Differentiation in
Main reference
Adipogenic cells
[12]
Chondrogenic cells
[12]
Endothelial cells
[13]
Hematopoietic cells
[14]
Hepatocytes
[15]
Laryngeal epithelial cells
[16]
Osteogenic cells
[12]
Oligodendrocytes
[17]
Neurons
[18]
Pancreatic endocrine cells
[19]
Renal tubular epithelial cells
[20]
Retinal pigment epithelial cells
[21]
Schwann cells
[22]
Skeletal muscle cells
[23]
Skin epithelial cells
[24]
Smooth muscle cells
[25]
Urothelium-like cells
[26]
ASCs, referred also as stromal vascular fraction cells (SVFs), are collected from adipose tissue by collagenase digestion and differential centrifugation; this procedure can be performed in approximately 1–2 h [11]. Since liposuction involves only local anesthesia, cells may be obtained from the patient with a repeatable, not debilitating operation and autologously transplanted at the site of tissue regeneration. The possibility of autologous transplant represents a clear advantage compared to the use of cells from allogeneic or xenogeneic sources, reducing the risks associated with immune compatibility and tolerance.
Abundance of the adipose tissue which can be harvested means that clinically relevant numbers of cells could be obtained. This eliminates the need for large in vitro expansion of cells before transplant, a process associated with increased risk of genetic instability and with problems correlated to the maintenance of the precursor cells in an undifferentiated state. Isolation of ASCs from adipose tissue can be performed in a large-scale, reproducible manner according to Good Manufacturing Practice (GMP) regulations. The translation of these procedures has lead to phase I/II clinical trials involving transplantation of autologous adipose-derived cells for different conditions [6, 29] including healing of Crohn’s fistulas [30], multiple sclerosis [31], urinary incontinence [32], and rheumatoid arthritis [33]. In addition, the use of adipose-derived cells to resurface vasculogenic and osteogenic prosthetic grafts is under evaluation [34].
Currently, there are several preclinical and clinical studies investigating a possible use of ASC-based cell therapy interventions for tissue regeneration and repair of chronic and acute tissue damage [6]. The major areas of research include promotion of angiogenesis, wound healing, and treatment of skin aging.
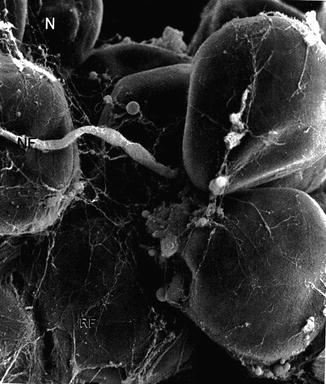
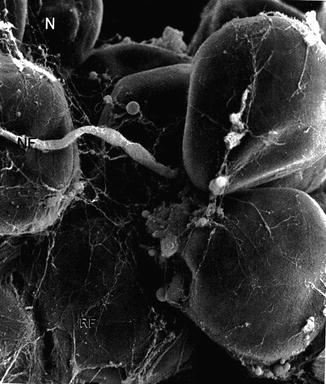
Fig. 21.1
Adipose tissue at electron microscope. N nucleus, NF nerve fiber, RF reticular fibers (Motta P: Anatomia Microscopica, 3a edizione, Piccin, Padua, Italy 1984)
21.3 Adipose Tissue Homeostasis
In the human body the process of tissue regeneration and repair is continuously active in response to cell turnover in physiological homeostasis (balance between proliferation and apoptosis, i.e., programmed cell death) or as consequence of aging, traumas, diseases, or congenital anomalies. In tissues with high cellular regenerative potential, such as hematopoietic tissues and skin and mucosal gastrointestinal epithelium, the process of repair results in restitutio ad integrum (i.e., reconstruction of the damaged tissue with an analogous one). In quiescent tissues, characterized by the presence of cells with low replicative potential such as neurons and cardiomyocytes, the process of repair is mainly compensatory, with deposition of fibrotic tissue in replacement of the injured area, with consequent scar formation. Typically scar tissue is due to connective tissue and excessive extracellular matrix (ECM) deposition. ECM normally provides the necessary framework for cell migration and maintains the correct polarity in multilayered cellular structures. Furthermore, ECM embeds fibroblasts, macrophages, and other cell types essential for reparative processes, including resident stem cells, which can actively play a role in tissue regeneration. During tissue repair peripheral blood circulating and tissue resident stem cells are activated and recruited at the site of injury. Homeostasis maintenance, activation, and homing of stem cells are regulated by soluble mediators (ligands), by cell-to-cell interactions, and by the influence of the microenvironment surrounding the cells. The signals can be mediated by ligands of different nature, including cytokines (Table 21.2), growth factors (Table 21.3), ECM peptides, and hormones, which reach specific surface receptors on the target cell and activate the signal transduction cascade.
