Fig. 35.1
VASER™ system (Sound Surgical Technology, Denver, CO, USA)
Traditionally, physicians have used handheld syringes, following the Coleman technique. This method is both laborious and time consuming and, depending upon the size of the needle and syringe used, may still disrupt fat cells.
To perform bilateral breast augmentation with AFT, large amounts of harvest material are required (a minimum of 500 mL unfiltered material). In the last 3 years, the authors have used standard VASER™ treatment, reducing the power to 60 % of the total at continuous setting, followed by low-vacuum aspiration using the VentX system and achieved a raw fat viability of 83 %. This high level of viability did not come at the expense of operative time or convenience. With experience, there was an average operating time of 3½ h, including using VASER™ to two to four areas for lipoplasty, fat harvesting, PureGraft® washing and preparation, and fat introduction into both breasts with small syringes. All treatments were performed under tumescent anesthesia and intravenous (IV) sedation. All patients were treated as day case with discharge few hours after surgery. A series of 120 cases, with regular follow-up to 3 years, have been performed.
An important factor in the viability is the use of lower-power VASER™ to emulsify the adipose tissue before aspiration. Separating the adipose tissue into smaller clumps in situ permits the use of atraumatic cannulas and lower vacuum levels, which, in turn, avoids traumatic injury to the fat during the aspiration. Also, by maintaining the overall cellular distribution and ratio of the lipoaspirate (mature adipocytes and the “loosely adherent” cell population (peri-adipocytes, stem cells, stromal cells, etc.), the retention rate of the implanted tissue matrix is enhanced. In addition to the use of harvested fat for immediate re-injection, adipose tissue is a significant source of stem cells for both enhancement of autologous fat grafts and for the use in often developing clinical treatments. As with direct autologous fat injection, the viability of the regenerative cells derived from the harvested adipose tissue is of primary importance, both as fresh tissue and as tissue after freezing.
The field of clinical treatments using adult stem cells continues to expand, and patients undergoing liposuction are increasingly interested in storing tissue samples containing stem cells.
The tissue could then be thawed at some future date and utilized in the development of genetic and regenerative treatments. The viability of the adipose-derived stem cells was higher than that for the overall tissue samples at over 87 % (Fig. 35.2). Even more importantly, the viability of the adipose-derived stem cells was only slightly reduced to 75 % after freezing using liquid nitrogen vapor.
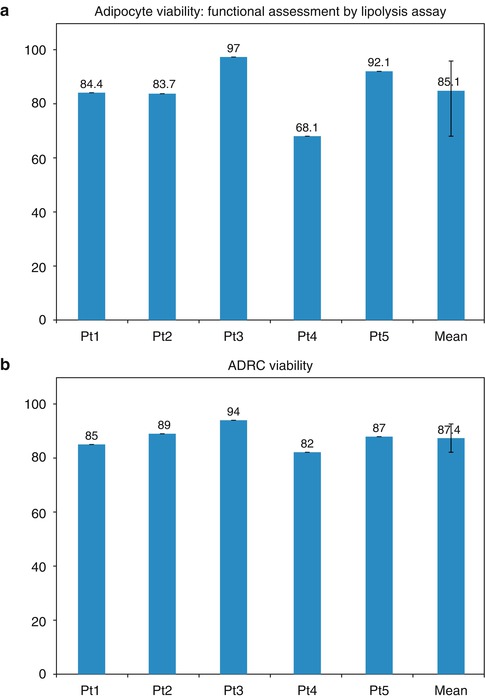
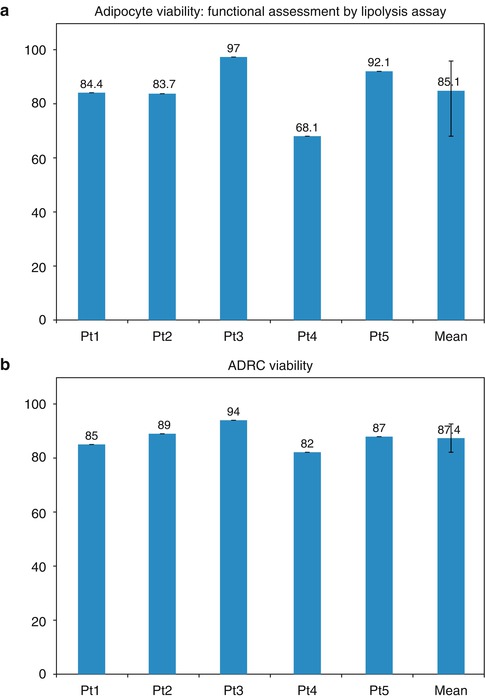
Fig. 35.2
(a, b) Adipocyte viability: functional assessment by lipolysis assay
In this application, the harvesting of the adipose tissue using VASER™ system minimizes tissue damage and improves the viability of the harvested cells.
