use of foam dressings with a hole on the ulcer site and footwear with cushioning insoles. If clinically appropriate, other weight off-loading devices, such as crutches, half-shoes, half-sandals, and wheelchairs, are applied
Clinical Study
A stratified, randomized, single-blinded, controlled, multicenter study was carried out involving patients with diabetic foot ulcers who were recruited consecutively from three centers in Korea.
Fifty-nine patients were included in the clinical trial. Of these 59 patients, 27 were assigned to the treatment group, and 32 were assigned to the control group. In the control group, Vaseline gauze was applied as the primary dressing over the wound. Control group visits and dressing changes were scheduled as in the treatment group. The wounds were evaluated weekly until complete wound closure or at the 12th week visit if not completely healed.
Results of per-protocol analysis showed that complete wound healing occurred in all patients (100 %) in the keratinocyte-treated group and 69 % in the control group (p < 0.05, chi-square test and Fisher’s exact test) after 12 weeks. The mean percentages of wound area reduction were 100 ± 0 % and 85 ± 65 % in the treatment and control groups, respectively (p < 0.05, Wilcoxon rank-sum test). The time required for complete healing was 41.5 ± 25.4 days and 42.6 ± 19.4 days in the keratinocyte-treated and control groups, respectively (p = 0.90, unpaired t-test). The Kaplan–Meier median time to complete ulcer healing was 35 days for the treatment group and 57 days for the control group.
Intention-to-treat analysis showed similar results. Complete wound healing occurred in 85 % of the keratinocyte-treated group and 59 % of the control group (p < 0.05, chi-square test and Fisher’s exact test). The mean percentages of wound area reduction were 87 ± 49 % and 74 ± 67 % in the treatment and control groups, respectively (p < 0.05, Wilcoxon rank-sum test). The time required for complete healing was 41.6 ± 26.1 and 43.6 ± 19.4 days in the keratinocyte-treated and control groups, respectively (p = 0.78, unpaired t-test).
There were no adverse events thought to be related to the study dressings, and no significant differences were noted between the two groups (p = 0.67, Pearson’s chi-square test). No clinically meaningful changes occurred from the baseline clinical and laboratory parameters in any of the patients, including serum chemistry, hematology, urinalysis, and vital signs (Figs. 10.11, 10.12, 10.13, 10.14 and 10.15).






Fig. 10.11
A Kaplan–Meier diagram showing the results of time to wound closure

Fig. 10.12
A control group wound which was treated with Vaseline gauze. (A) Preoperative view. (B–K) Wound appearance was taken every week. (L) The wound did not heal even at the 12th week after treatment

Fig. 10.13
A keratinocyte-treated wound which had similar patient and wound characteristics to those of the Fig. 10.12 wound at baseline. (A) Preoperative view. (B–E) Wound appearance was taken every week. (F) The wound completely healed after 5 weeks

Fig. 10.14
A control group wound which was treated with Vaseline gauze. (A) Preoperative view. (B–K) Wound appearance was taken every week. (L) The wound did not heal even at the 12th week after treatment

Fig. 10.15
A keratinocyte-treated wound which had similar patient and wound characteristics to those of the Fig. 10.14 wound at baseline. (A) Preoperative view. (B–D) Wound appearance was taken every week. The wound completely healed after 3 weeks
Adipose-Derived SVF Cells
In order to utilize cultured cells for clinical purposes, Food and Drug Administration (FDA)-approved facilities and techniques are required, which may be an obstacle for clinicians who culture cells in a laboratory dedicated to research. In addition, a lengthy culture period is needed for the application. Fortunately, SVF cells obtained from adipose tissue are relatively easy to harvest in large quantities without culturing of cells.
Autograft of Adipose-Derived SVF Cells
Adipose-derived SVF cells contain heterogeneous cell populations, including fibroblasts, stem cells, endothelial cells, mast cells, pericytes, preadipocytes, smooth muscle cells, and progenitor cells, which are well known to accelerate wound healing. Based on the results of Suga et al., SVF cells contain blood-derived cells (37 %), adipose-derived stem/stromal cells (37 %), endothelial cells (15 %), and other cells (11 %). All these SVF cells grafted on the wound bed could not only stimulate host cells around the wound but also be taken on the wound bed and function independently by providing growth factors and extracellular matrices due to the autologous nature of the cells.
In this section, the method of using uncultured SVF cells for the treatment of diabetic ulcers is described.
Experimental Study
The author performed an in vitro study to determine the effect of cell therapy using uncultured SVF cells on cell proliferation and collagen synthesis of diabetic fibroblasts, which are the major contributing factors in wound healing. Briefly, culture human diabetic fibroblasts were seeded onto culture plates and allowed to attach for 24 h. Thereafter, a polycarbonate culture plate insert with a pore size of 3 μm was placed into the culture plate. Based on the results of a corollary study, the optimal pore size of the microporous polymeric membrane, which acts as a barrier to prevent direct contact between the cells inside the insert and the diabetic fibroblasts in the monolayer culture outside the insert but allows extracellular matrices and cytokines produced by the cells inside the insert to successfully transport to the diabetic fibroblasts and to stimulate them, was 3 μm. Uncultured SVF cells were placed in the culture plate insert. After 3 days of incubation, the inserts and the cells inside were removed. Cell proliferation and production of collagen were measured using the 3-(4,5-dimethylthiazol-2-yl)-2,5-diphynyl tetrazolium bromide assay and the collagen type I carboxy-terminal propeptide enzyme immunoassay, respectively.
The results showed that cell proliferation and collagen synthesis in the SVF cell treatment group were 28 % and 44 % higher than those in the control group, respectively (Chap. 6, Fig. 10.16). In addition, a synergic effect of the SVF cell autograft was observed possibly due to mutual stimulation of the diabetic fibroblasts and SVF cells by growth factors secreted from them.