Table 21.2
Main cytokines involved in wound healing
Cytokines | Main sources | Biological activity | Excessive levels | |
---|---|---|---|---|
Pro inflammatory | TNFα tumor necrosis factor | Macrophage | Pleiotropic hemostasis, increased vascular permeability, increased vascular proliferation | Impaired wound healing |
IL-1 interleukin-1 | Macrophage | Neutrophil activation, adhesion molecule upregulation, fibroblast and keratinocyte proliferation collagen synthesis, endothelial cell induction to secrete proinflammatory cytokines | Chronic nonhealing wound | |
IL-2 interleukin-2 | T lymphocytes | T-cell activation, increase of fibroblast metabolism, promotion of inflammatory cell and fibroblast infiltration | ||
IL-6 interleukin-6 | Macrophage | Stem cell and fibroblast proliferation, B and T lymphocyte activation | Chronic diseases and severe burns | |
IL-8 interleukin-8 | Macrophage and fibroblasts | Increased neutrophil and monocyte chemotaxis, keratinocyte migration | Psoriasis | |
IFN-γ interferon γ | T lymphocytes and macrophage | Macrophage and neutrophil activation and increased cytotoxicity, wound remodeling | ||
Anti-inflammatory | IL-4 interleukin-4 | T lymphocytes | B-lymphocyte proliferation, IgE release, inhibition of macrophage production of proinflammatory cytokines, fibroblast proliferation collagen and proteoglycan synthesis | Scleroderma |
IL-10 interleukin-10 | T lymphocytes, macrophages, and keratinocytes | Inhibition of neutrophil activation; synthesis of proinflammatory cytokines | Chronic venous insufficiency ulcers |
Table 21.3
Main growth factor involved in wound healing
Growth factor | Cell source | Biological activity |
---|---|---|
EGF epidermal growth factor | Keratinocytes, macrophages | Migration and proliferation of keratinocytes for the reepithelialization and the formation of granulation tissue; fibroblast proliferation and collagenase secretion |
HGF hepatocyte growth factor | Platelets, mesenchymal cells, fibroblasts, endothelial cells | Mitogen epithelial cells, endothelial cells, hepatocytes, biliary epithelium cells, epithelial cells of the lungs, mammary glands, and other tissues |
VEGF vascular endothelial growth factor | Keratinocytes, mesenchymal cells, macrophages, fibroblasts | Vasculogenesis/angiogenesis; improve granulation tissue formation; increase vascular permeability |
Isomers C and D stimulate lymphangiogenesis | ||
PDGF platelet-derived growth factor | Platelets, macrophages, endothelial cells, keratinocytes, muscular smooth cells | Migration and proliferation of fibroblasts, smooth muscle cells, and monocytes; chemotactic for macrophages, PMNs; active PMN, macrophages, and fibroblasts; mitogen for endothelial cells; collagen and proteoglycan synthesis; production of MMPs, fibronectin; angiogenesis and wound contraction; improved wound breaking strength and healing time |
TGF-β transforming growth factor β | Platelets, macrophages, endothelial cells, fibroblasts, keratinocytes, T cells, muscular smooth cells | Multiple actions, sometimes even tweak opposite. Inhibitor of growth for most of the epithelial cells and leukocytes; proliferation of fibroblasts and smooth muscle cells; powerful fibrogenic; chemotaxis of PMNs, macrophages, smooth muscle cells, fibroblasts; ECM deposition with collagen, fibronectin, and proteoglycan synthesis; reduces collagen degradation; increases TIMP; involved in the development of fibrosis in many chronic inflammatory states |