35.2 Stem Cell Niche Therapy Using Adipose Tissue
Adipose-derived stem cell niche refers to the complex relationships that ADSCs establish with all of the physiological or ectopic factors contributing to determine their identity and fate. The phenotypical identity of any cell and its reaction to a given stimulus are, therefore, not only determined by the genetic or epigenetic equipment of the cell but also depend on the specific niche context in which it resides. A change in niche parameters or the transplantation of cells into other niches can have a considerable effect on cell physiology and alter its properties.
Research has shown that the signals controlling the embryonic origin of ADSCs and their differentiation in adult adipose tissue include the same pathways as those involved in the homeostasis of other adult and embryonic stem cell populations [1], and their complex orchestration can have different effects depending on the concentration; stage of differentiation and extrinsic niche factors, such as cell–matrix and cell–cell interactions; the presence of vasculature; and the level and type of innervation. Despite extensive research into the intracellular signaling involved in ADSC homeostasis and differentiation, little is known about the role of cell–cell and cell–matrix interactions. Zannettino et al. [2] have suggested that ADSCs reside in perivascular niches, which prompts the speculation that perivascular structures (cells and extracellular matrix) may provide signals that balance the maintenance of ADSCs in an undifferentiated state and their commitment to differentiation.
It has recently been shown that in vitro culture and expansion significantly alter the transcriptional phenotype of ADSCs. Freshly isolated stromal vascular fractions (SVFs) express hematopoietic markers (CD34) that are lost within a few days of culturing [3]. In line with previously published findings [4], the authors have found the increased expression of mesenchymal stem cell-associated markers in cultures of both human and murine ADSCs, whereas the longer-term loss of markers of undifferentiated status, such as undifferentiated transcription factor (UTF-1), indicated a shift toward differentiation. Prolonged culturing also significantly downregulated various isoforms of procollagen, matrix metallopeptidases, and inflammatory cytokines, thus indicating adaptation to the artificial environment. Under extreme conditions, it has even been shown that prolonged in vitro culturing can induce the neoplastic transformation of ADSCs [5], although it is still unclear whether these changes are reversible or how they may affect the therapeutic potential of the cell. However, ADSCs can be used in many clinical applications (particularly in the fields of plastic and reconstructive surgery) by means of transplantation without removing them from their fat niche.
In addition to molecular and cell biology, the dynamic and regenerative nature of fat grafts has been established in various areas of plastic surgery and clinical practice that has shown that the long-term outcomes of fat grafting can include rejuvenation of skin texture [6, 7], adipocyte formation, and a large number of precursors at different stages of differentiation. Ultrastructural studies of centrifuged fat have revealed that mature adipocytes show interruptions in their cytoplasmic membrane and various degrees of degeneration including cell necrosis, but the SVF appears to be well preserved. Rigotti et al. [8] compared the ultrastructure of mammary radiolesions before, and at different times after, fat grafting and found clear signs of regeneration, with evidence of new adipocyte phenotype. Before transplantation, almost all of the adipocytes were seriously damaged by centrifugation, and in the radio-damaged recipient tissue, there were neither mature adipocytes nor differentiating pre-adipocytes. Reasonable interpretations of these findings are that the differentiating pre-adipocytes observed after treatment were either adipocyte-committed ADSCs originally embedded within the transplanted fat or locally present endogenous adipocyte-committed ADSCs activated by the ectopically transplanted fat. The massive survival of ADSCs in fat transplants, despite liposuction and centrifugation procedures, strongly supports the first hypothesis, but the second may be equally valid as it assumes that transplanted fat enriched with ADSCs by centrifugation can behave as an atypical ectopic niche that orchestrates tissue regeneration by modulating endogenous tissue resources.
