Fig. 18.1
Kinetics of tumescent-administered lidocaine into the subcutaneous fat layer (Courtesy of Klein [8])
5.
The time for plasma concentration to approach zero was about 24 h after administration (Fig. 18.1).
6.
Medications alter the liver enzyme cytochrome P-450 used to metabolize lidocaine. The lidocaine dosing needs to be altered to prevent toxicity.
Silkiss and Baylis [9] described injecting fat into the periorbital area, while Chajchir and Benzaquen [10] described injecting suctioned fat into the entire face. Teimourian [11] and Illouz [12] described injecting fat into iatrogenic liposuction deformities, which were unfortunately a frequent occurrence with early liposuction techniques. Although some initial impressions of fat grafting were positive [13, 14], many noted the limited reliability of grafted fat with high absorption rates [9, 12].
Like any good scientist, when the results are less than optimal, a reevaluation of the current method is warranted. This thinking enabled practitioners to improve their aesthetic outcomes. A fat layering technique resulted in an improved result and a long-lasting fat grafting outcome (Fig. 18.2) [15]. The layering of adipocytes into the extracellular matrix scaffold with intact capillary network and enough space between grafted cells for oxygen diffusion during the first 1–2 days, some believe up to 4 days, post-transfer was the probable reason for the improved survival with layering (Fig. 18.3). Others have observed good long-term fat grafting outcomes [16, 17].
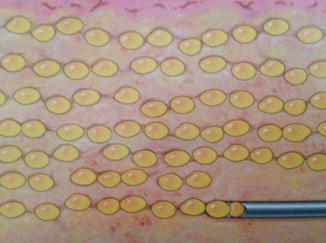
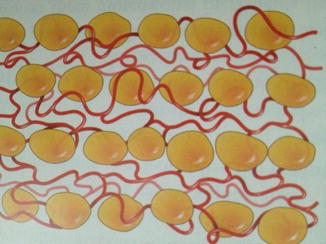
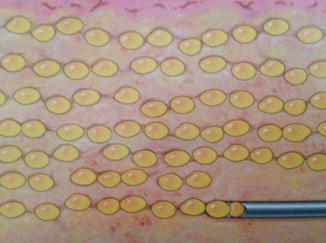
Fig. 18.2
Fat injection by blunt-tipped cannulas in a “layering” technique (Courtesy of Coleman [18])
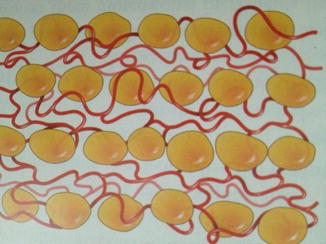
Fig. 18.3
Fat layering technique to optimize vascular access post-transplant (Courtesy of Coleman [18])
Coleman [18] led the resurgence of interest in autologous fat transfer after the observance of fat absorption variability in the 1980s. His fat harvesting, preparation, and injection technique was continually improved upon between 1987 and 1994, modernizing the use of fat for soft tissue augmentation all over the face and body. He developed the Coleman method for reliable harvesting and subcutaneous transplantation of autologous fat (Fig. 18.4) [19, 20]. However, although improving the fat-processing method of his time, the exposure to air and the manual manipulation of the fat, such as pouring off and wicking the oil, produced its own deleterious effects on the fat.
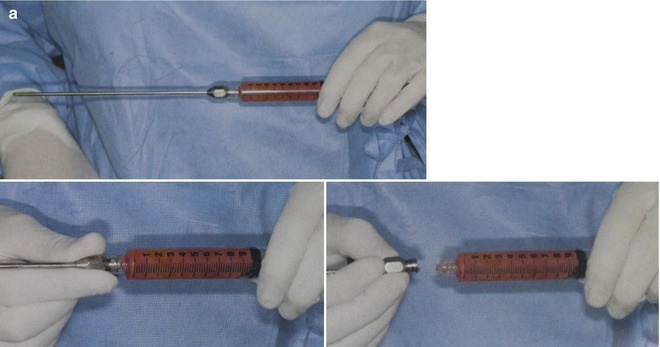
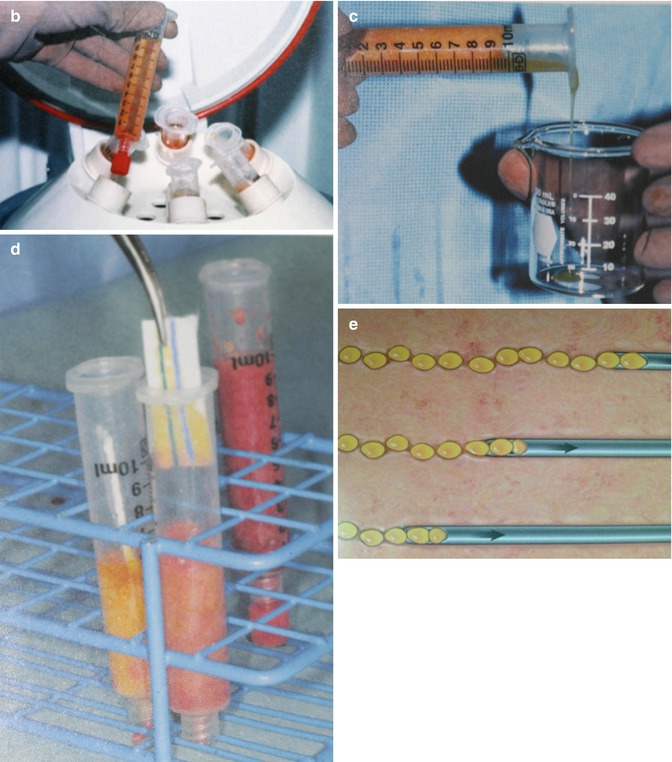
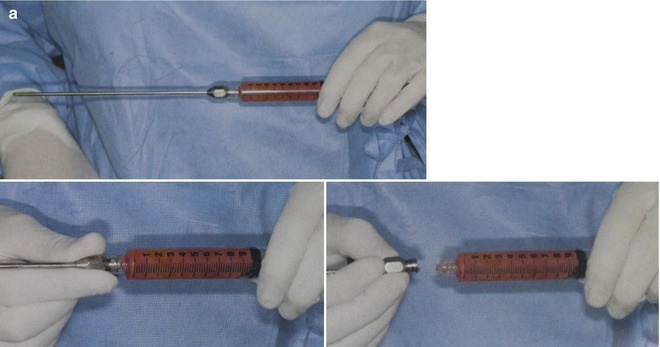
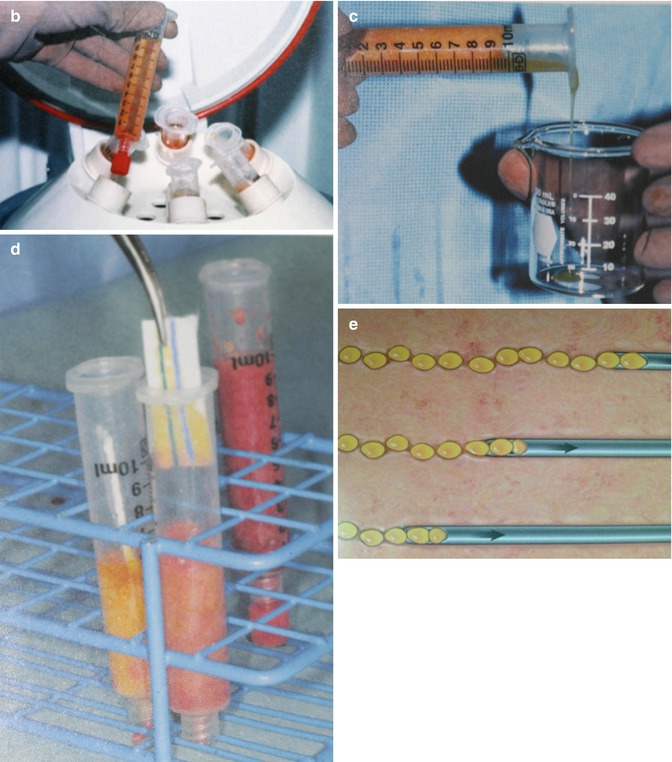
Fig. 18.4
Coleman fat grafting technique. (a) Harvesting with 3 mm blunt-tipped cannula. (b) Centrifugation. (c) Decanting. (d) Wicking of oil. (e) Syringe transfer for blunt cannula microdroplet injection (Courtesy of Coleman [18])
Over the past 25 years, since Coleman’s initial technique descriptions, there has been a dramatic increase in the use of autologous fat grafting for soft tissue augmentation or to treat contour defects in cosmetic and reconstructive surgery. According to the International Society of Aesthetic Plastic Surgery, fat grafting represented 5.9 % of the nonsurgical procedures within aesthetic surgery, with more than 84,000 patients treated in the United States and 514,000 procedures performed globally in 2009 [21].