TGF-β3 conversely decreases collagen and fibronectin deposition, prevents scarring | ||
FGF fibroblast growth factor acidic (aFGF or FGF-1); basic (bFGF or FGF-2, 10 times more potent) | Macrophages, mastocytes, endothelial cells, T cells, fibroblasts | Angiogenesis by endothelial cell migration and proliferation |
Wound healing by migration of macrophages, endothelial cells, fibroblasts; keratinocyte migration and proliferation; ECM synthesis and wound contraction | ||
FGF-7 and FGF-10, respectively, KGF-1 and KGF-2 | Fibroblasts, endothelial cells | Important mediator of epidermal-dermal interaction, keratinocyte migration, proliferation and maturation, for an improved reepithelialization; in particular KGF-1 may be important in epidermal homeostasis |
IGF insulin-like growth factor | Macrophages, neutrophils, fibroblasts, liver, skeletal muscle | Helps regulate cell metabolism and growth; keratinocyte migration; fibroblast and keratinocyte proliferation; collagen, proteoglycan, protein, and glycogen synthesis (tissue metabolism); endocrine effects similar to those of growth hormone; angiogenesis |
Signals can be (Fig. 21.2):
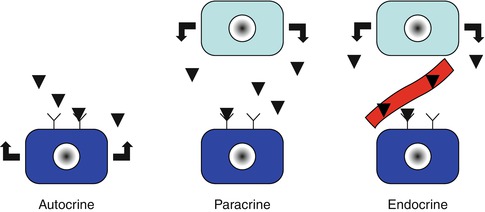
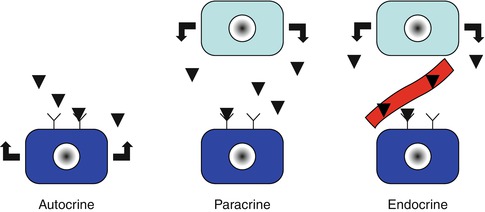
Fig. 21.2
Different types of cell signaling
1.
Autocrine: ligands are produced by the same target cell.
2.
Paracrine: ligands are produced by different adjacent cells.
3.
Endocrine: ligands correspond to hormones produced by an endocrinal cell situated at distance and released in circulation.
21.4 The Microenvironment Where the Stem Cells Are the Niche
Resident skin stem cells have been identified in various niches including the epidermis (hair follicle bulge, sebaceous glands, and interfollicular epidermis) and the dermis (dermal papilla of hair follicle). The dynamic microenvironment of stem cells includes adjacent cells, ECM architecture, signaling molecules, physical forces, oxygen tension, and other environmental factors. ASCs are intimately associated with the perivascular space. This localization, in addition to specific receptor PDGFRβ expression, supports the current hypothesis that ASCs derive from the perivascular niche. ASCs play a role in skin and vascular homeostasis, controlling hair follicle stem cell activity through PDGF signaling. ASCs exhibit significant potential for skin repair via cytokine release, promotion of fibroblasts’ migration during wound healing, and neovascularization.
21.5 Adipose Tissue and Adipose Tissue-Derived Stromal Cell Transplant for the Treatment of Epithelial Pathologies
21.5.1 Angiogenesis Promotion
The development of capillaries occurs during embryogenesis via the proliferation, specification, and differentiation of hemangioblast-derived cells into endothelial cells (ECs). In healthy adult individuals, the low basal turnover of the endothelium (angiogenesis) is thought to occur by proliferation and migration of preexisting mature ECs. The term “postnatal vasculogenesis” indicates the generation of vascular endothelial cells from precursors in addition to mature endothelial cells as defined in angiogenesis.