The term “atypical ectopic niche” could be used as a common paradigm of stem cell-based therapies. It is based on the idea of a “bystander” mechanism in which the stem cells ectopically transplanted into a generic lesion (radiolesion, myocardial infarction, cerebral stroke, etc.) do not replace tissue-specific cells by direct differentiation, but locally form an atypical stem cell niche that suppresses inflammation, promotes neoangiogenesis, and favors the activation of endogenous stem cell precursors by releasing trophic factors, such as cytokines, proangiogenic molecules, and growth factors. Therefore, adipose tissue can be considered an ideal “biologic product.”
The role of this essential component of the stromal fraction of the lipoaspirates argues for a regenerative theory based on angiogenesis imbued with various growth factors, not yet fully identified, released from stem cells. Thus, in addition to the traditional understanding that fat is a high-energy reservoir involved in homeostasis for its ability to bind large amounts of fluids and in the maintenance of thermoregulation, it becomes apparent that it is also a regenerative organ providing the basis for soft tissue regeneration.
ADSCs have become one of the most popular adult stem cell populations for research in soft tissue engineering and regenerative medicine applications. Compared with other stem cell sources, ADSCs offer several advantages including an abundant autologous source, minor invasive harvesting (liposuction), significant proliferative capacity in culture, and multi-lineage potential. To harvest the adipose tissue, a liposuction procedure is less invasive than bone-marrow aspiration, and the technique produces less patient discomfort and donor site morbidity. Small amounts of adipose tissue yield approximately 5 × 103 stem cells, which is 500-fold greater than the number of MSCs in 1 g of bone marrow [9]. Numerous preclinical studies have been pursued, with early clinical data appearing in the literature. Fat as a living, free graft does more than just fill the area into which it is placed. Grafted fat affects the tissue into which it is placed in many ways for the life of the patient. It can improve the quality of aged and scarred skin and heal radiation damage and chronic ulcers. Just how grafted fat causes these changes remains unanswered. We know that fat can perform amazing feats in a glass tube and in some animal models, but we have little insight into what happens to fat when it is grafted from one part of the human body to another part. The focus of the near future should be researched to explain and expand on future clinical successes.
In addition to the outstanding results of lipotransfer for volume restoration, surgeons have become increasingly interested in the apparent rejuvenative effects on the skin itself. Coleman has noted improved skin quality, with softening of wrinkles, decreased pore size, and improved pigmentation during the first year posttreatment. In fact, analysis of clinical results following both reconstructive and aesthetic surgery reveals that after one or several repeated fat grafting procedures, the trophicity and quality of the recipient site are improved. These changes develop over several months following the fat grafting procedure, with an often variable expression, as improvements in skin texture, skin suppleness, skin color, and/or scar quality.
Topographical skin analysis systems such as the recently developed VISIA system (Canfield Imaging Systems) may determine whether the effect from fat grafting is an effective skin rejuvenation technique in comparison to chemical peels and laser resurfacing. Aside from effects on normal, aged skin, when fat has been grafted beneath depressed scars, there was improvement not only in the depression but also in the character of the scar itself, with an apparent transformation to normal-appearing skin. Other authors have reported a diverse range of improvements in soft tissue conditions, including radiation damage, breast capsular contracture, damaged vocal cords, and chronic ulceration, as well as regrowth of calvarial bone. While many of the exact mechanisms for these effects remain to be described, what seems to be at the center of these changes is the presence of adipose-derived stem cells (ADSCs) in human adipose tissue. In fact it has been proven to be the most abundant source of stem cells in the body.
35.3 Benefits of Stem Cells in a Fat Transfer
1.
Grow new blood vessels to nourish the fat.
2.
Release anti-inflammatory agents to aid healing.
3.
Generate and release growth factors that support graft survival.
4.
Improve skin tightening and rejuvenation.
5.
No risk of allergic or adverse reaction.
6.