Although fat grafting is generally safe with good patient satisfaction, many still experience unpredictable outcomes with varying fat cell survival. The vast procedural variations make it difficult to compare outcome studies. Despite this difficulty, there is scientific evidence of numerous variables that can be controlled during the harvesting, processing, and grafting procedure to improve long-term fat viability.
Since an adipose-derived stem cell harvesting technique was described by Zuk in 2001 [22], much research has been conducted, attempting to optimize the harvesting, processing, and applications of ASCs. Thus, the field of cosmetic surgery aligned itself with the field of cellular biology. Coincidentally, it’s no surprise that the current leader in the field of fat and stem cell research, Yoshimura, and his colleagues have training and expertise in both plastic surgery and cell biology. In the author’s humble opinion, Yoshimura and his colleagues’ laboratory with both basic science and clinical research has advanced the field of stem cell medicine more than any other.
The adipose-derived stem cells may differentiate into a number of cells (Fig. 18.5) [23–26]:
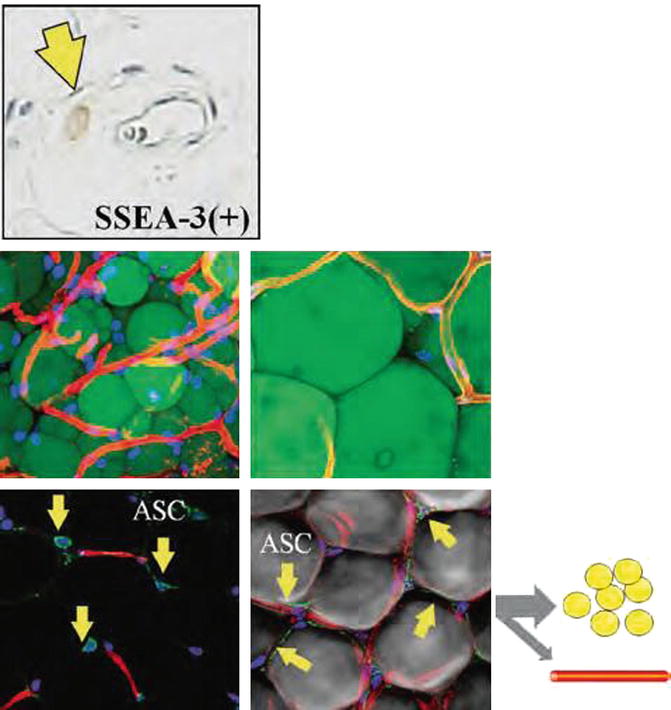
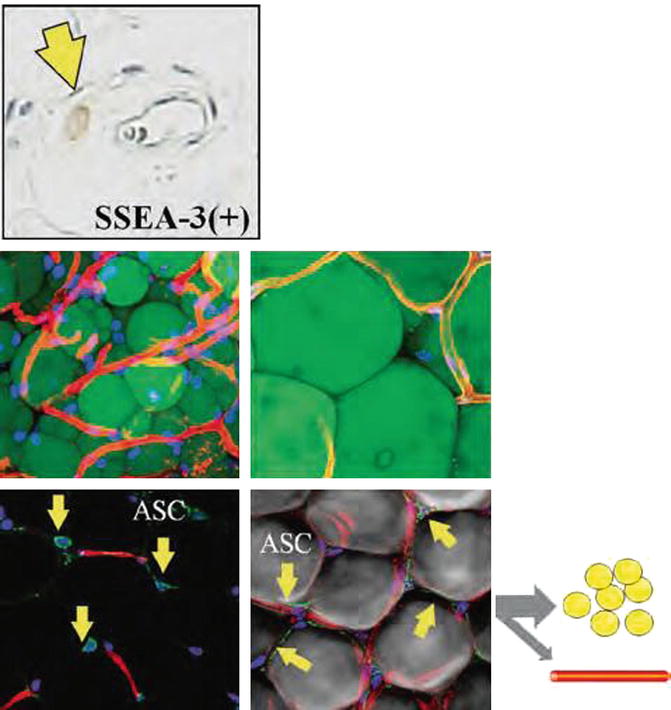
Fig. 18.5
There are a number of different stem cells located in the fat layer with the highest numbers adjacent to blood vessels. (Top) Master stem cells, also known as Muse cells, are multipotent, undifferentiated cells activated under emergency conditions from tissue injury. (Bottom) Progenitor cells (ASC) are monopotent stem cells used in physiological cellular turnover and differentiate into adipocyte and vascular endothelial cell lineages. Lectin (capillary), CD34+ (stem cell), and Hoechst stain (nuclei) (Courtesy of Yoshimura et al. [91])
1.
Adipocytes.
2.
Vascular endothelial and probably vascular mural cells. This process promotes angiogenesis and graft survival.
3.
Other stem cells by mitosis and cell division.
4.
ASCs have the potential to become any of the body’s cells.
Additionally, the stem cells survive as original stem cells and release angiogenic growth factors in response to local tissue hypoxia [27]. The transplanted and the resident mesenchymal stem cells are attracted chemotactically to the grafted area from the inflammatory response. Specifically, platelet-derived growth factors (PDGF aa, bb, and ab) through mitogens of stem cells promote the synthesis of the extracellular matrix. This extracellular matrix is formed through the binding of fibrin monomers and provides a scaffold for the grafted fat and stem cells. Also, transforming growth factors (TGF alpha and beta) from platelets stimulate DNA synthesis and promote cellular proliferation, including those of stem cells [28].
Autologous fat transfer has provided a permanent, naturally feeling, and naturally appearing treatment method for facial rejuvenation and soft tissue augmentation to include natural breast augmentation, gluteal augmentation, hand rejuvenation, and lower-extremity rejuvenation. However, the greatest limitation has been the unpredictability of fat graft survival.
Research continually discovers more information; however, it may subsequently introduce more questions. Some of the questions that need to be answered to optimize fat and stem cell transfer survival include the following:
1.
Is it adipocyte survival or adipocyte cell death and regeneration?
2.
Does one need to add stem cells and growth factors or is the normal wound healing response satisfactory?
3.
Are the stem and progenitor cells more important in regenerating new adipocytes or do they simply cause neovascularization by generating vascular structures to keep grafted adipocyte cells alive?
4.
Does more fat grafted or thicker layers of fat decrease adipocyte survival?
5.
How important is the donor site inherent blood supply and cellular environment?
18.4 Therapeutic Uses of Autologous Fat
The therapeutic uses of autologous fat grafting are for aesthetic soft tissue volume replacement or for reconstruction of soft tissue defects that include the following:
1.
Facial rejuvenation
2.
Facial lipodystrophy
3.
Natural breast augmentation
4.
Breast defect reconstruction
5.
Hand rejuvenation
6.
Gluteal reshaping and augmentation
7.
Lower limb atrophy
18.5 Harvesting
18.5.1 Introduction
Most of the techniques for harvesting cellular material were developed specifically for fat. Adipocytes are less robust than stem cells and require a nontraumatic method of harvesting to prevent cellular injury and subsequent apoptosis or phagocytosis. Simply, harvesting requires two pieces of equipment: (1) a suction source and (2) a collection receptacle.