Attempts to increase blood flow to ischemic tissue and to promote tissue regeneration have included a variety of physical and biological methods. These approaches include gene transfer of proangiogenic molecules or transplantation of cells capable to support vessel sprouting by a paracrine effect or by directly participating into new vessel formation. A cell transplant approach for therapeutic angiogenesis was originally demonstrated using bone marrow-derived circulating cells referred as endothelial progenitor cells (EPCs) [35]. These cells were isolated from human peripheral blood by selection for the hematopoietic marker CD34. These cells were found to differentiate into endothelial cells and to express both hematopoietic markers such as CD133 and endothelial cell markers, including CD31 and VEGF receptor type 2. Crucially, these cells are also incorporated into sites of angiogenesis in vivo. These observations were the first to suggest that blood vessels in adults may not only arise by angiogenesis but may also result from recruitment to blood vessels of a progenitor cell population. This opened the way to cell-based therapeutic interventions to promote angiogenesis for tissue repair and regeneration.
The definition of EPCs derived from bone marrow has been elusive, as various labs have used different approaches to their isolation and characterization. Therefore, despite their use in several clinical trials, the functional characteristics and even the existence of circulating EPCs are still object of debate [36, 37]. New evidence indicates that in case of injury, EPCs may be recruited from other tissues including adipose tissue [38]. In fact, mature endothelial cells (ECs) [24] and EC precursors [39, 40] able to incorporate into vessels and promote postischemic neovascularization can be easily isolated from adipose tissue.
We recently described the use of a microcannula, normally utilized for Klein’s solution infiltration and designed with small ports, to harvest fat from the more vascularized hypodermic layer. With this procedure the authors have been able to improve of approximately twofold the recovery of ASCs compared to conventional suction-assisted liposuction, which removes fat from a deeper layer (Fig. 21.3).
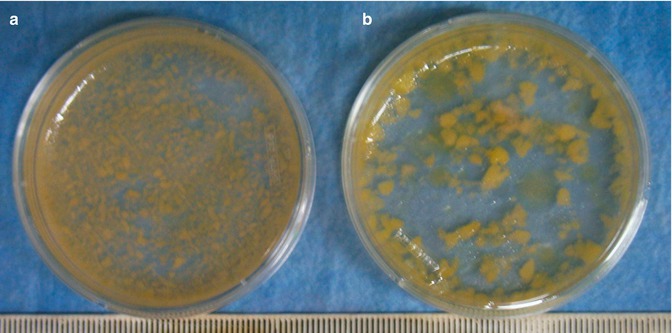
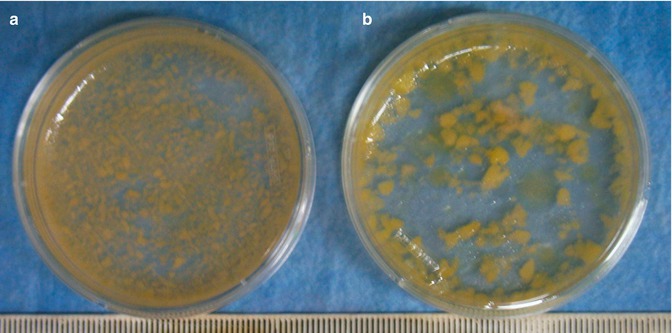
Fig. 21.3
Lipoaspirate gross examination. (a) Macroscopic examination of adipose tissue fragments obtained with standard cannula (blunt tip, 170 mm long, 3 mm Ø, single port Ø 3 × 9 mm). (b) Infiltration with microcannula (blunt tip, 170 mm long, 2 mm Ø, 5 ports, single port Ø 1 mm) [41]
Moreover, the authors assessed an increase in the percentage of cells able to acquire endothelial-like morphology, to express the CD31 endothelial specific marker, and to form vascular-like networks in vivo and in vitro and which can persist for several weeks upon transplant in animal models, possibly taking part in vascular regeneration (Fig. 21.4) [41]. Recently evidence showed that the ASCs isolated from the superficial layer of the abdominal fat tissue presents different features than those harvested from the deep layer. They proliferate significantly faster. They are more resistant to apoptosis. They have most affinity for skin stem cells, with which interact for homeostasis, or wound healing, therefore they are more effective for skin regeneration.