A cutting-edge option used around the world today.
7.
The therapeutic target is the reconstruction of autologous niches, by simply moving stem cell niches from one connective tissue in which they are abundant into another in which they are scarce [9].
35.4 Patient Selection
All the patients selected for bilateral breast augmentation to improve shape, contour, and volume using VASER™ as primary source of fat harvest followed these criteria:
1.
No significant PMH or strong family history of breast cancer.
2.
Mammography.
Pre-op as baseline to exclude pathology. Patients with fibroadenoma, suspected cysts >1 cm, or significant calcifications are discouraged.
3.
Patients with moderate to severe glandular or skin ptosis are denied surgery (Fig. 35.3).
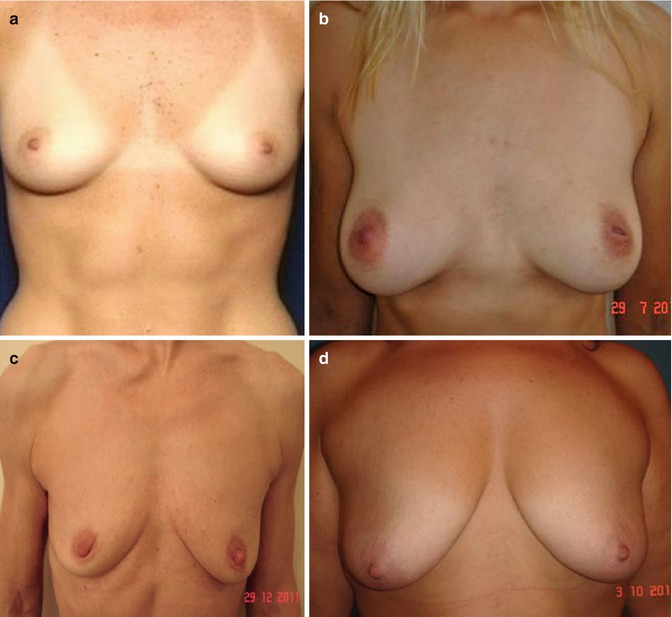
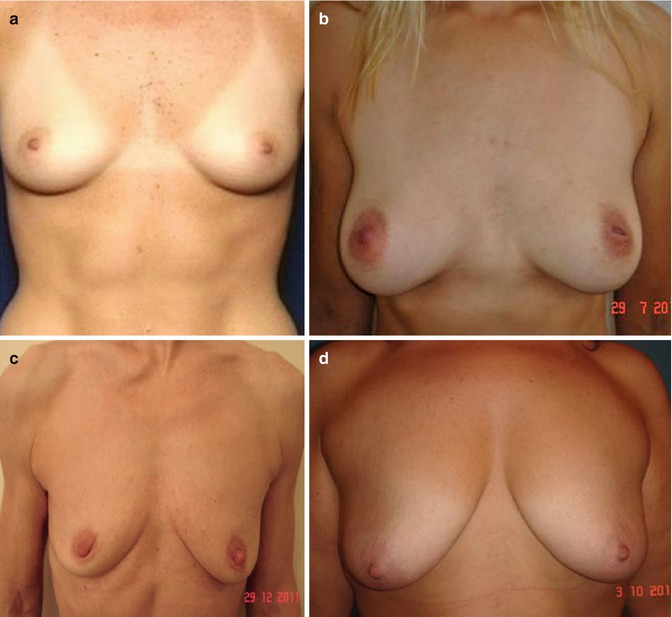
Fig. 35.3
(a) Good candidate for fat transfer (good skin tone, elasticity, nipple position). (b) Good candidate for fat transfer (mild ptosis). (c) Poor candidate (breast ptosis, poor skin tone, no projection). (d) Contraindication to fat transfer for breast enlargement (severe breast ptosis)
4.
Fat donor area (Fig. 35.4).
