Skin Embryology The earliest stage of skin development is known as the specification stage, which occurs by the 4th week of gestation. During specification, ectoderm lateral to the neural plate is committed to become epidermis, and subsets of mesenchymal and neural crest cells are committed to form the dermis. Initially, a single layer of ectodermal cells covers the embryo’s surface. In the 2nd month of gestation, this layer divides to form a superficial protective layer of simple, flattened squamous epithelial cells, the periderm. The cells of the periderm layer then undergo keratinization and begin to slough superficially to be replaced by cells arising from the basal layer. The framework of future layers and specialized structures of skin are now formed. Next, during the morphogenesis stage, these tissues begin to take their particular form: The dermis and hypodermis are delineated; vascular formation occurs; epidermal stratification and epidermal appendage formation also take place. By the 11th week, the basal layer (stratum germinativum) forms an intermediate skin layer. By the end of the 4th month, all the epithelial layers of the epidermis have acquired their definitive arrangement. Also by the 4th month, the mesoderm underlying the ectoderm has differentiated into the dermal layer, which begins to produce collagen and elastic fibers; blood vessel formation also takes place in this dermal layer. Hair follicle and sebaceous gland growth initiate in the growing fetus by the 5th month. During the first 3 months of development, neural crest cells infiltrate the epidermis and differentiate into pigment-producing melanocytes.1 The skin is divided into three main layers that vary in quality and appearance in different areas of the body: the epidermis, dermis, and superficial fascia. The epidermis is divided into five distinct layers and may range in thickness from 0.05 mm on the eyelids to 0.8 to 1.5 mm on the soles on the feet. The cellular layers of the epidermis from deepest to most superficial (▶ Fig. 2.1) include: Fig. 2.1 Depiction of the microscopic appearance of the dermis and epidermal layers. Note the progression of the nucleated keratinocytes of the basal layer as they migrate superficially and differentiate into the anucleated, flattened corneocytes of the stratum corneum. Stratum basale (basal or germinal cell layer) Stratum spinosum (spinous or prickle cell layer) Stratum granulosum (granular cell layer) Stratum corneum (horny layer) Stratum lucidum (a thin layer of translucent cells seen in thick epidermis representing a transition from the stratum granulosum and stratum corneum) The epidermis is mainly composed of keratinocytes, with smaller numbers of melanocytes, Langerhans cells, and Merkel cells. The Langerhans cells are dendritic cells responsible for capturing and presenting antigens within the epidermis to circulating lymphocytes. Merkel cells are somatic afferent sensory cells that provide light touch discrimination; they can become neoplastic in some cases and form Merkel cell carcinoma. The stratum basale, the innermost layer, lies adjacent to the dermis and contains keratinocytes in various stages of division, attached to the basement membrane by hemidesmosomes. The basal layer also contains melanocytes, the pigment-producing cells. Through their dendritic processes, the melanocytes transfer pigmented melanin granules in vesicles named melanosomes to keratinocytes. The melanosomes accumulate atop the nucleus of the recipient cell protecting the deoxyribonucleic acid (DNA) from ultraviolet (UV) radiation damage. Melanin, a derivative of tyrosine, is an effective absorber of light; it is able to dissipate more than 99.9% of absorbed UV radiation. The number of melanocytes is the same in equivalent body sites in human beings of all skin pigments; however, the distribution and rate of production of melanin differ. The cells of the stratum basale are anchored into a complex structure known as the basement membrane, or dermoepidermal junction. This poorly defined, eosinophilic staining structure provides nutrients and waste disposal for the epidermis, and acts as chemical and physical barriers. The basement membrane has strong anchoring collagen fibrils that secure it to the underlying dermis. In addition, the undersurface of the epidermis contains epithelial projections called rete ridges, or rete pegs, that extend between the papillae of the dermis and provide increased mechanical support. Superficial to the stratum basale lies the stratum spinosum, or prickle cell layer that derives its name from the appearance of the intercellular desmosomes linking these keratinized polygonal cells. The next layer is the stratum granulosum, or granular cell layer. At this point the keratinocytes begin to lose their nuclei, exhibit coarse cytoplasmic granules, and appear basophilic. On the palms of the hand and the soles of the feet there is an intermediate, clear cell layer, the stratum lucidum; this represents a transition between the cells of the stratum granulosum and stratum corneum. The stratum corneum, the outermost layer, forms