Suction is achieved using either an external suction machine or the suction forces created by retracting the plunger of a syringe. Suction devices require a sterile collection container in series with the liposuction cannula. The process seems simple at first glance; however, there are numerous variables, including the equipment and the cellular environment, that affect the number and viability of the harvested fat and stem cells. Some of these theoretical variables have been studied, and others have little or no scientific evidence affecting cellular activity.
Every step in fat and stem cell transfer that consists of harvesting, processing, and administration may have deleterious effects on cell viability. The goal is to have the highest number of viable cells for administration. The host’s health is paramount on both the quality of the fat and stem cells harvested and the corresponding recipient local cellular environment.
18.5.2 Harvesting, Processing, and Administration Variables
To simplify the understanding of the effects of the variables on cellular viability, I have classified them under separate headings based on a common feature related to instrumentation or an aspect of the cellular environment (Tables 18.1, 18.2, 18.3, 18.4, 18.5, 18.6, 18.7, 18.8 and 18.9).
Table 18.1
Cellular environment variables
Lidocaine exposure and concentration |
pH of wetting solution |
Epinephrine concentration |
Air exposure |
Temperature |
Supplements |
Hyperbaric oxygen |
Blood contaminants |
Bacterial contamination |
Antibiotics |
Steroids |
Table 18.2
Fat harvesting technique variables
Fat donor site |
Tumescent vs. superwet infiltration technique |
Components of wetting solution |
Harvesting cannulas |
Size (diameter) |
Design (hole geometry) |
Vented vs. non-vented |
Suction tubing diameter and compressibility |
Use of dissectors (Manubat and Blugerman) |
Use of energy delivery devices |
Ultrasound-assisted liposuction (frequency, amplitude, duration) |
Water-jet-assisted liposuction |
Powered-assisted liposuction (PAL) |
Table 18.3
Harvesting suction devices
Simple motorized suction machines |
VASER ultrasound, liposuction, and infiltration system |
LipokitTM fat harvesting and processing unit (Adivive system) |
Water-jet-assisted liposuction device (Body-Jet) |
Table 18.4
Harvesting syringes
Simple syringe |
Simple spring syringe |
Tulip syringe |
Filtered syringes |
LipiVage® |
LipokitTM |
Table 18.5
Fat collection containers
Origins (Sound Surgical Technologies) |
AquaShape Lipo Collector I, II—filter (HumanMed) |
AquaShape Lipo Collector III—no filter (HumanMed) |
Aquavage (MD Resource) |
Red Head (Miami Fat Supply) |
LipoFilter (MicroAire) |
Tissue-Trans Canisters- filter (Shippert Medical) |
Table 18.6
Fat-processing techniques
Gravity separation |
Decanting |
Centrifuge separation |
Washing |
Filtering (syringes and closed canisters) |
Combination techniques (i.e., Coleman technique-manual decanting, wicking oil, and centrifugation) |
Table 18.7
Stem cell processing devices
Maxstem Celltibator (MediKhan) |
UniStation (Advanced Global Bio Medical Solutions) |
Celution One {Cytori} |
Cha-Station and Multi-Station (P&C International) |
Tissue Genesis Cell Isolation System (TGI) |
Table 18.8
Stem cell processing variables
Collagenase vs. no collagenase use |
Stem cell purification and centrifugation |
Cellular storage and culturing |
Cryopreservative used |
Table 18.9
Fat grafting technique variables
Small aliquots versus larger grafts |
Overfilling versus not overfilling |
Multilayered placement |
Muscle versus subcutaneous placement |
Grafting cannula size |
Recipient site |
Serial injections |
Stem cell addition |
Platelet-rich plasma (PRP) addition |
The vast number of potential variables complicates the previous notion of a simple procedure for autologous fat harvesting and transfer. This chapter attempts to summarize the enormous amount of medical literature regarding these variables to arrive at a logical procedure to reach the goal of optimizing fat and stem cell viability for medical administration. Many technique innovations have been devised to overcome cellular viability limitations.
A multitude of cells inhabit the fat layer, although 90 % by volume are adipocytes (Fig. 18.6) [29]:
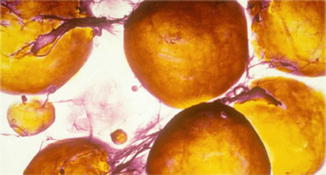
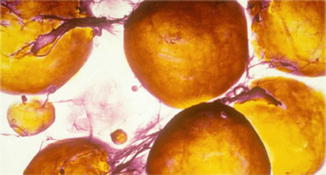
Fig. 18.6
Scanning electron microscopy of human aspirated fat with capillary network
1.
Adipocytes
2.
Adult stem cells
3.
Endothelial cells
4.
Endothelial progenitor cells
5.
Vascular smooth muscle cells
6.
Perivascular cells
7.
Tissue resident macrophages
8.
Lymphocytes
It is estimated that between 2 and 5 % of these cells are stem and regenerative cells [30]. Although they are low in number, these progenitor and regenerative cells certainly have one of the most important impacts on fat transfer viability [31]. They will have a monumental presence as we discover ways that these stem cells will revolutionize medicine.
18.6 Fat Cell Survival Theories
One way to understand the variables in fat cell survival in autologous fat transfer is to work backward from the cellular environment present in the fat- and stem cell-grafted recipient site. However, one needs to understand the stem cell. What defines a stem cell? A stem cell has two primary attributes: (1) differentiation, ability to differentiate into one or more terminally differentiated cell types, and (2) self–renewal, the ability to generate into additional equipotent stem cells.
There seems to be two identifiable theories on fat cell survival via autologous fat transfer. Let us call one of them the Stem Cell Differentiation to Adipocyte Theory and the other the Adipocyte Survival Stem Cell-Assisted Theory. Both theories have cellular biology evidence to support their position.
18.6.1 Adipocyte Survival Stem Cell-Assisted Theory
The main point of this theory is that the majority of adipocytes transferred survive and the stem cells mainly differentiate into vascular endothelial and mural cells and release angiogenic factors, which aid in preventing the adipocyte cellular death.
18.6.2 The Stem Cell Differentiation to Adipocyte Theory
This theory was postulated by the laboratory of Yoshimura and his colleagues at Tokyo University (and Troell, personal communication, January 2011) [32]. The main point of the theory is that the majority of the adipocytes (≈95 %) transferred do not survive and that stem cells become adipocytes. However, the transplanted adipocytes initially need to be alive in the recipient bed. The failing adipocytes send out a chemotactic signal, alerting local mesenchymal stem cells and adipose-derived transplanted stem cells of their upcoming demise. In non-vascularized fat transfer, there are inflammatory signals from both tissue injury (inflammation) and bleeding from the procedure. The dying adipocytes seem to be the most significant signal to induce ASC differentiation into an adipogenic lineage. The fat acts as an essential living scaffold during this process. Angiogenesis promotes a capillary network from differentiation of stem cells to vascular endothelial and mural cells. Larger vessels are thought to be formed by the attraction of bone marrow cells to the local area.
In both theories, if the adipocyte suffers cell death during the harvesting, processing, or grafting process, then the chemotactic signal is either not generated or does not elicit the tissue processes that promote cellular viability. In conclusion, stem cells are vital to the autologous fat transfer survival.
The complex cascade of tissue processes interact like a symphony:
1.
Acute inflammation and wound remodeling
2.
Endothelial vessel formation
3.
Cellular differentiation
4.
Paracrine signaling
5.
Immune modulation—cellular and humoral
6.
Cell death and prevention of cellular death
7.
Growth factors release and their cellular effects
The cellular environment along each step of the fat and stem cell journey from the donor site, through the aspiration cannula, along the suction tubing, propelling the cells into the collection container; the transfer into a processing unit; the environment during processing; the technique and amount of time until administration; and, finally, the recipient bed microenvironment all play a crucial role in cellular survival. If even one of these environments is hostile to the stem cell or adipocyte, cell death may occur. Fortunately, “the manufacturer” ensured that stem and regenerative cells are more robust than adipocytes.