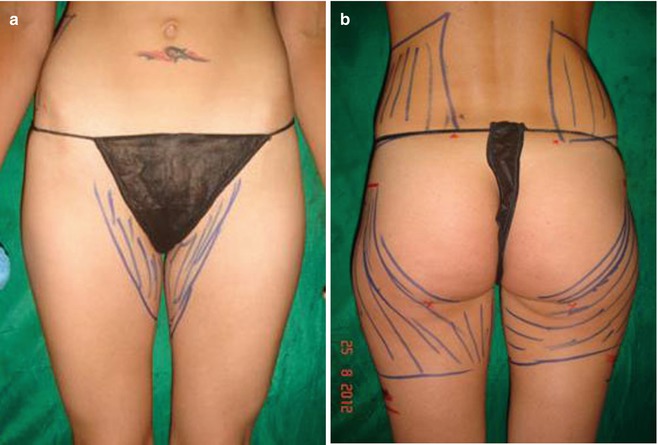
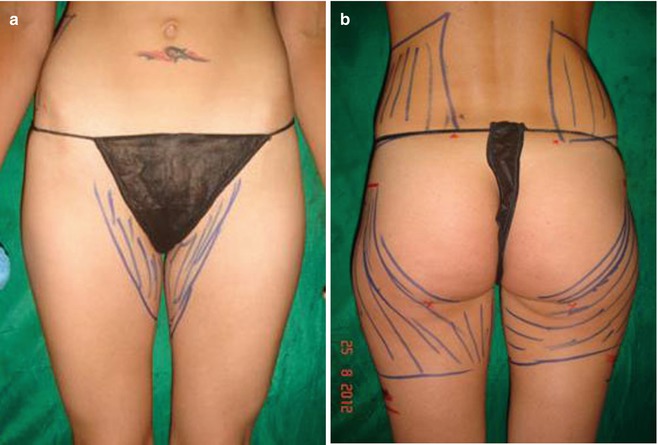
Fig. 35.4
(a, b) Preoperative donor area for fat transfer: trochanters, inner thighs, and flanks. From this patient a total of 1,800 mL emulsion was removed and 600 mL of PureGraft was transferred
5.
Extremely slender patients are denied surgery (Fig. 35.5).
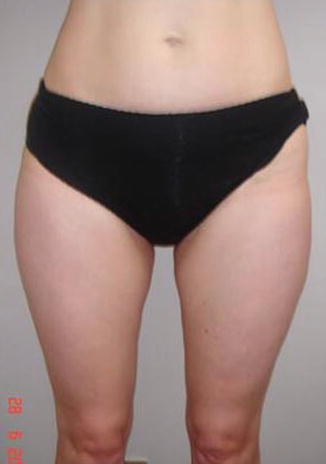
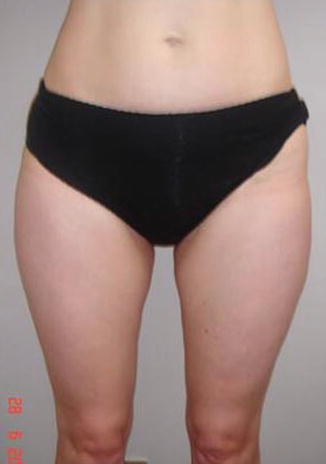
Fig. 35.5
Extremely slender patient. Contraindication for fat transfer
35.5 Patient Assessment
All candidates underwent:
1.
Preoperative clinical evaluation.
2.
Photographs.
3.
4.
Some patients had 3D imaging pre- and postoperatively to assess fat survival and fat distribution in long-term follow-up.
5.
In preparation for surgery, standard ECG and blood test.
6.
All patients operated were ASA 1–2 categories.
7.
Patients were warned to maintain their weight in the post-op period.
8.
Weight loss could severely influence fat retention.
Hormonal changes, as in pregnancy and menopause, may affect fat retention, decreasing the breast volume.
9.
Patients with unrealistic expectations of large volume were denied surgery.