a protective barrier against infection, dehydration, chemicals, UV radiation, and mechanical stressors. Studies have determined this layer to be from 8.7 to 12.9 µm thick.2 The cells of this layer, known as corneocytes, are anucleated keratinocytes that become flattened and stack up 10- to 30-cells thick at the skin surface. The palms of the hand and soles of the feet have the highest density of corneocytes at the skin surface. The corneocyte is enveloped in a proteinaceous covering and contains water-retaining keratin. In addition, the extracellular matrix surrounding these cells contains stacked, lipid bilayers providing a hydrophobic barrier. The dermis is found beneath the basement membrane and is composed of a supportive cell matrix. The dermis varies in thickness from 0.6 mm on the eyelids to 3 mm on the back, palms of the hands, and soles of the feet. Within the dermis lie cells such as fibroblasts, mast cells, and macrophages, as well as branches of the peripheral nervous system, blood vessels, and epidermal appendages. The two layers of the dermis are the papillary layer and the reticular layer. The papillary dermis contains loosely organized collagen fibers, blood vessels, and fibroblasts. It is located below the dermoepidermal junction and is relatively thin in comparison with the reticular dermis. The fibroblasts are plentiful within the papillary dermis and are responsible for synthesizing collagen, elastin, and proteoglycans. During the wound-healing process they may become active collagen-producing cells and may transform into contractile cells during the contraction phase of wound healing. Experimental data suggest that this differentiation occurs after wound formation under the influence of transforming growth factor-beta (TGF-β1), mechanical tension, and exposed extracellular matrix components. Blockade of the contraction cascade and treatment of wounds with new dermal regeneration templates appear to prevent scar formation.3 The reticular dermis is composed of rigid, netlike, bundles of collagen that run parallel to the skin surface, extending from the papillary layer to the subdermis or superficial fascia. The structure of the dermis differs greatly at diverse anatomical sites. For example, the structure of facial dermis is highly influenced by abundant hair follicles and numerous large sebaceous glands in men. The high concentration of these appendages likely puts the connective tissue matrix of the dermis under added tension. Hair follicles are most abundant on the scalp and the face, and are derivatives of the epidermis and dermis. Each hair follicle is lined by germinative cells that produce keratin and melanocytes that synthesize pigment. The hair shaft consists of an outer cuticle, a cortex of keratinocytes, an inner medulla, and a surrounding root sheath. An erector pili muscle is associated with the hair shaft and is innervated by the sympathetic nervous system. Sebaceous glands are epidermal cell derivatives that are found in the hair-bearing regions of the skin. Sebaceous glands secrete a substance called sebum through holocrine secretion into the hair follicle. Sebum may help to maintain the epidermal permeability barrier, function in hormonal signaling, transport antioxidants, and provide added protection from UV radiation. Sweat glands are classified into two main types: apocrine and eccrine. Apocrine glands are found in the axillae and groin and produce an odorless, protein-rich secretion. When acted upon by bacteria, this secretion becomes odiferous. Eccrine sweat glands are found throughout the body, and in especially high density on the palms, soles, forehead, and axillae. In fact, the only cutaneous regions lacking eccrine glands are the mucocutaneous junctions and nail beds (▶ Fig. 2.2). Fig. 2.2 All the layers of the skin including dermal appendages, blood vessels, and peripheral nervous structures are shown here. This layer, also known as the subcutis, contains a mixture of fibrous and fatty tissue. The ratio of fibrous to fatty substance differs based on the following factors: bodily region, gender, and nutritional status (smaller fat deposits in more malnourished individuals). On the trunk and extremities the fatty substance predominates, allowing easier surgical undermining of the skin.4 On the face the subcutaneous tissue plays quite an important functional role since it contains the tendon fibers of the facial muscles.5 The superficial fascia of the face contains a mixture of fibrous septa and fatty tissue. On the lateral face, the fibrous septa attach to the dermis with vertical septa, forming the superficial musculoaponeurotic system (SMAS) that extends from the platysma to the galea aponeurotica. The SMAS constitutes a compartment through which vessels branch and connect before reaching the subdermal level. The deep plexus is a vascular network that lies in the outer portion of the superficial fascia. From this deep network small arteries pass vertically to the papillary dermis becoming the superficial plexus. Branches of the superficial plexus give rise to ascending capillary loops that supply