18.7 Harvesting Technique
The main variables of fat harvesting are related to the type of anesthesia (wetting solution only, intravenous sedation, or general anesthesia), donor site, infiltration phase, harvesting cannulas, fat collection receptacles, suction source and amount of suction pressure, as well as use of energy-based systems (Tables 18.1 and 18.2). Studies have shown the highest patient safety when liposuction is performed under local anesthesia (true tumescent technique), followed by that done with intravenous sedation, and, lastly, under general anesthesia. Office based liposuction had a total of 0.07 % serious adverse complications in a dermatologic surgery survey study [33]. No study has compared fat or stem cell viability using different anesthetic methods, with the exception of the wetting solution composition.
18.7.1 Donor Site
Rohrich et al. [34] found no differences in cell viability in harvested fat from the abdomen, flank, thigh, or medial knee. However, Padoin et al. [35] noted increased adipose stem cell numbers in fat from the lower abdomen compared with adipose stem cell levels in the other sampled anatomical locations.
18.7.2 Infiltration Technique
The infiltration phase uses a local anesthetic, usually lidocaine, epinephrine for hemostasis, and bicarbonate to buffer the pH of the wetting solution in a normal saline or lactated Ringer’s solution (Fig. 18.7). Since lactate is converted to bicarbonate in the liver, there is no benefit of using lactated Ringer’s solution over normal saline. Some practitioners have used additives, such as steroids and antibiotics, in the wetting solution during the liposuction process; no study has even shown this method to be of benefit [8]. The infiltration technique relates to the amount of wetting solution infused: either tumescent or superwet techniques. Tumescent technique refers to a 3:1 ratio of infused wetting solution to the quantity of extracted fluid during liposuction compared to a 1:1 ratio using the superwet technique. There is also an amount of time that is recommended to wait until fat extraction is performed after infusing the wetting solution, which is between 30 and 60 min to maximize the amount of fat from liposuction [8]. Klein has even waited up to 2 h prior to performing liposuction to increase the amount of fat to be extracted (personal communication, April 2012). There are no studies evaluating the effects of a tumescent technique versus a superwet technique relating to adipocyte viability.
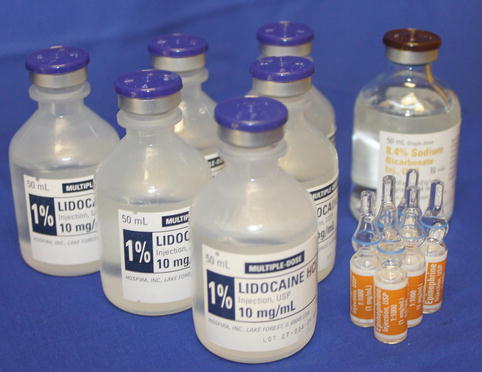
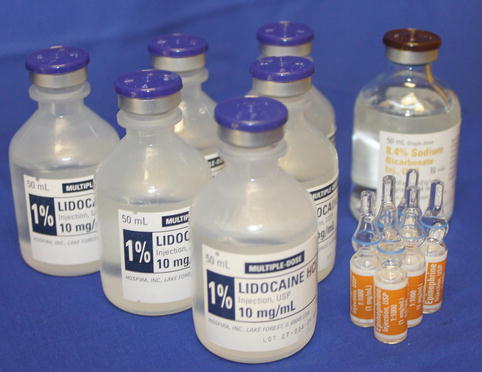
Fig. 18.7
Typical dosing of liposuction wetting solution agents in a 70 kg patient. Lidocaine at 45 mg/kg totaling 3,150 mg using 750–1,000 mg/L wetting solution, epinephrine at 1–1.5 mg/L totaling about 6 mg, and bicarbonate 8.4 % at 10–20 cc/L
Shoshani et al. [36] compared human fat harvested using either normal saline or with a saline solution containing 0.06 % of lidocaine and epinephrine (1:1,000,000) grafted into nude mice. There was no significant difference between the groups in terms of either volume or weight of the fat grafts. Kim et al. [37] studied fat cell viability with different epinephrine doses and found no differences in fat viability. The theoretical maximum dose of epinephrine in liposuction surgery is 0.07 mg/kg or about 10 ampules (mg of 1:1,000 epinephrine).
Moore et al. [38] looked at the effect of epinephrine and lidocaine on human fat viability and found no significant effect on cell attachment in culture, cell morphology, proliferation, or adipocyte metabolic activity. In summary, these studies noted that there was no effect of local anesthetic agents (lidocaine) or epinephrine on adipocyte viability.
18.7.3 Suction Liposuction
Suction devices are divided into syringes and motorized machines (Tables 18.3 and 18.4). Many of the syringes are not only used for harvesting but simultaneous cellular processing.
Shiffman and Mirrafati [39] used various cannulas, needles, suction pressures, external ultrasound, and preoperative massage to determine whether there was an effect on cell viability. There were no appreciable cell membrane disruption from hematoxylin and eosin (H&E) histological examination with suction pressures of −250, –300, or −500 mmHg. About 10–15 % of cells were lost when the suction setting was on or near maximum, –28 in. Hg (−700 mmHg) or one atmosphere. The flaw of this study is that the H&E staining cannot reliably identify viable versus nonviable cells. The study did reveal that cellular damage is caused by external forces affecting the cell membrane from blunt trauma, barotrauma, or biochemical injury.
Pu et al. [40] looked at the cellular function of adipose aspirates and demonstrated decreased metabolic activity using conventional liposuction technique. They also performed a prospective and comparative study between two techniques of harvesting fat, the Coleman technique and the conventional liposuction, on the same patient [41]. The Coleman technique entails fat harvesting with a blunt-tipped 3 mm cannula using a 10 mL Leur-Lok syringe, in which 2 mL of negative pressure space in the barrel of the syringe provides low-level suction; followed by centrifugation at 3,000 rpms for 3 min, expressing the aqueous portion; and then fat preparation steps including decanting oil and manual cotton wicking. A significantly higher quantity of viable adipocytes, determined by the glyceraldehyde-3-phosphate-dehydrogenase activity test, was found in the Coleman technique group.
A review of the medical literature in 2012 titled Fat Grafting: Evidence–Based Review on Autologous Fat Harvesting, Processing, Reinjection, and Storage found no articles in which human fat was harvested with different techniques and then evaluated in a clinical setting [42]. Although sixteen articles were located that included preclinical prospective studies examining harvesting procedures using human fat in vitro and/or in vivo. Table 18.10 shows the comparison of stem cell processing methods.
Table 18.10
Comparing stem cell processing devices
Cha-Station | Multi-Station | UniStation | Celution | Tissue genesis | LipoKit/celltibator | |
---|---|---|---|---|---|---|
Separation time (min) | 30–40 | 60–90 | 60 | 60–90 | 60 | 40–60 |
Automatic type | Semi-auto | Manual | Semi-auto | Automatic | Automatic | Semi-auto |
Contamination prevention | O | X | O | O | O | O |
Weight | 70 | 200 | 70 | 70 | 50 | 50 |
Demo possibility | X | X | X | O | O | X |
Cell count function | O | X | O | X | X | O |
Maximum volume (cc) | 400 | 400 | 800 centr/400 incuba | 400 | 60 | 240 |
Consumable expenses $US | 80 | 60 | 15/syringe | 1,100 | 1,300 | 100/syringe |
SVF (per 1 cc adipose) | 300,000 | 350,000 | 460,000 | 350,000 | N/A | 1,000,000 |
ASC cells (per 1 cc adipose) | 180,000 | 210,000 | 287,274 | 177,105 | N/A | 500,000 |
Cell viability (%) | 85.10 | 90.00 | 90.10 | 86.60 | N/A | 80 |
Samples studied (n) | 2 | 1 | 1 | 6 | N/A | 10 |
18.7.4 Ultrasonic Assisted
The author [43] presented fat harvesting data comparing standard suction liposuction (SAL), water-jet-assisted liposuction (WAL), and ultrasound-assisted liposuction (UAL) techniques in facial fat transfer with 25 patients in each group at the annual meeting of the American Academy of Cosmetic Surgery in 2011, which should be published this year (Fig. 18.8). Laser lipolysis, such as SmartLipo and SlimLipo (Cynosure, Burlington, MA), was not chosen as a study technology since it is thought that laser energy produces nonviable adipocytes. Patient and physician surveys evaluating the percent of grafted fat volumetric survival at four to six months post-transplant revealed 50 and 60 % for SAL, 80 and 80 % for UAL, and 85 and 80 % for WAL. The histological appearance of the lipoaspirates using these three techniques revealed some of the reasons for the studies’ volumetric outcome (Figs. 18.9 and 18.10). The limitation of this study is that there was no radiologic documentation of volumetric changes. In summary, this study revealed that water-jet-assisted and ultrasound-assisted liposuction (VASER mode at 60 % amplitude energy) revealed the highest volume outcomes in facial fat grafting.