Fig. 10.16
(A) Liposuction of the adipose tissue. (B) Isolated SVF cells (arrows)
Application Method
Isolation of SVF Cells
Abdominal adipose tissues are obtained from a patient by liposuction using a cannula with a 3-mm inner diameter in order to isolate autogenous SVF cells. The obtained samples are rinsed with Mg2+– and Ca2+-free Dulbecco’s phosphate buffered saline (DPBS) and then incubated in DMEM/F-12 containing 0.075 % type I collagenase for 2 h at 37 °C. The top lipid layer is removed, and the remaining liquid portion is centrifuged at 300 x g for 10 min at 4 °C. The resulting pellet is treated with 160 mM NH4Cl for 10 min to lyse red blood cells. The remaining cells are then washed twice in 40 mL DPBS, resuspended in 5 mL DPBS, and filtered through a 100μm nylon mesh (Fig. 10.16). Cell density is determined by counting using a hemocytometer, and cell viability is assessed using trypan blue exclusion assays.
According to the author’s experience, 6.3 × 104 to 2.2 × 105 SVF viable cells can be isolated per ml of aspirated adipose tissue (Fig. 10.17).


Fig. 10.17
Calcein staining of the isolated SVF cells. Arrows (round sky-blue colored cells) indicate viable SVF cells. According to the author’s experience, 6.3 × 104 to 2.2 × 105 viable SVF cells can be isolated per ml of aspirated adipose tissue
Technique
Patients are screened for eligibility before applying SVF cell autografts. During the screening period, patients receive ongoing debridements as required to remove nonviable tissue.
On the day of cell transplantation, patient’s abdominal adipose tissue is obtained in the operation room. This process is done in the morning, and the patient is dismissed from the operation room. After this, aspirated adipose tissue is processed for autogenous SVF cell isolation in the same way as described above. Once the SVF cells are ready for the graft, the patient returns to the operation room, and the wound is debrided.
After being suspended in commercially available fibrinogen in a 50 ml centrifuge tube, the SVF cells are transferred to a plastic syringe and dispersed onto the debrided wound. The SVF cell autograft is then sealed using commercially available thrombin according to the wound size. Using fibrinogen and thrombin as a cell carrier enables the grafted SVF cells to persist on the wound more effectively. Film dressing is applied to the graft site and then changed 5–7 days later. The patients returns every 3–7 days so that the wound can be examined and the dressing can be changed. The dressing is kept moist until complete wound closure is achieved. All patients with ulcers on weight-bearing sites, or sites otherwise receiving pressure when wearing shoes, have appropriate pressure off-loading (Fig. 10.18).