the dermal papillae before becoming venules in the superficial venous plexus. Due to the dilated arteriolar network concentrated in the face, telangiectasias of the facial skin respond well to lasers emitting wavelengths in the yellow portion of the spectrum.4 Venous predominant telangiectasias respond better to sclerotic agents than to laser therapy. All cutaneous nerves have their cell bodies in the dorsal root ganglia. Free nerve endings in the dermis sense pain and temperature. Specialized receptors named Meissner corpuscles lie in the dermal papillae and receive light touch sensation. Pressure and vibration are detected by specialized structures named Pacinian corpuscles that are densely distributed throughout the superficial fascia of the palms and soles. The autonomic nervous system supplies the motor innervation of the skin―adrenergic fibers innervate blood vessels, erector pili muscles, and apocrine glands―whereas cholinergic fibers innervate eccrine sweat glands. The endocrine system regulates the sebaceous glands, which are not innervated by autonomic fibers. The wound-healing process consists of four highly integrated and overlapping phases6: Hemostasis Inflammation Proliferation Tissue remodeling Tissue injury (e.g., abrasion, laceration, burn, or contusion) affecting the dermal blood vessels triggers an immediate 5- to 10-minute vasoconstrictive response. The exposed, injured endothelial cells activate the coagulation cascade initially triggering platelet aggregation and adhesion. Vasoconstriction is then followed by a period of vasodilation, due to mast cell-mediated release of histamine and serotonin. Vasodilation allows infiltration of platelets and leukocytes, which release cytokines, thereby initiating the inflammatory process―persisting for up to ~ 72 hours. Gross inflammation manifests as hyperemia and edema in and around the wound. Cytokine release by platelets, exposure to bacterial antigens, and the complementing activation cascade stimulate neutrophil influx into the wound. The inflammatory stage can be classified into two parts: In the early phase there is an influx of neutrophils followed by a late phase in which macrophages predominate. Cytokine release and the complementing activation stimulate neutrophil migration, an essential aspect of proper wound healing. The neutrophils migrate to the site of the wound and through phagocytosis remove bacteria and foreign debris from the wound. By the 3rd day after the injury, macrophages become the predominant cell type found in the healing wound. Macrophages stimulate wound healing through direct phagocytosis; activation of lymphocytes; and synthesis of potent growth factors such as transforming growth factor (TGF), fibroblast growth factor (FGF), platelet-derived growth factor (PDGF), and vascular endothelial growth factor (VEGF). These growth factors promote endothelial cell growth, fibroblast proliferation, and angiogenesis. By the 3rd to 7th day of wound healing, the system focuses on covering the wound surface, forming granulation tissue, and restoring the vascular network. This is referred to as the proliferative stage. During this period fibroblasts migrate along the fibrin network laying down the matrix of connective tissue for wound closure and restoration of mechanical strength. Next, keratinocytes lying at the edge of the wound, as well as epithelial stem cells from hair follicles and sweat glands, migrate toward the center of the wound. This process occurs through enzymatic loosening of keratinocyte desmosomes and keratinocyte “shuffling” along the newly created fibrin network along a chemotactic network toward the center of the wound.7 The final wound-healing stage, known as neovascularization, deals with the restoration of the intricate vascular network of the fresh wound. Existing endothelial cells on intact neighboring vessels interact with newly synthesized growth factors from the wound and begin to proliferate and sprout new branches that connect with others, forming vascular loops. From this point, the new endothelial cells may recruit pericytes and smooth muscle cells in order to differentiate into venules and arterioles. Next, granulation tissue forms through high density of fibroblasts, granulocytes, macrophages, capillaries, and loose bundles of collagen. It appears characteristically red and fragile due to disorganized, incomplete angiogenesis. The final stage of wound healing is tissue remodeling, which may last from 21 days up to 1 year after injury. The fibroblast is the workhorse of extracellular matrix formation and is responsible for wound strengthening by the conversion of type III collagen to the stronger type I collagen. Fibroblasts begin to differentiate into myofibroblasts, leading to wound contraction and closure. Wound myofibroblasts help decrease wound surface area through extracellular collagen fiber deposition and contraction by intracellular contraction and concomitant alignment of the collagen fibers by integrin-mediated pull on collagen bundles. Finally, wound blood flow declines and metabolic activity within the wound stops. Multiple