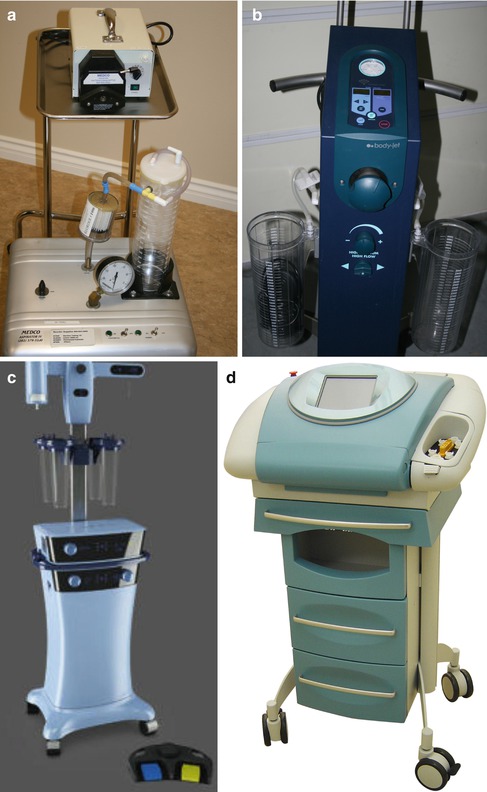
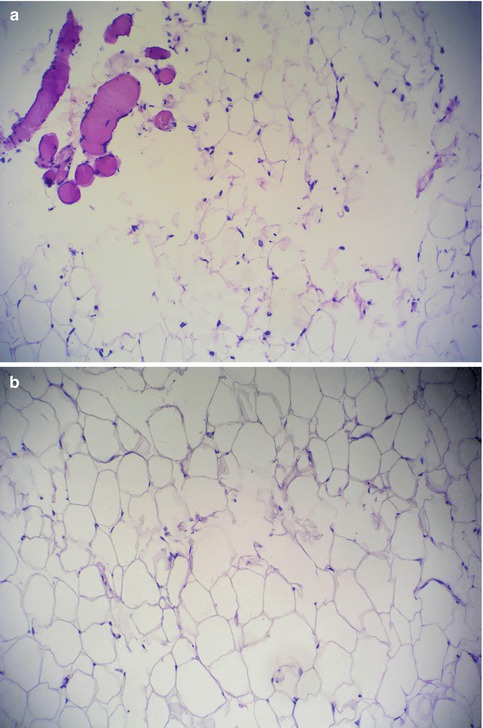
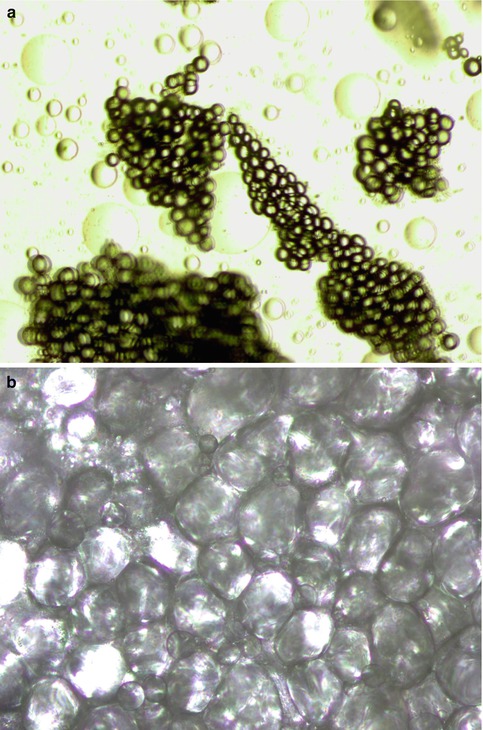
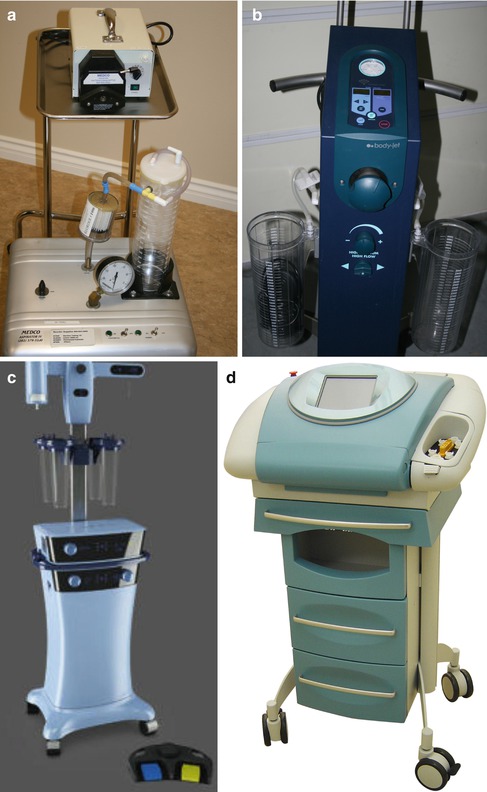
Fig. 18.8
There are four types of liposuction machine technologies. (a) Medco standard liposuction machine. (b) Body-Jet water-jet-assisted system. (c) VASER ultrasound-assisted system. (d) Laser lipolysis, such as SmartLipo and SlimLipo
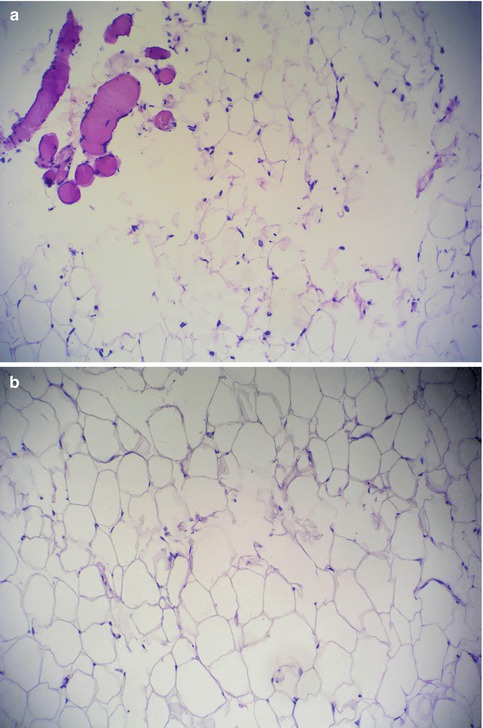
Fig. 18.9
(a) Fat harvested using standard liposuction powered machine. There is evidence of univascular fat cells, swollen cellular membranes, and disruption of connective tissue and blood vessels. (b) The water-jet-assisted device (Body-Jet, HumanMed, Hamburg, Germany) technique. There are more intact cell membranes and normal fat architecture (Courtesy of Meyer H, Hamburg, Germany)
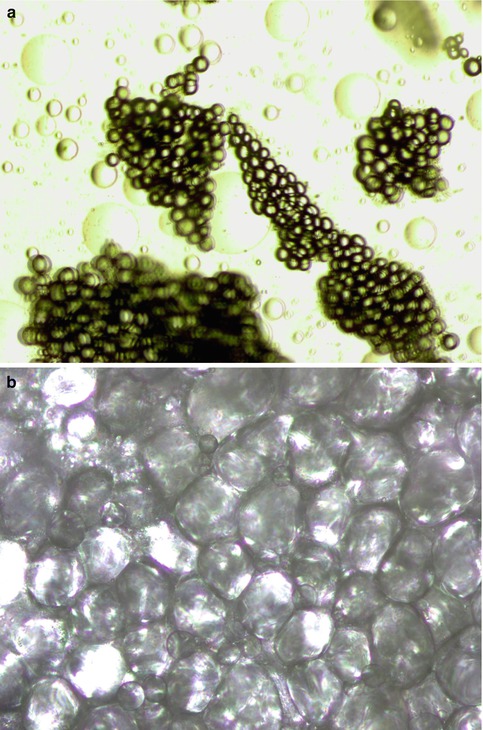
Fig. 18.10
(a) Ultrasound-assisted lipo-harvesting creates smaller aggregates of adipocytes. (b) Using VASER mode at 60 % amplitude, 85 % of adipocytes, and 87 % of stem cells has been shown to be viable for stem-enhanced lipotransfer (CAL)
Rohrich et al. [44] isolated fat using traditional liposuction, internal ultrasound-assisted liposuction, external ultrasound-assisted liposuction, or massage. They found no significant histological or chemical effect of external ultrasound-assisted lipoplasty on adipocytes; however, internal ultrasound-assisted aspirates revealed a thermal liquefaction of mature adipocytes. The ultrasonic levels of this study were from a first-generation ultrasound device, and energy levels were much greater than those used in a recent study by Schafer et al. [45]. In Schafer’s study, cellular analysis revealed 85 % of the adipocytes and 87 % of the stem cells were viable in five patients undergoing lipoaspiration using a third-generation ultrasound device (Valeant Pharmaceuticals International Inc., Quebec, Canada). The energy levels were VASER mode with 60 % amplitude. The ultrasound energy separates adipocytes by a method known as “cavitation” (Fig. 18.11).