Fig. 10.18
(A) A plantar ulcer. (B) Trypan blue staining of the isolated SVF cells. (C) SVF cell pellet. (D) Application of SVF cells. (E) Dressing using a polyurethane film and foam. (F) Three weeks after treatment, the wound was successfully closed. (G) Three months after treatment
Clinical Study
Fifty-four patients with diabetic foot ulcers were included in the author’s clinical study. Of the 54 patients, 28 patients were treated using uncultured autologous SVF cells. The ulcer size of the SVF cell-treated group ranged between 1.2 and 7.6 cm2 (mean area, 4.3 ± 2.1 cm2) with a wound duration of 6–30 weeks (mean, 12.5 ± 5.6 weeks). Sixteen patients had ulcers with bone exposure. Control treatment was performed on 26 patients. For control treatment, only fibrinogen and thrombin, without cells, were topically applied over the debrided wounds. The wound size of the control group ranged from 1.4 to 10.0 cm2 (mean, 4.0 ± 2.1 cm2) with a wound duration of 6–24 weeks (mean, 12.5 ± 5.5 weeks). Twelve patients had bone-exposed ulcers.
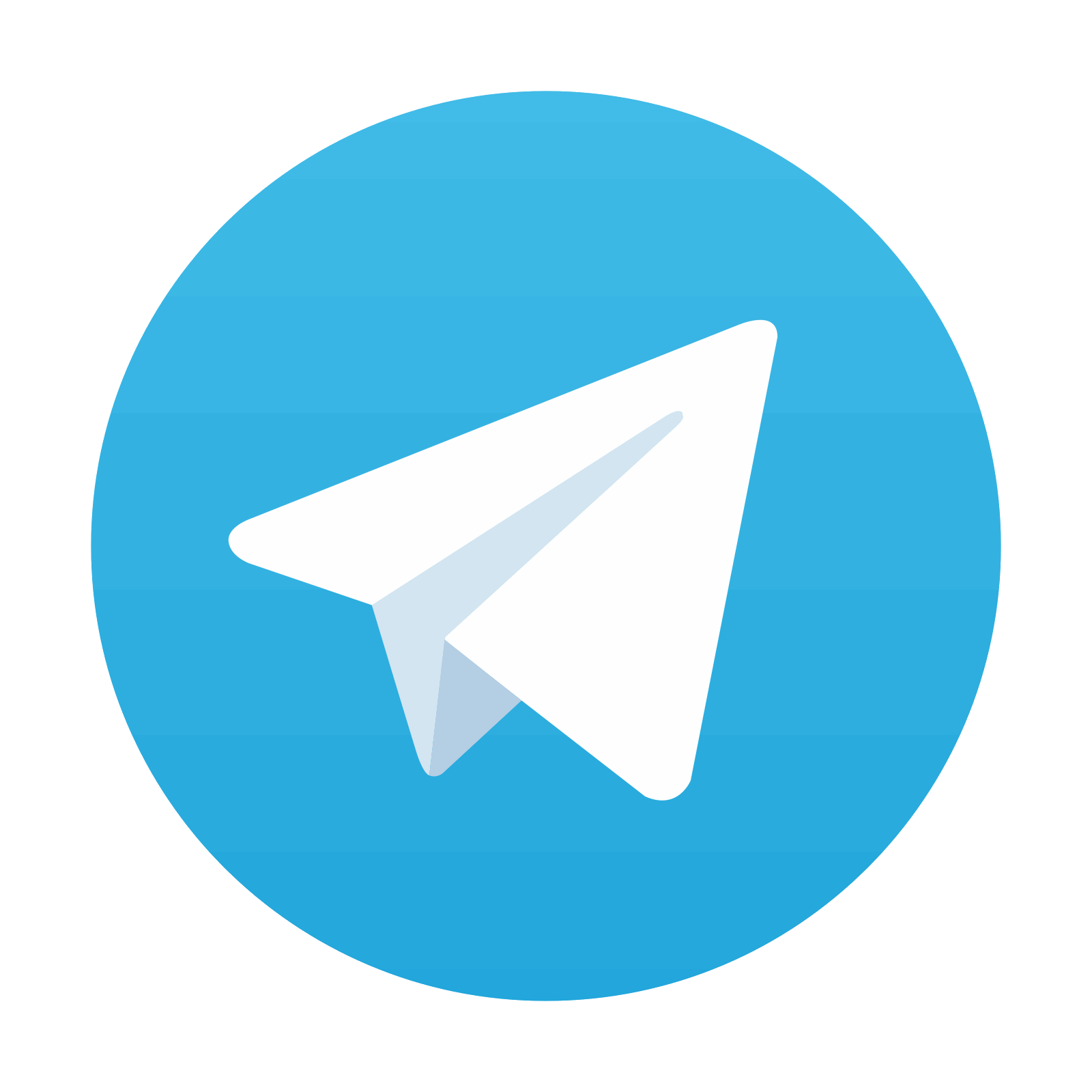
Stay updated, free articles. Join our Telegram channel

Full access? Get Clinical Tree
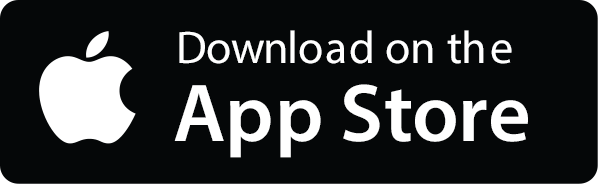
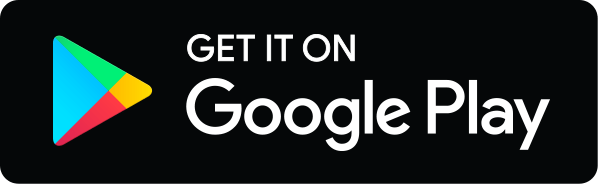