To harvest these cells, we have patented a 2 mm microcannula with 4 holes of 1 mm diameter arranged on a single row (Fig. 21.4e).
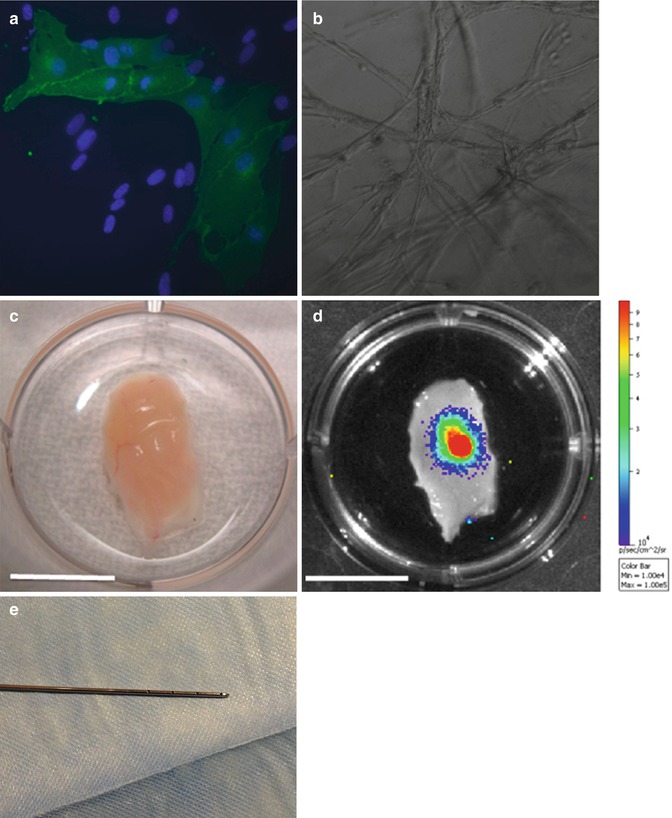
Fig. 21.4
Adipose tissue-derived cells for vascular promotion. (a) ASC expression of endothelial specific marker CD31 assessed by immunohistochemical analysis (magnification 40×). (b) Formation of capillary-like network in a Matrigel assay in vitro. Implant of Matrigel plug containing 7 × 105 luciferase expressing human adipose-derived cells in immunosuppressed mice (magnification 40×). (c) Vessels were visible after Dissection 3 weeks after implantation in the region surrounding the gel plug (scale bars 1 cm). (d) The same area was analyzed by bioluminescent analysis, assessing engraftment of human cell plug (scale bars 1 cm). (e) 2 mm microcannula with 4 holes of 1mm for harvesting of the superficial fat layer [41]
21.5.2 Wound Healing
Cutaneous wound healing is a complex, multistep process involving cells, ECM, and soluble factors. The main phases of this process are coagulation, inflammation, proliferation, retraction, and remodeling.
The coagulation phase begins immediately after injury with platelet aggregation and release of a great variety of products (i.e., vasoactive substances, adhesive proteins, growth factors, cytokines, proteases, and proenzymes of the intrinsic and extrinsic coagulation cascade, including prostaglandin D2, E2, F2, thromboxane A2, acid-12-idrossi-eicosatetraenoic, LTB4, serotonin, thrombospondin, fibronectin, fibrinogen, platelet factor 4, PDGF, EGF, TGF-β, TGF-α, cytokines, proteases – collagenase, Q antitrypsin, cathepsin) inducing the formation of a fibrin clot. There is also the release and the processing of some soluble factors by endothelial cells, attracted to PDGF, FGF, and ECGF (endothelial cell growth factor). The clot is critical for reepithelialization occurring at the wound edges; moreover, the clot provides a provisional matrix for the migration of inflammatory cells.