experimental models have demonstrated that skin damage caused in utero heals differently from nonfetal skin. Fetal skin injuries heal without scar formation and with preservation of dermal appendages such as hair follicles and sebaceous glands.3 In nonfetal scar tissue the dermoepidermal junction is repaired without rete ridges and dermal skin appendages, which may contribute to the susceptibility of scar tissue to damage from shearing forces. Scar formation in skin wounds appears to be secondary to contraction during healing; this was demonstrated in studies that showed near abolishment of scar formation when skin wounds were manipulated with scaffolds that partially blocked contraction.3 In the newborn, the skin is smooth, usually uniform in pigmentation, and lacks surface irregularities. During the aging process there are endogenous and exogenous causes of skin change. Endogenous or intrinsic aging proceeds in all organisms due to the interplay of predetermined genetic factors and the influence of time. Endogenous changes can be characterized by cellular or microscopic tissue changes or macroscopic and functional changes. Similar to cellular aging in other organs, endogenous aging is thought to involve decreased proliferative capacity leading to cellular senescence, and altered biosynthetic activity of skin-derived cells.8 It is believed that the progression of keratinocytes from their beginnings in the stratum basale to the anucleated corneocyte in the stratum corneum takes ~ 2 weeks. This cellular maturation process of the epidermal keratinocytes is thought to progress more slowly as the human being ages, thereby diminishing the capacity for re-epithelialization after tissue insult. As a result, corneocytes are found to be larger due to delayed epidermal turnover. Loose adhesion of the corneocytes due to the delayed epidermal turnover rate may contribute to epidermal skin loss after repeat application and removal of adhesive tape in the older adult, as well as predispose to higher rates of contact dermatitis due to greater dermal permeability. The aging skin is also characterized by a decrease in the number of basal keratinocytes per unit area, thinning/flattening of the dermoepidermal junction, a decrease in Langerhans cell population, and a decrease in active melanocyte population by 8 to 20% per decade.9 This population decline affects epidermal and hair follicle melanocytes resulting in irregular skin pigmentation and hypopigmented hair. Thinning of the dermoepidermal junction is observed by the sixth decade and is attributed to a decrease in the number and size of dermal papillae. Studies have demonstrated a decrease from 40 papillae/mm2 in young skin to 14 papillae/mm2 in subjects age 65 and older. In older skin, this decrease in dermal papillae interdigitation leads to increased susceptibility to tissue damage from shearing forces.10 Other changes include decreased cell populations of Meissner and Pacinian corpuscles, resulting in poorer discrimination of pressure and light touch. Over time, changes to the dermis include: elastic fiber degradation, decrease in collagen synthesis, decrease in blood vessel and nerve ending distribution, and overall decrease in cellularity. Rete pegs flatten in the aging human, causing increased susceptibility to shearing forces from minor trauma in the older adult. In the human dermis the organization of collagen fibers determines the mechanical characteristics of the tissues and resistance to deformational forces. Elastin functions to recoil the stretched collagen fibers to their relaxed position. Fibroblasts and dermal dendrocytes produce proteoglycans, glycosaminoglycans, and glycoproteins that permeate the elastin network creating a structural steady state of connective tissue fibers.11 As humans age there is a decrease in the elastic rebound properties of the skin with an increase in extensibility. This is likely due to increased degradation of dermal collagen by tissue matrix metalloproteinases over time. Collagen becomes more haphazardly oriented and loss occurs at around 1% per year throughout adult life.12,13 There does not appear to be any relationship between gender and quantifiable change in human skin biomechanics over time. One of the most ubiquitous and obvious skin changes with aging is reduction in skin thickness. Thinning of skin affects all cell layers, although dermal collagen and elastin losses account for most of the reduction in total skin thickness. This effect is most obvious in exposed areas such as the extensors, neck, upper chest, and face. The hypodermis and superficial fascia tend to undergo atrophy of fat deposits, contributing to volume loss; the basement membrane, however, is noted to increase in thickness over time (▶ Fig. 2.3). Fig. 2.3 Skin aging is characterized by endogenous and exogenous changes. Sun exposure mainly affects the epidermal layer of the skin, whereas age-related changes are found in each level. Epidermal pigment change in the aging skin, thinning of the epidermis and dermis, and atrophy of adipose tissue in the subcutaneous layer are displayed here.