35.6 Selection of Site of Fat Harvesting (Fig. 35.8)
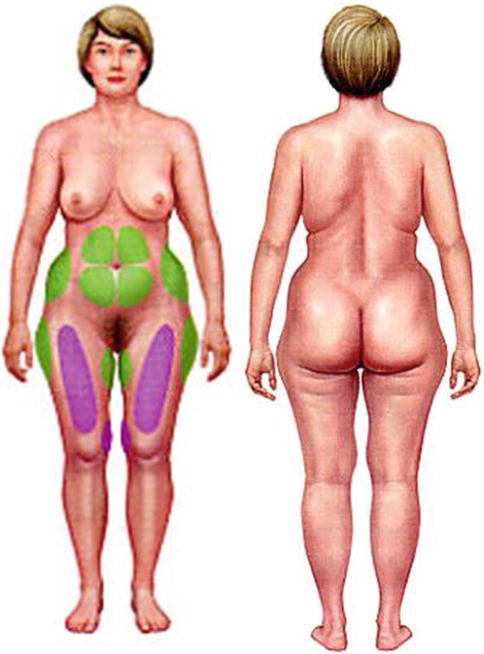
Fig. 35.8
Sites for fat harvesting
1.
Abdomen
2.
Flanks
3.
Anterior thighs
4.
Outer thighs
5.
Arms
6.
Buttocks
35.7 Selection of Anesthesia
All the patients in this series underwent office-based surgeries, with local tumescent anesthesia and intravenous (IV) sedation. Tumescent anesthesia consisted of 1,000 mL normal saline (0.9 %), 1 mL adrenaline (1:1,000), and 500 mg lidocaine. Author DW uses routinely 600–800 mg of lidocaine in his tumescent anesthesia. Breast tumescence infiltration ranges from 100 to 300 mL (Fig. 35.9).
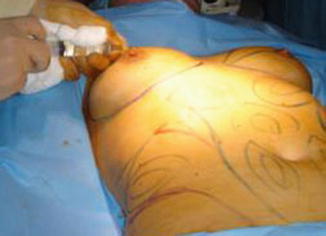
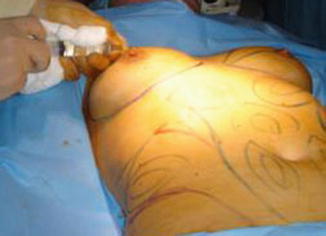
Fig. 35.9
Local anesthetic in peri-areolar incision
IV sedation is given by an anesthetist to a fully monitored patient, consisting mainly of propofol, fentanyl, midazolam, and IV paracetamol. Sedation was mainly light in the majority of patients remaining conscious and conversant. Fentanyl and/or Fentanest was added following patient’s analgesia requirement and according to anesthetist protocol and indications.
Harvest site infiltration ranges from 2,000 to 4,000 mL; aspirate ranges from 800 to 3,000 mL.
PureGraft ranges from 280 to 400 mL.
All patients had intraoperative antibiotics. Author ADG uses metronidazole IV (400 mg) and cephotaxime (1 g) followed by 5 days of oral course of the same antibiotics while author DW uses co-amoxiclav 1.2 g IV 5 days oral postoperatively. Antiemetic drugs and gastroprotectors were given by author ADG routinely.
Typical dressing includes compression garment (apart from breast, where a light support bra (impact level III) or brassiere is advised). Absorbable pads were used for postoperative leakage. Soft silicone drains (corrugated) were positioned at the lower abdomen. Analgesics were used (if needed). All patients were discharged 1–2 h on average after surgery.
Follow-up was on the first and second days postoperatively. Manual lymphatic drainage (MLD) starts on the third day post-op, followed by LPG (endermologie or similar) or VelaSmooth program post-liposuction, for 6/8 weeks on areas treated. Breasts need no compression for the first 3/4 weeks (i.e., sleeping in supine position). Swelling normally lasts about 3 weeks. Two-pillow elevation of the thorax for the first 2–3 weeks is advised to reduce swelling as well as a light bra for few weeks.