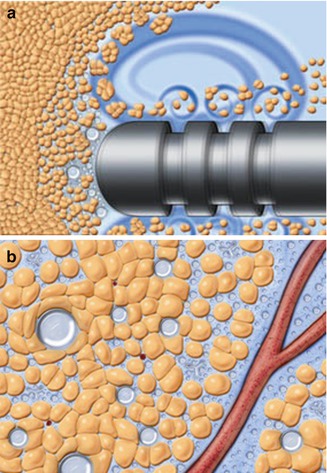
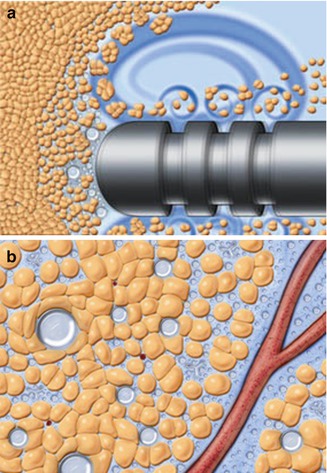
Fig. 18.11
Ultrasound-assisted liposuction mechanism of action is the creation of (a) acoustic streaming and (b) “cavitation.” The dissolved air bubbles from 5 to 10 μm then coalesce to sizes approaching 180 μm. These bubbles reach their maximum surface tension and burst; this energy disrupts the cohesive bonds between fat cells. However, the air bubbles are too large to get between and injury blood vessels and nerves (Courtesy of Solta Medical/Sound Surgical Technologies, Hayward, CA)
Evaluation of cellular composition demonstrated that ASCs were composed of different stromal vascular and hematopoietic subpopulations proportionate to cells obtained from syringe-harvested fatty tissue. The data indicate that adipose tissue acquired using VASER methodology is viable at harvest and suitable as a source of autologous fat graft and ASCs. Vented liposuction cannulas (Valeant Pharmaceuticals International Inc., Quebec, Canada) were used for the harvesting, and this study also supported the potential benefits of a vented cannula, diminishing adipocyte barotrauma.
Rubin [46] used the VASER ultrasound device to harvest human fat using continuous mode at 50 % energy amplitude (approximately 50 % of the maximum energy output of the device), and the fat was transplanted into the back of athymic mice. The mice were sacrificed at 6 weeks post-transplant; the grafts were removed and were measured using the water displacement method. The results revealed that 1.6 mL of the initial 2 mL of grafted fat survived. He did not believe the results and repeated the study with similar outcome. Rubin concluded that there is an 80 % fat graft survival using the ultrasonic harvesting technique. Summarizing these studies reveals that ultrasonic fat harvesting culminates in about 80 % survival by volume of grafted fat and that 85 % of adipocytes and 87 % of stem cells are viable.
18.7.5 Water-Jet-Assisted Liposuction (WAL)
Ueberreiter et al. [47] evaluated the efficacy of WAL for autologous fat transfer used for natural breast augmentation. The procedure was performed under local anesthesia with mild sedation. The fat grafted volume was between 120 and 292.5 mL (avg.184.4 mL) per breast with follow-up at 6 months. Outcome measures used before and after magnetic resonance imaging scans and Brainlab software for volume calculation (±10 %) revealed 76 % (±11 %) fat graft survival (n = 85) by volume.
18.7.6 Syringe Suction Method
The Coleman technique entails fat harvesting with a blunt-tipped 3 mm cannula using a standard 10 mL Leur-Lok syringe with 2 mL of negative pressure space in the barrel of the syringe providing low-level suction. Leong et al. [48] compared harvesting fat using a syringe method with that using a motorized pump-assisted liposuction. They found no difference in cell viability, cell metabolic activity, or adipogenic responses.
Spring-loaded syringes have the advantage of being easier and faster than the manual syringe method; however, they create higher pressures, which increase the risk of cellular injury. The Tulip syringe system, a clip-loaded syringe, has the advantage over the spring-loaded syringes of lower internal pressures. There is a weighted 100 μm filtered syringe that is part of the lipocondensation and lipo-filtering system, known as LipokitTM (Medikan Co. Ltd., Seoul, Korea) (Fig. 18.12).
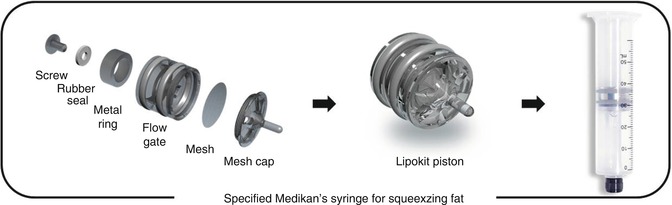
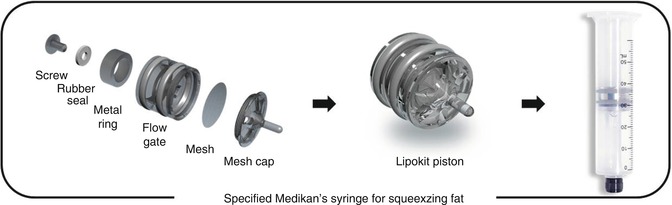
Fig. 18.12
50 mL syringe with a weighted 100 m filter piston plunger used in the centrifuge for both filtering and lipocondensation (Medikan Co. Ltd., Seoul, Korea)
Ferguson et al. [49] demonstrated a higher viable adipocyte count using the LipiVage® syringe filter system (Genesis Biosystems, Lewisville, Texas) (syringe aspiration at low vacuum pressure) compared to conventional liposuction (Fig. 18.13). The LipiVage® filtered syringe is connected to an aspirator at 60 % of maximum suction, about −15 to −18 in. Hg; the fat is cleansed and concentrated in the sterile syringe filtration chamber, but the LipiVage® system does not use centrifugation or decanting.