2.2.1 Epidermis
2.2.2 Dermis
2.3 Skin Appendages
2.3.1 Superficial Fascia
2.3.2 Blood Vessels
2.3.3 Nerves
2.4 Wound Healing
2.5 Skin Aging
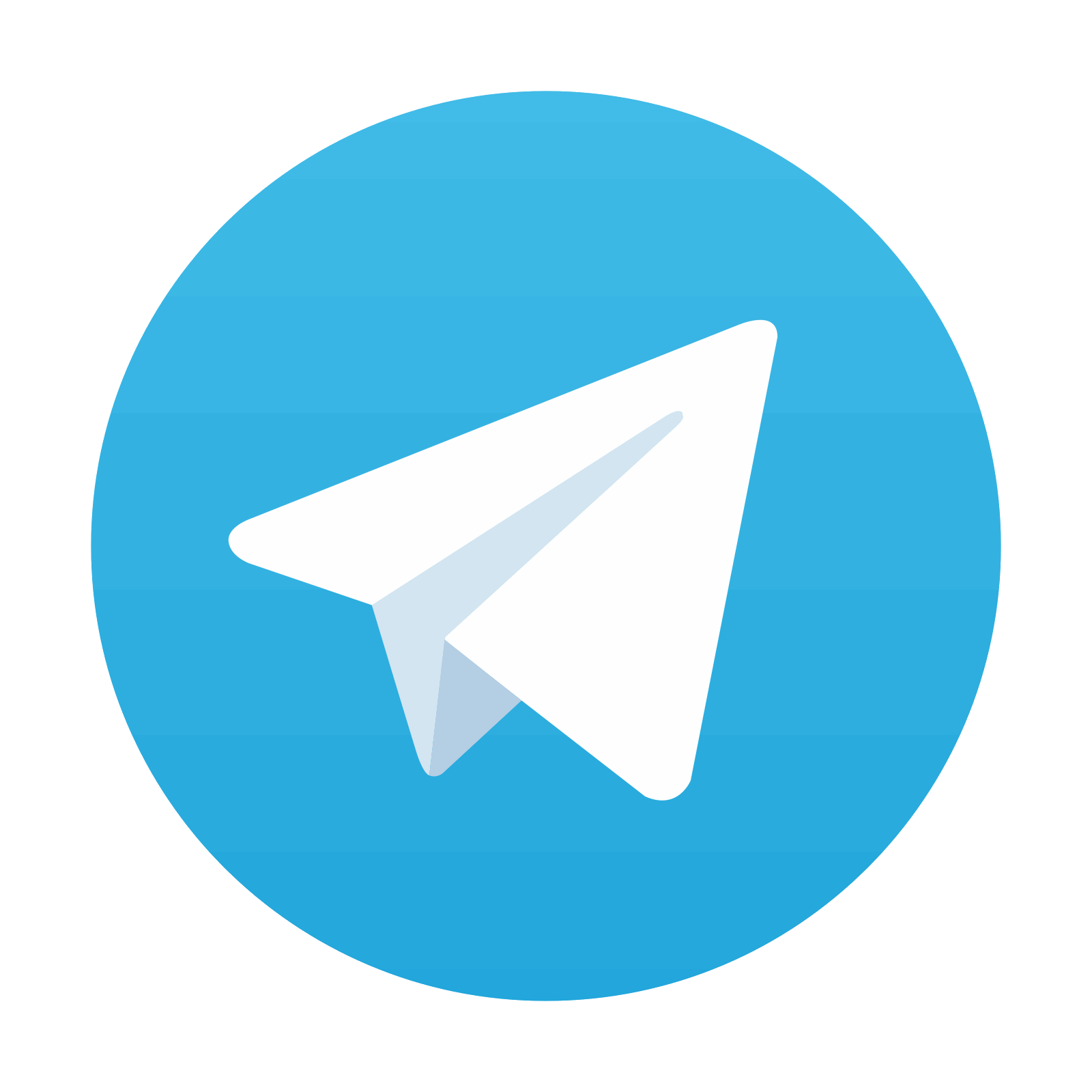