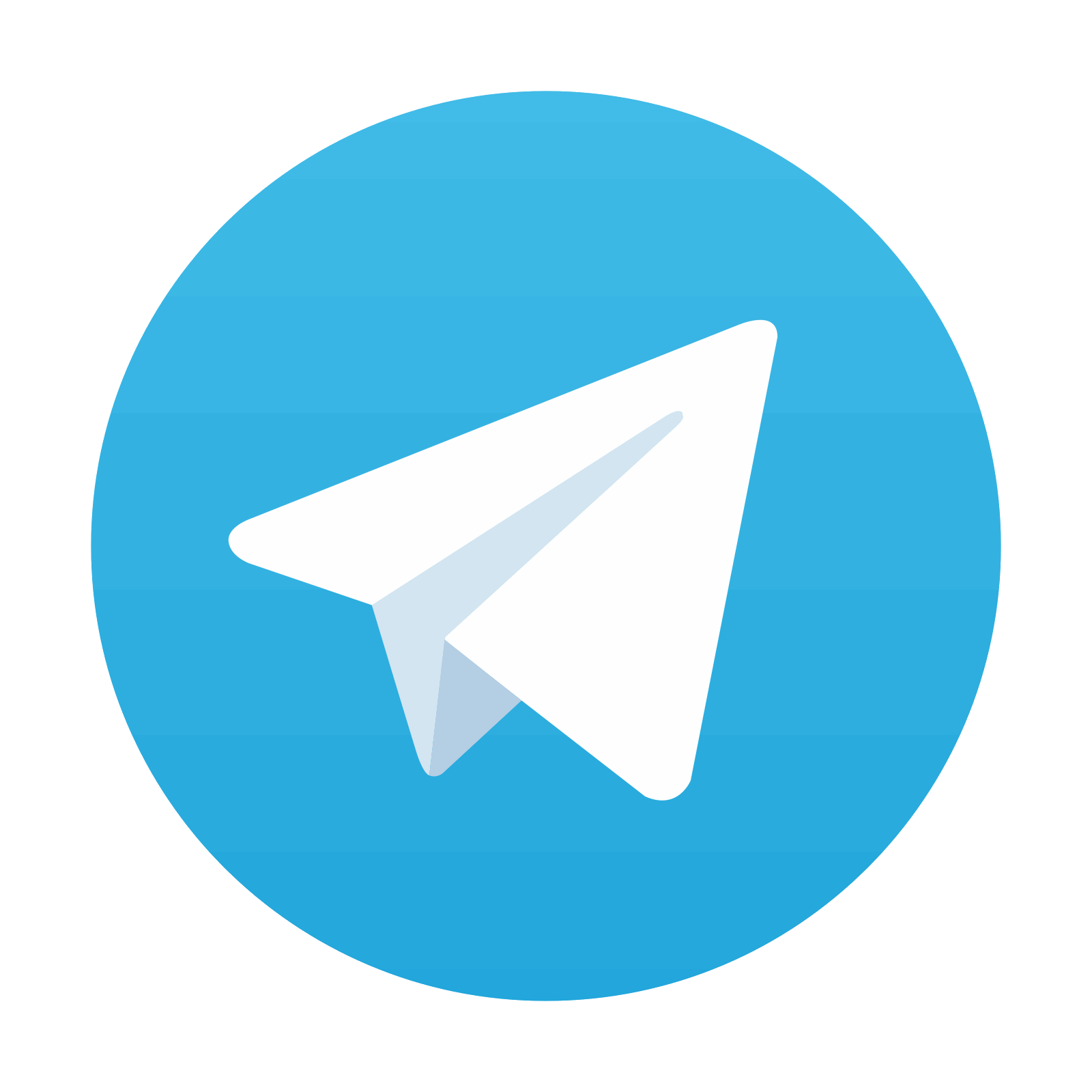
Stay updated, free articles. Join our Telegram channel

Full access? Get Clinical Tree
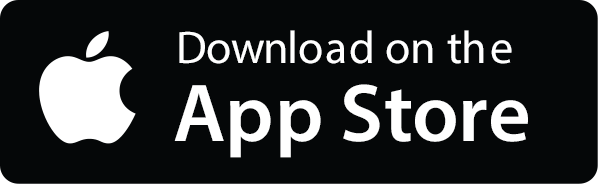
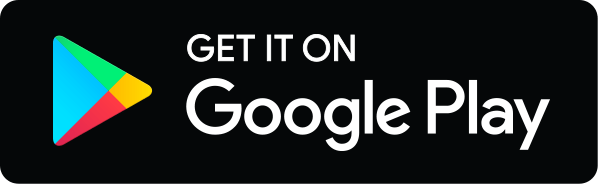