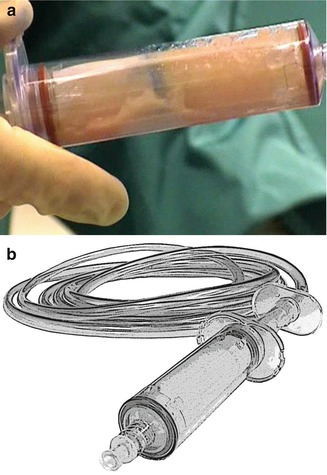
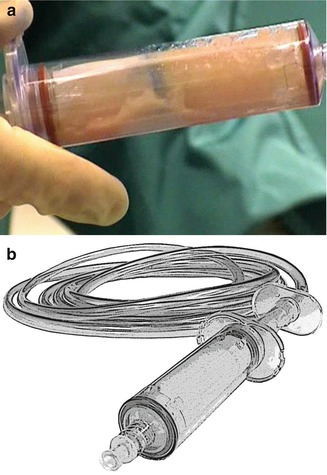
Fig. 18.13
(a) LipiVage® is a disposable fat harvesting 180 μm filtered syringe (Genesis Biosystems). (b) Vented liposuction cannulas (2.2, 2.9, 2.7, and 4.6 mm diameter), VentX for VASER system (Sound Surgical Technologies/Solta Medical, Hayward, CA)
18.7.7 Cannula Size and Style
One of the best manuscripts evaluating the forces of physics produced by a wide variety of instrumentation in suction-assisted lipoplasty yielded many conclusions that can be correlated to the viability of fat and ASCs [50]. Selected clinical measurements were obtained and compared to bench data trends and ratios. They showed that the variation in aspiration rate for different diameter cannulas was small and the flow rate was dominated by the diameter of the suction tubing, with the standard measurements of ¼ to 3/8 in internal diameter (ID) tubing. They discovered that the resistance (R) of any length (L) cannula or tubing is represented by the following relationship: R = L/ID. A shorter larger-diameter cannula had the lowest resistance [50].
A vented cannula, VentX (Valeant Pharmaceuticals International Inc., Quebec, Canada), refers to a cannula manufactured with a very small continuous leak just proximal to the location where the shaft joins the handle (Fig. 18.13). This venting is designed to reduce the relative viscosity of the tissues and fluids in the suction tubing allowing for less resistance and continuous movement of the fluid, potentially reducing cellular barotrauma. Vented cannulas improved aspiration rates compared to non-vented ones in all diameters by decreasing the average viscosity of the fluid. A secondary beneficial effect is that it reduces collection canister splash, which may further traumatize harvested cells [50].
Yoshimura et al. [32] harvested fat using a 2.5-mm cannula or 18-gauge needle at a vacuum lower than 700 mmHg (−28 in. Hg), followed by injection using an 18-gauge needle without significant adipocyte damage. Ozsoy et al. [51] in a prospective study demonstrated a greater number of viable adipocytes with a 4-mm-diameter cannula compared with the smaller 2- or 3-mm cannulas. Erdim et al. [52] also recommended the use of larger cannulas to increase cell viability.
Crawford et al. [53] examined the Viafill system (Lipose Corp., Maitland, Fla.), which uses a hand syringe aspirate at low 50 g-force centrifugation for 2 min versus standard power-assisted liposuction. Significantly higher cell counts were observed when using the Viafill system.
In summary, the body of evidence does not support one harvesting technique above another as superior. However, techniques that use low-pressure suction by means of larger-bore cannulas appear to increase adipocyte viability. If higher pressures are used during harvesting, then it’s best to employ a fat-processing methodology to include filters, washing, and/or centrifugation to remove dead cells and oil. With energy-based systems, ultrasound-assisted liposuction has shown benefits of 53 % better skin tightening and up to 30 % less blood loss than standard liposuction. But, related to fat and stem cell transfer, ultrasound-assisted fat and stem cell harvesting has been shown to have 85 % adipocyte and 87 % stem cell viability, respectfully [45, 54].
18.7.8 Fat Collection Containers
Coleman [55] states in his textbook that exposure of fatty tissue to open air in histological studies has demonstrated cell membrane lysis of up to 50 % of cells exposed for a 15 min duration. Closed collection containers and syringes are now the standard of care (Fig. 18.14), because of the air exposure effects from cell desiccation, bacterial contamination, and cellular death (Table 18.5). Some fat collection containers contain filters, such as the Filtron (Shippert Medical, Centennial, CO) and the Lipofilter (Microaire, Charlottesville, VA). Shippert Medical examined the pore size of a filter and discovered that the optimal pore size was 800 μm, although this size was only nominally better than the 500 μm filter size. It was very effective for removal of oil and extracellular fluid and retained viable adipocytes and stem cells (personal communication, January 2013).
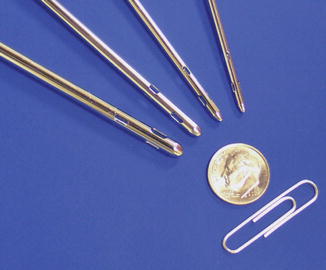
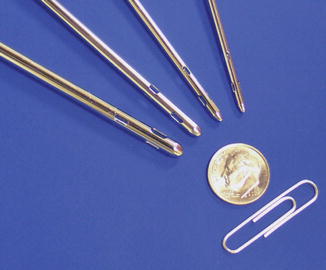
Fig. 18.14
Vented liposuction cannulas (2.2, 2.9, 2.7, and 4.6 mm in diameter), VentX for Caser systems (Sound Surgical Technologies/Solta Medical, Hayward, CA)
18.8 Time to Fat Grafting
Matsumoto et al. [56] compared adipose tissue cell viability from one to 4 h post-harvesting and showed an increasing loss of adipocytes over this time period. Although the scanning electron microscopic assay indicated no remarkable anatomical changes based on preservation methods (Fig. 18.15), oil volume significantly increased in fat preserved at room temperature for 4 h (Fig. 18.16). They concluded that adipocytes should be transplanted as soon as possible if stored at room temperature. All physicians perform the surgical autologous fat harvest at room temperature; thus, the specimens remain at room temperature until processed or grafted. With regard to Yoshimura’s and the author’s technique, fat processing is performed as soon as there is enough fat collected to process and then the fat, with or without concentrated stem cells, is immediately grafted into the planned recipient site.
