Chapter 28 Biomechanics of Intratunnel Anterior Cruciate Ligament Graft Fixation
Introduction
In 1983, Lambert1 first introduced the technique of intratunnel anterior cruciate ligament (ACL) graft fixation by securing a vascularized bone–patellar tendon–bone (BPTB) ACL graft with 6.5-mm AO cancellous screws. General acceptance of interference screw fixation of BPTB ACL grafts came about in large part due to the biomechanical study of Kurosaka et al.2 This study demonstrated that fixation of a 10-mm BPTB ACL graft in human cadaveric knees with a custom-designed, headless, 9-mm, fully threaded interference screw had superior strength and stiffness compared with fixation with a 6.5-mm AO cancellous screw, staple fixation, or sutures tied over a button.2 Because of the many biomechanical studies demonstrating superior initial fixation properties and clinical outcomes studies demonstrating a high rate of success, interference screw fixation of BPTB grafts is now considered the standard against which all ACL graft fixation techniques are compared.3,4 Based on the success of interference screw fixation of BPTB ACL grafts, Pinczewski5 in 1993 introduced the use of blunt threaded metal interference screws to fix four-strand hamstring tendon ACL grafts. This fixation technique has subsequently been extended to the use of nonmetallic bioabsorbable interference screws.
In the late 1960s, Morrison,6,7 using force plate and gait analysis, estimated the forces experienced by the ACL during activities of daily living to range from 27 N to 445 N. Noyes et al8 estimated that the ACL is loaded to approximately 454 N during activities of daily living. However, at the present time the forces placed on the ACL with rehabilitation exercises performed in the early postoperative period or during activities of daily living are unknown. In vitro mechanical studies have demonstrated that the initial strength and stiffness of BPTB and four-strand hamstring grafts far exceed the estimated loads on the ACL.8–10 However, compared with ACL grafts, all current ACL graft fixation methods demonstrate inferior initial tensile properties.11 Therefore mechanical fixation of the ACL graft in the bone tunnels is the weak link in the early postoperative period. Consequently, initial graft fixation properties are of great relevance in determining the success of ACL reconstruction in the early postoperative period.
Limitations of Biomechanical Studies
In vitro biomechanical studies are most commonly used to evaluate initial ACL graft fixation properties.12,13 However, in vitro biomechanical studies have inherent limitations. First, the use of different research models and biomechanical testing protocols make it difficult to compare the results of one study with another. Ideally, human specimens are used for biomechanical testing; however, the material properties of cortical and cancellous bone, tendons, and ligaments can vary greatly among specimens. Because of the lack of availability of human cadaveric specimens in the age range of patients typically undergoing ACL reconstruction, specimens from older donors are often used or the same specimen is tested multiple times. As demonstrated by Brown et al,14 the use of specimens from older human donors underestimates the fixation strength of fixation devices that rely on cancellous bone for fixation strength. In this study, the initial fixation strength of BPTB grafts fixed with metal interference screws in the distal femur of bovine cadavers, young human cadavers (mean age 41 years, range 33–52 years), and elderly human cadavers (mean age 73, range 68–81 years) was compared. There was no significant difference in the failure load of the bovine (799 ± 261 N) and young human specimens (655 ± 186 N); however, the failure load of the elderly human specimens (382 ± 118 N) was significantly lower than the young human and bovine specimens. Based on these findings the authors concluded that elderly human cadavers are not an appropriate model for ACL reconstruction fixation studies. Performing multiple tests in the same specimen will introduce carryover effects that may affect the fixation properties of subsequent fixation techniques after the first fixation method has been tested. Beynnon and Amis12 suggest testing male specimens younger than 65 years old and female specimens younger than 50 years old to minimize these problems.