The inflammatory phase is characterized by leukocyte migration at the wound site. Neutrophils arrive first and secrete IL-2 and IL-5 with the main action of phagocytosis and lysis of bacteria. Monocytes attracted to PDGF, FGF, TGF-β, TNFα, IFNγ (secreted by T cell and NK), microbial stimulus (e.g., LPS), and bacterial endotoxins arrive next (3rd–10th day), differentiating into macrophages, with proinflammatory functions through antigen presentation, phagocytosis, and production of growth factors and cytokines, including PDGF, TNFα, IL-1, IL-6, IL-8, TGF-β, and TGF-α, for recruitment and proliferation of fibroblasts and keratinocytes and other angiogenic factors released under hypoxic conditions. Lymphocytes arrive later (6th–7th day): they secrete migration inhibitory factor (MIF), IL-2, and IFN-gamma. They have a role in macrophage activation and fibroblast proliferation. Fibroblasts secrete IL-2, IL-6, and IL-8. But the same actions could also cause damage to the surrounding healthy host tissues, if not interrupt it, with passage to the next step.
The proliferation phase is characterized by reepithelialization, neovascularization, and extracellular matrix (ECM) remodeling. During this phase macrophages stimulate proliferation of connective, endothelial, and epithelial cells. At this stage, the rate of moisture in the wound bed is critical for keratinocyte migration. The VEGF pathway mediates the formation of the granulation tissue (3rd–4th day), the aggregation of fibroblasts, inflammatory cells, newly formed vessels, and ECM components. VEGF is also responsible for the increase in vascular permeability, which leads to the formation of an exudate, containing plasma proteins, such as fibrinogen and fibronectin, forming a provisional matrix for the growth of fibroblasts and endothelium. The new vessels are labile and allow for the passage of red blood cells and proteins (fibrinogen and fibronectin) into the extravascular space; for this reason the granulation tissue is edematous. Fibroblasts, stimulated by TNF, IL-1, PDGF, FGF, TGF, and EGF secreted by platelets, macrophages, and activated endothelial cells, produce type III collagen, glycosaminoglycans, elastin, fibronectin, and some proteases, such as collagenase, which serve for the removal of debris. Glycosaminoglycans, including hyaluronic acid, present in the granulation tissue are fundamental for their hygrophilous capacity and for their chemotactic and proliferative action on cells during repair. Accumulation of collagen depends both on increased synthesis and on reduced degradation. In the late stages of granulation tissue formation, there is a gradual replacement of hyaluronic acid with proteoglycans, which controls collagen synthesis, but especially stabilizes links between cells and ECM, hindering cellular migration. During angiogenesis endothelial cells migrate into the wound under the chemotactic effect of VEGF, angiotensin, and FGF. Some authors have described also the recruitment of endothelial precursor cells (EPCs) derived from the bone marrow through pathways mediated by TNFα, FGF, TGF-α, prostaglandins, and angiogenin. Some of these factors, such as TNFα, are probably released by macrophages in hypoxic condition and have strong chemoattractive action on endothelial cells, promoting their migration into the wound.
During the contraction phase, collagen fibers pull together the wound hedges. Dysfunction during this process may cause functional and/or cosmetic deformities.
The remodeling phase (the equilibrium between synthesis and degradation of ECM) is characterized by disappearance of leukocyte infiltrate, edema, diminished vascularity, complete coverage of mature epithelium, replacement of hyaluronic acid by proteoglycan, and gradual replacement of fibronectin and collagen type III, by collagen type I. During remodeling there is no increase in collagen deposition, but the wound breaking strength is highly increased because macrophages and fibroblast work to replace the randomly dispersed collagen fibers with cross-linked fibers organized along the skin lines of tension, conferring resistance to the healing tissue. During this phase the matrix metalloproteases (MMPs) play a pivotal role. They are produced not only by the basal keratinocytes of the skin adjacent to the wound but also by macrophages, fibroblasts, and neutrophils under the stimulus of PDGF, FGF, IL-1, TNF, EGF, phagocytosis, and physical strength. The MMPs are proteases, such as collagenase, gelatinase (active on amorphous collagen and fibronectin), and stromelysin (active on amorphous collagen, laminin, proteoglycans), that digest matrix proteins. MMPs are necessary for removing debris in the damaged areas and for tissue remodeling. Their activity is strictly regulated since their uncontrolled action may cause damages to healthy tissues. MMPs are then inhibited by TGF and steroids and inactivated by tissue inhibitors of metalloproteinases (TIMP), produced by mesenchymal cells.