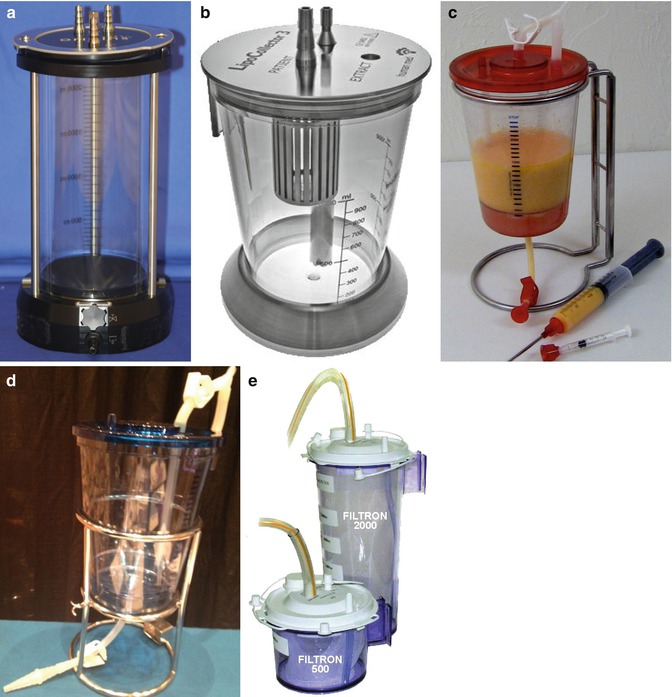
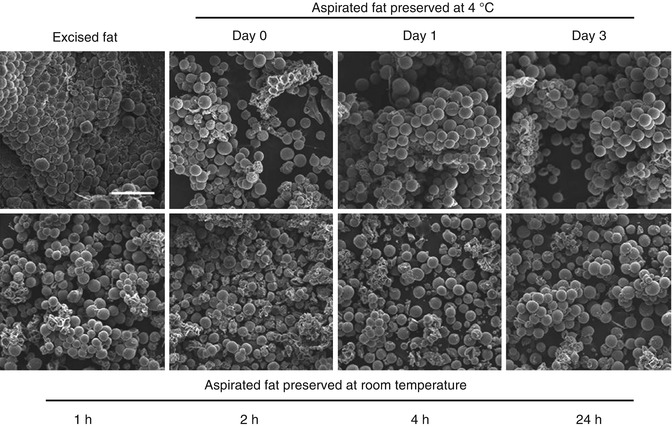
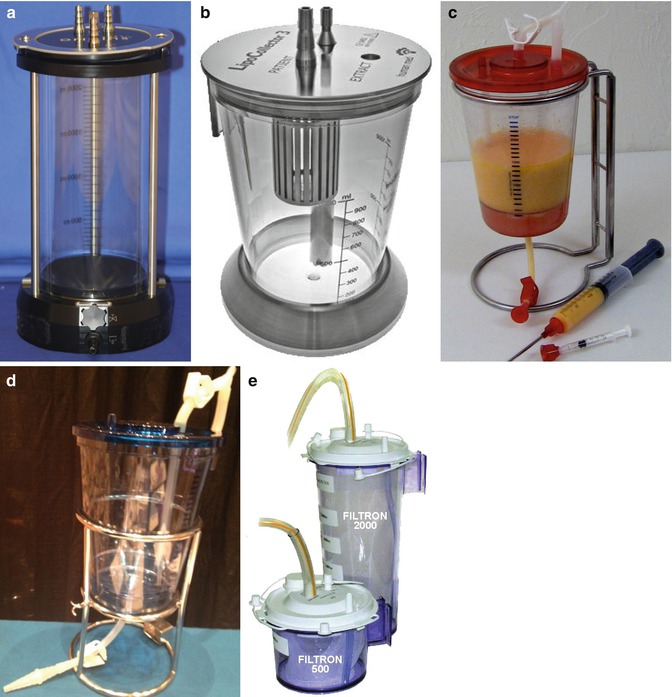
Fig. 18.15
Closed fat collection containers for harvesting. (a) Origins (Solta Medical/Sound Surgical Technologies). (b) Lipocollector III (HumanMed). (c) Redhead (Miami Surgical Supply). (d) LipoFilter (1,588 m filter) (MicroAire). (e) Filtron (800 m filter) (Shippert Medical)
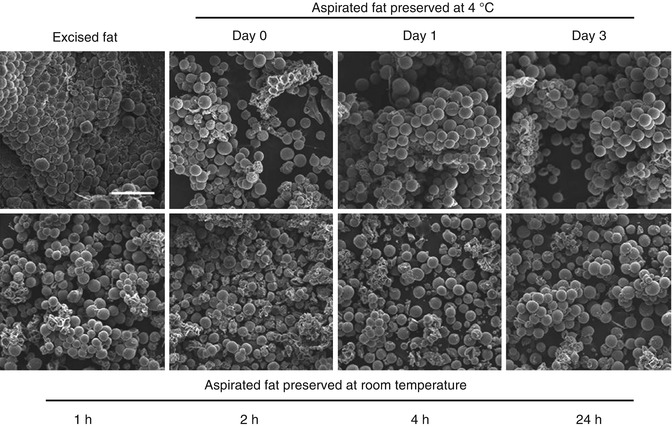
Fig. 18.16
Comparison with scanning electron microscopy of human aspirated fat tissues after preservation at 4 °C or room temperature. (Top, left) Excised adipose tissue was fixed immediately after the operation. (Top, second from left) Aspirated fat tissues preserved at 4 °C were fixed on day 0. (Above, second from right) Day1. (Above, right) Day 3. Those preserved at room temperature were (bottom, left) fixed at 1 h. (Bottom, second from left) 2 h. (Bottom, second from right) 4 h. (Bottom, right) 24 h after the operation. Each sample was treated for evaluation with scanning electron microscopy, and representative photographs are shown. No significant morphological changes over time were found by scanning electron microscopy in aspirated fat, even in samples stored at 4 °C for 3 days or at room temperature for 24 h. Scale bar = 250 m (Courtesy of Matsumoto et al. [56])
18.9 Harvesting Stem Cells: Processing
Stem cell-assisted lipotransfer (CAL) found that liposuction-aspirated fat has approximately half the number of ASCs compared to surgically excised whole fat (Fig. 18.17). They theorized this relative deficiency was due to [57] the following:
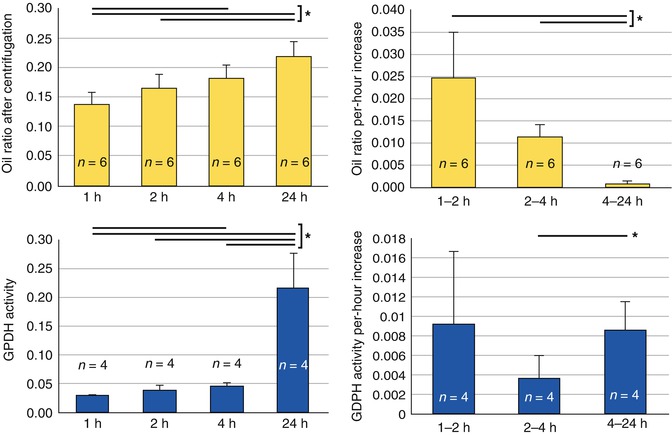
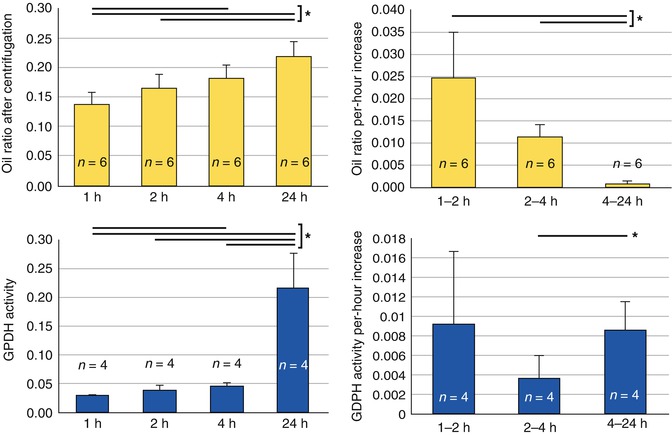
Fig. 18.17
Assessment of damaged adipocytes after preservation at room temperature. (Top) Damaged adipocytes were evaluated by measurement of the oil ratio after centrifugation. (Bottom) Glycerol-3-phosphate-dehydrogenase activity of preserved fat in washing solution. Statistical analysis was performed using paired t tests between groups. Values are mean SE; *p < 0.05. (Top, left) Oil ratios in aspirated fat preserved at room temperature for 1, 2, 4, or 24 h are shown. The oil volume ratio gradually increased with storage time, likely because of the breakdown of adipocytes. (Top, right) The oil ratio per-hour increase slowed remarkably with storage time. (Bottom, left) Glycerol-3-phosphate-dehydrogenase activity of preserved fat in washing solution for 1, 2, 4, or 24 h was measured for assessment of damaged adipocytes. Glycerol-3-phosphate-dehydrogenase activity had slightly increased up to 4 h and was markedly increased at 24 h. (Bottom, right) Glycerol-3-phosphate-dehydrogenase activity increase per hour did not change significantly up to 4 h, but did increase after 4 h (Courtesy of Matsumoto et al. [56])
18.9.1 Centrifugation
There are a number of fat-processing techniques to include gravity separation, decanting, centrifuge separation, washing, filtering (syringes and closed canisters), and combination techniques (Table 18.6). Yoshimura et al. [29] proposed that the short-term survival rate of aspirated adipose grafts per volumetric unit after centrifugation increased with centrifugal forces up to 3,000 g-force. Generally, older fat cells are relatively larger in size than the younger fat cells. The smaller cell sizes are in the majority in the condensed fat from centrifugation, especially using the LipokitTM fat-processing unit (Medikan Co. Ltd., Seoul, Korea). This implies that squeezing the cells by centrifugation enables selective destruction of larger and older cells that tend to be more fragile. Excessive centrifugation can destroy adipocytes and adipose-derived stem cells, but appropriate centrifugation concentrates them. They found that 1,200 g-force is the optimal centrifugal force for obtaining good short- and long-term results in adipose transplantation. Condensation of the graft material and ASCs is thought to be the significant contribution to graft survival enhancement.