Due to the paucity of suitable human cadaveric specimens, animal models are often used. Animal models have the advantage of eliminating the potential variability introduced because of the large differences in bone mineral density (BMD) that exist in human specimens, and their availability eliminates the need to perform multiple tests using the same specimen. However, because of the differences in BMD and tensile properties of bone that exist between human and animal specimens, the results of biomechanical tests performed using animal models cannot be directly compared with studies performed using human specimens.15 Aerssens et al15 have shown that human female femoral specimens (age range 30–60 years) demonstrate lower BMD and failure stress compared with specimens from dogs, pigs, cows, or sheep. In this study the pig femur came closest to matching the BMD and failure stress of the human femur. Because of the higher BMD and tensile properties of animal specimens, biomechanical tests performed in animal models tend to overestimate initial fixation properties.16,17 This is particularly true for devices such as interference screws and cross-pins that rely on cancellous bone for fixation strength.
Another limitation of in vitro biomechanical studies is that they simulate the time zero period prior to biological fixation of the ACL graft. These studies fail to account for the progressive healing of the ACL graft to the bone tunnel walls, which shifts the weak link from the ACL graft–fixation–bone tunnel interface to the bone–ligament interface and eventually to the intraarticular part of the ACL graft.18 Although the healing response does not affect graft fixation properties in the early postoperative period, bony or soft tissue healing in the bone tunnels will alter graft fixation properties over time. Few studies document the time frame for healing to occur at the ACL graft fixation sites. Based on the studies of Clancy et al18 and Walton,19 it appears that the bone blocks of BTB grafts heal to the bone tunnel wall by 6 weeks. Compared with BTB grafts, soft tissue grafts take longer to heal to bone. In a dog model, Rodeo et al20 demonstrated the formation of Sharpey’s fibers connecting the periphery of a soft tissue graft to the bone tunnel wall at 6 weeks. However, mechanical fixation was not achieved until 12 weeks.
Two types of biomechanical tests are commonly used to evaluate the mechanical behavior of ACL ligament fixation techniques.12,13 The first and most commonly used is the single-cycle load to failure (single LTF) test. Single LTF tests attempt to simulate the response of the graft fixation technique to a sudden mechanical overload event such as a slip or fall. The load-displacement curve can be analyzed to determine the ultimate failure load, yield load, linear stiffness, and displacement at failure. Advantages of single LTF testing are that the weak link in the fixation system can be easily identified, the mode and site of the fixation failure are well defined, and an upper limit of the strength of the graft-fixation construct is established. Because failure testing attempts to replicate traumatic loading conditions, a high rate of elongation, typically 100% per second, is used.
The second testing method involves cyclical loading of the bone–ACL graft–fixation complex. Cyclical testing evaluates the ability of the bone–ACL graft–fixation complex to resist elongation or slippage under repetitive submaximal failure loads over time. Cyclical testing attempts to approximate the loading conditions associated with rehabilitation exercises or activities of daily living in the early postoperative period prior to biological fixation of the graft. Most commonly a load control test is performed, in which the upper and lower loads are controlled and displacement over time of the ACL graft relative to the bone is measured. By determining the distance between markers on the bone and the ACL graft at the beginning and end of the test, elongation or slippage of the ACL graft with respect to the bone can be measured. At the present time there is little agreement on the force limits or the number of cycles that should be performed, making it difficult to compare data among studies. Beynnon and Amis12 have recommended force limits between 150 N and −150 N and 1000 load cycles. One thousand cycles approximates 1 week of flexion-extension loading of the knee.13 The number of cycles is limited by the ability to keep the specimen moist during testing and the thawing of the freeze clamps that are commonly used to grip soft tissue ACL grafts.
Bone Mineral Density
Because intratunnel fixation methods depend on the graft fixation device generating friction between the bone tunnel wall and the ACL replacement graft, BMD is perhaps the most important variable that influences initial fixation strength and stiffness and resistance to slippage during cyclical loading. It is well known that BMD in humans decreases with age and that the BMD of females is less than that of males. Cassim et al21 found that the fixation strength of BPTB grafts fixed with metal interference screws in human specimens with a mean age of 79 years resulted in a 42% decrease in failure load compared with specimens with a mean age of 35 years. There are also significant differences in the BMD of the human distal femur and proximal tibia.22 The BMD of the proximal tibia is significantly lower than that of the distal femur.22 The lower BMD of the proximal tibia, along with the fact that tibial fixation devices must resist shear forces applied parallel to the axis of the tibial bone tunnel, combine to make tibial fixation the weak link in ACL graft fixation.