21.5.3 Chronic Wound
The skin is at the interface with the external world and maintains key homeostatic function throughout life. Sometimes causes such as persistent infections, autoimmunity, and prolonged exposure to exogenous and/or physiological insults disturb normal skin homeostasis. Complication in normal skin repair, especially in patients suffering from inadequate healing (diabetic, obese, or aged), may result in chronic wounds, excessive scarring, or even malignant transformation. The chronic wound is a persistent nonhealing wound characterized by unbalanced proinflammatory cytokine production and increased (even 100 times higher) protease activity (mainly matrix metalloproteinases).
Stem cell-based therapies offer tremendous potential for skin regeneration following injury or disease and in chronic wound. The beneficial effects observed after delivery of stem cells at the wound site seem to be correlated to their paracrine activity toward activation of host cells that are capable of modulating the inflammatory response, angiogenesis, and ECM remodeling. ASCs are also able to regenerate injured or diseased tissue via direct transdifferentiation influenced by local microenvironment and by a variety of secreted factors. In addition, ASCs contribute to reduction of inflammation, promoting regulatory T cells and inhibiting macrophage activation [44].
Therefore, topical administration of progenitor cells is emerging as a valuable option for the treatment of diabetic chronic ulceration for which current treatments are not sufficient to guarantee adequate healing [45]. The authors recently reported that administration of ASCs promotes wound healing in diabetic mice [46] (Fig. 21.5). Similar results have been described by other groups paving the way to clinical application [45].
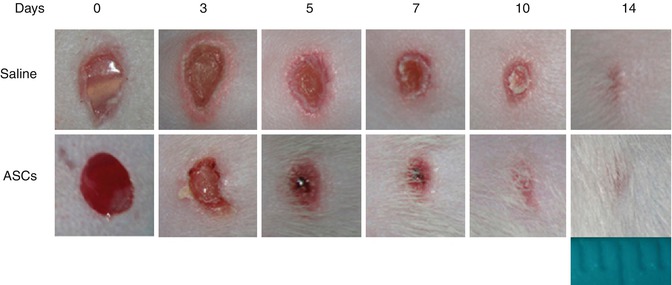
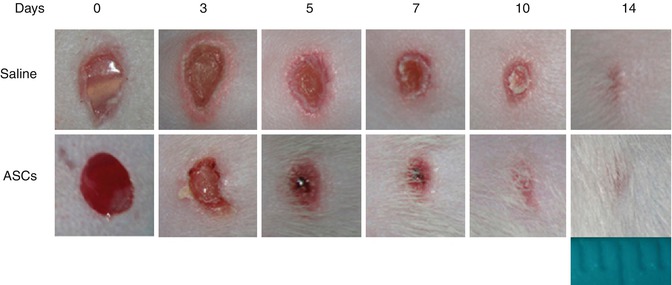
Fig. 21.5
Adipose tissue-derived cells for wound healing enhancement in diabetic mice. Effect of topical administration of ASCs at the lesion site. Representative images from animals receiving saline solution or ASCs (7.5 × 105 cells/mouse) taken immediately after wounding (day 0) and at different time points after injury (Modified from Di Rocco et al. 2011 [46])
Several data indicate the role of ASCs in supporting angiogenesis and wound healing in different experimental and clinical settings [45, 47]. Unfortunately, the heterogeneous nature of the cell population isolated from adipose tissue, as well as the different conditions in the harvesting and isolation procedures, often hampers direct comparison of the results obtained by different groups.