Although BMD is a critical factor, other variables correlate with initial fixation properties. In a BPTB model, Brown et al14 found that insertion torque, an indirect measure of BMD, was linearly correlated with pullout force but with weak significance. Using elderly human cadaveric knees, Brand et al22 found that BMD measured using dual-energy x-ray absorptiometry and screw insertion torque was strongly correlated to the fixation strength of doubled semitendinosus and gracilis tendons fixed with bioabsorbable interference screws in the distal femur and proximal tibia of human specimens. In this study, the variables of insertion torque and BMD explained 77% of the ultimate failure load observed. The R2 value for the relationship between ultimate failure load and BMD was 0.65, indicating that BMD explained 65% of the ultimate failure load. This study found that BMD of 0.6 gm/cm2 resulted in better initial fixation properties. Using the proximal tibia of human cadaveric specimens (mean age 40 ± 11 years, range 17–54 years) and doubled tibialis tendons fixed with a tapered bioabsorbable screw, Jarvinen et al23 found that insertion torque was linearly correlated to fixation strength (R2 = 0.54) and was the most strongly associated variable in their study for predictors of fixation strength. Unfortunately, despite the correlation, insertion torque was a poor predictor of cyclical loading failure or single LTF. Clearly, other secondary factors influence the properties of intratunnel graft fixation. The remainder of this chapter is aimed at understanding the role and importance of these other variables.
Bone–Patellar Tendon–Bone Fixation
Interference screw fixation of BTB ACL grafts has been well studied and documented over the past 20 years. The fixation properties of interference screw fixation of BTB grafts depend on the generation of friction between the bone block and bone tunnel wall. Friction is generated by compression of the bone block into the bone tunnel wall and engagement of the screw threads into the bone block and bone tunnel wall. As illustrated in Fig. 28-1, factors that influence the initial tensile properties of interference screw fixation of BTB ACL grafts include the following:
Overlap exists between the effects of screw diameter and gap size on initial fixation properties. In the study of Kurosaka et al,2 custom-made 9.0-mm screws demonstrated higher ultimate failure loads compared with 6.5-mm AO cancellous screws. However, the researchers reported that making the size of the patellar and tibial bone blocks close to the size of the bone tunnels was extremely important “in obtaining a solid fixation.”2 Using a porcine experimental model, Reznik et al24 demonstrated that gap size significantly influenced the ultimate failure load of BTB grafts fixed in 10-mm bone tunnels with 7-mm screws. When the gap between the bone block and bone tunnel wall was 4 mm or more, increasing the screw diameter to 9 mm increased the failure load by 97%. However, when the gap was less than 4 mm and a 9-mm screw was used, the results were inferior to those seen with a 7-mm screw and a gap of less than 4 mm. Kohn and Rose,25 using human cadaveric knees (mean age 30 years), reported that both femoral and tibial fixation using 9-mm screws were stronger than with 7-mm screws. Based on their findings, they recommended against using 7-mm screws for tibial fixation.
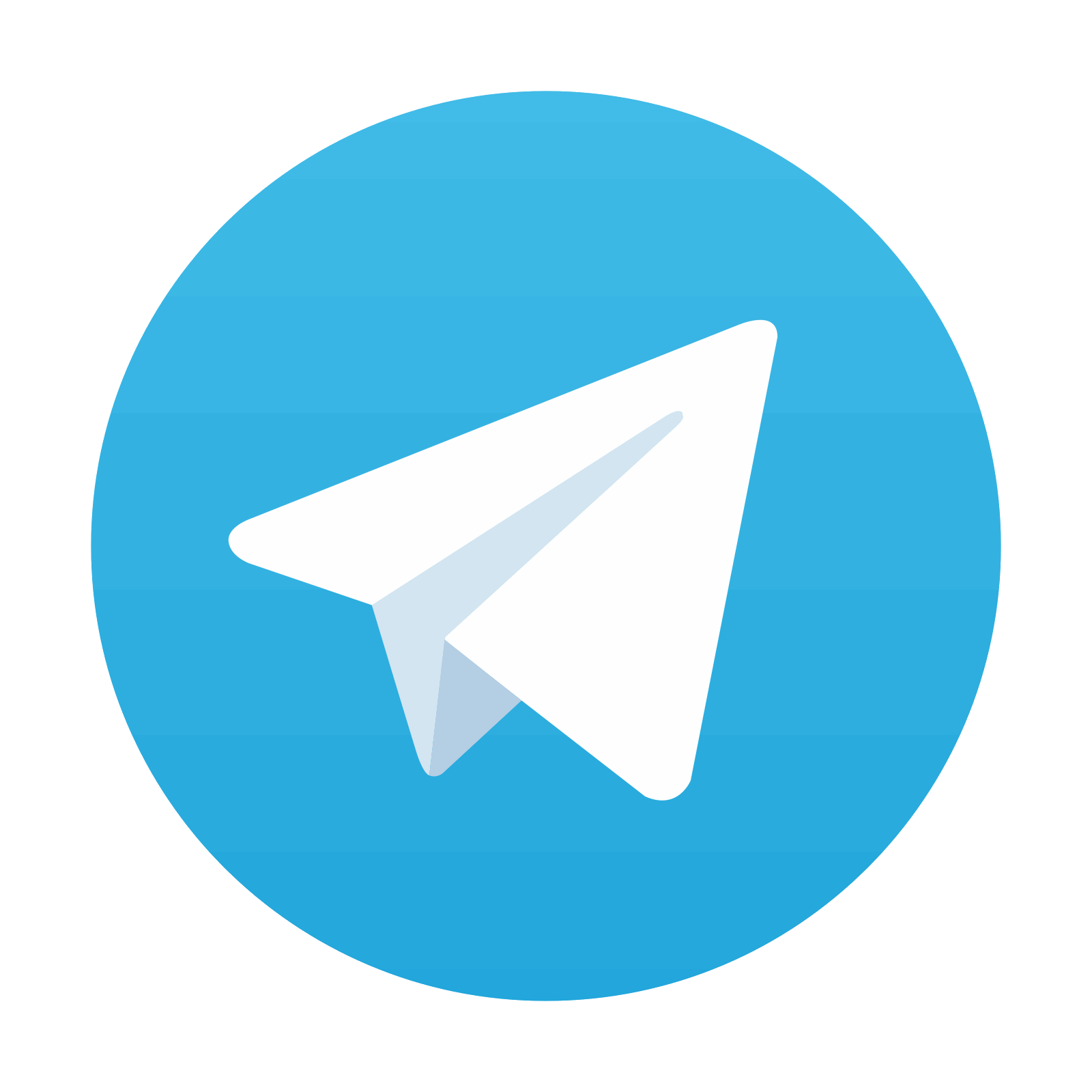
Stay updated, free articles. Join our Telegram channel

Full access? Get Clinical Tree
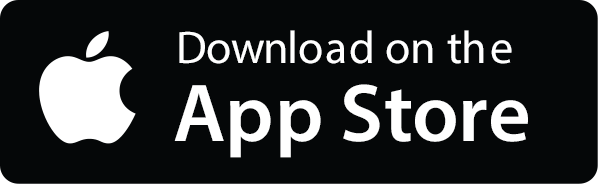
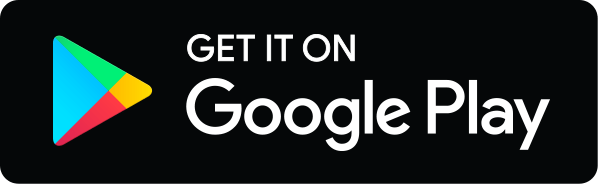