The exact mechanism of action of ASCs in promoting angiogenesis is multifaceted. ASCs release multiple proangiogenic factors and trophic cytokines (Table 21.4) including vascular endothelial growth factor (VEGF), hepatocyte growth factor (HGF), insulin-like growth factor 1 (IGF-1), placental growth factor (PlGF), and chemokine stromal cell-derived factor-1 (SDF-1), which acts as paracrine factors in angiogenesis promotion and in homing and recruitment of circulating EPCs at the damage site [55]. Secreted factors may also play a role in supporting proliferation of resident cells as well as in reducing apoptosis and necrosis at the site of damage or inflammation. ASCs provide antioxidant factors and free radical scavengers. ASCs promote angiogenesis also by direct differentiation into vascular cells which take part actively to vascular remodeling; in addition ASCs may differentiate or fuse with cells which play a role in tissue regeneration (building block function).
Table 21.4
Profile of some of the main factors expressed by adipose-derived stromal cells
Factor | Role in | Reference |
---|---|---|
Vascular endothelial growth factor (VEGF) | Angiogenesis | [48] |
Hepatocyte growth factor (HGF) | Angiogenesis | [48] |
Transforming growth factor-b (TGF-b) | Angiogenesis | [48] |
Basic fibroblast growth factor (bFGF) | Angiogenesis | [48] |
Keratinocyte growth factor (KGF) | Angiogenesis | [49] |
Insulin-like growth factor 1 (IGF-1) | Proliferation | [50] |
Tissue inhibitor metalloproteinase 1 and 2 (TIMP-1 and TIMP-2) | Extracellular matrix homeostasis | [51] |
Nerve growth factor (NGF) | Neurotrophic | [52] |
CXC 5 ligand (CXCL5) | Neurotrophic | [53] |
Macrophage colony-stimulating factor (M-CSF) Granulocyte-macrophage colony-stimulating factor (GM-CSF) | Hematopoiesis | [54] |
Tumor necrosis factor-a (TNFa) | Inflammation | [54] |
Interleukin 6 (IL-6) and interleukin 8 (IL-8) | Inflammation | [54] |
Superoxide dismutase 2 (SOD2) | Oxidative damage protection | [45] |
Pigment epithelium-derived factor (PEDF) |
21.5.4 Increase the Rate of Engraftment of Transplanted Fat
Adipose tissue as autologous material for aesthetic filling assumes a new role today, not only for transplant of mature adipocytes, but also for transplant of ASCs, as an active autologous filler.
In the past, it was believed that the graft should be composed by mature adipocytes, isolated with minimal trauma. Today, after extensive elucidation of the composition of the adipose tissue, we know that mature adipocytes, although occupy most of the volume of the tissue compared to the other cell populations, are not proportionally more relevant. There is a smaller pool of cells, which is fundamental for the creation of homeostatic-like mechanisms, which helps in supporting the graft. Success of the engraftment may be due not only to mature adipocytes but also to more undifferentiated cells present within the adipose tissue stromal vascular faction. In fact, it is becoming increasingly likely that mature adipocytes are nearly completely lost soon after implantation, since they undergo severe anoxia. On the other hand, they may have an important role in supporting the engraftment and in inducing differentiation of adipose tissue-derived progenitor cells. Keeping with this new point of view, several variants to the traditional technique of lipostructure have been proposed aiming at increasing the survival of the fat graft. With no doubt the most significant advancement is represented by the so-called CAL (cell-assisted lipotransfer) developed by Yoshimura’s group. In this procedure autologous adipose-derived stromal cells are used in combination with lipoinjection, in order to increase the rate of fat graft survival and improve soft tissue augmentation [56]. In this way, implanted fat tissue functions as a real living bioscaffold for the implanted ASCs.
Another strategy to use ASCs as filler was proposed by Claudio-da-Silva et al. [57], combining adipose tissue-derived SVFs with hyaluronic acid. With this procedure it was observed both an immediate filler effect (due to hyaluronic acid) but also a longer time persistence of the graft (due to ASCs), with a progressive improvement of skin tone).