Abstract
This chapter is an introduction to laser use in plastic surgery. It is meant as a stimulus to the plastic surgeon who wants to use lasers in plastic surgery or the skin medicine field. The laser is a useful tool in the armamentarium of a cosmetic and reconstructive plastic surgeon. Knowledge of its many uses will complement the skills of the surgeon and facilitate the treatment of the aging process, as well as treating photodamaged skin, birthmarks, and a variety of dermatological disorders such as unwanted hair. Avoiding complications and building a practice are just two of the many ancillary benefits of laser education. There are many good resources to expand on the information presented herein, and a few are listed in the selected references at the end of the chapter. Those wanting to use lasers in medicine would be well advised to join the American Society of Laser Medicine and Surgery (ASLMS). Further information can be obtained online at www.aslms.org.
7 Lasers and Radiant Energy in Plastic Surgery
7.1 Objectives
Introduce the plastic surgeon to the theory and practice of laser use.
Understand the basics of physics and physiology of lasers and radiant energy.
Gain insight into the various laser technologies available and the pathology that can be treated.
Provide the foundation for further study, ultimately incorporating lasers into the plastic surgery practice.
7.2 Introduction
This chapter on “Lasers and Radiant Energy in Plastic Surgery” is meant solely as an introduction to laser use in plastic surgery. It is not meant to be comprehensive, but as a stimulus to the person who wants to use lasers in plastic surgery or skin medicine field. The laser is a useful tool in the armamentarium of a cosmetic and reconstructive plastic surgeon. Knowledge of its many uses will complement the skills of the surgeon and facilitate the treatment of the aging process as well as photodamaged skin, birthmarks, and a variety of dermatological disorders such as unwanted hair. Avoiding complications and building a practice are just two of the many ancillary benefits of laser education.
There are many good resources to expand on the information herein, a few listed in the selected references at the end of the chapter. Those wanting to use lasers in medicine would be well advised to join the American Society of Laser Medicine and Surgery (ASLMS). Further information can be obtained online at www.aslms.org.
7.3 Laser Physics and Physiology
LASER is an acronym for Light Amplification by Stimulated Emission of Radiation. It was first invented in 1960 and has evolved to be a useful tool in medicine as well as in many industrial applications. Conceptually, laser can be defined as a heat generator, as nearly all medical applications of the laser involve the conversion of laser energy into heat. An exception to this generalization would be photobiomodulation (PBM), which will be discussed toward the end of the chapter. The heat generated by the laser energy impacting tissue becomes a stimulus for tissue change. The change due to heating of the tissue may range from simple swelling to charring, and eventually evaporation as the tissue disappears into a plume. Healing of the stimulated tissues then occurs as the body reacts to the stimulus affecting the results which hopefully was the objective of the laser treatment. This is where the knowledge and skill of a laser therapist are essential. 1 , 2
Lasers come in many forms and have many purposes. Not every laser is suitable for every purpose. Only a few of the lasers are commonly used in plastic surgery, which will be reviewed here. Lasers are usually defined by the wave-length of the emitted energy. The emitted energy may be visible light or invisible to the human eye (Fig. 7‑1). The wavelength, intensity, and duration of the laser beam will determine the tissue response. Tissue interaction with the energy is also determined by a variety a tissue factors, including the absorption and spread of the energy. Tissue absorbers, sometimes referred to as chromophores, include water, hemoglobin, melanin, collagen, and other tissue components. Each will react differently depending on the factors described above. These tissue absorbers then become the target, intended, or otherwise. Understanding the characteristics of the target tissue as well as the laser wavelength and parameters is key to achieving a satisfactory result.

7.3.1 Selective Photothermolysis (SPTL)
The concept of selective photothermolysis (SPTL) was introduced by Anderson and Parrish in 1983 to explain the laser tissue interaction. 3 Briefly, the concept states that the combination of laser wavelength, pulse duration, laser energy, and tissue absorption will largely determine the tissue response. A brief explanation of these various factors will help the reader understand many of the laser technologies and techniques. There are many tissue absorbers (chromophores) in the skin, which are competing for absorption of the laser energy. Specific absorption curves can be plotted showing the percent absorption versus the wavelength (Fig. 7‑2). By overlaying these various curves for the major tissue absorbers, one can select the best available laser wavelength and laser, thus optimizing the absorption in the target tissue and minimizing the absorption in the competing chromophores. A tissue target consisting mostly of melanin would require a laser wavelength near the ultraviolet end of the spectrum while avoiding to a large extent water absorption, which is heavy in the infrared portion of the spectrum. The ruby laser (594 nm) would be a good choice for melanin targets, whereas the carbon dioxide (CO2) laser (10,600 nm) in the far infrared portion of the spectrum in general works well on cells containing much water.

A second consideration of SPTL is the pulse duration or length of time the laser energy is impacting the target. Ideally, this time period, known as the pulse duration or pulse length, would be long enough to effectively heat the target and short enough to avoid the spread of heat to the surrounding nontarget tissues. Thus, the thermal relaxation time (TRT) of the specific target should determine the pulse duration of the laser beam. The TRT is proportional to the size of the target. Melanophores, the extremely small, several microns wide packets of melanin, would require a nanosecond pulse, whereas the much larger hair follicle bulbs would require a pulse duration of perhaps hundreds of milliseconds.
The goal of almost every laser treatment is to selectively heat the target to a level sufficient to achieve the given purpose without heating the surrounding nontarget tissue that causes complications. This is where the experience and knowledge of the laser therapist are critical to a good outcome. Other factors can be equally important, such as the patient tissue factors including pigment and patient cooperation. Keeping in mind that every patient is different and conditions vary widely within a given lesion (vessel size, etc.), it is a wonder that a favorable outcome can be achieved.
Several different lasers can be used for a given purpose and different pathologies may respond to different lasers. It is our intent now to examine several of the major laser systems, including their characteristics and uses. This will then be followed by a discussion on the various pathologic problems that can be treated, including which lasers may have acceptable outcome.
7.3.2 Carbon Dioxide (CO2) Laser
Although the first laser was the ruby laser (1960), the carbon dioxide laser was developed early in laser history (1964) and has found extensive use in plastic surgery. In fact, in many practices, the carbon dioxide laser is the main wavelength used as it is excellent for rejuvenating tissue as well as ablating lesions and even cutting tissue with the modest hemostatic quality. At 10,600 nm, this laser falls in the far infrared portion of the electromagnetic (EM) spectrum. It is heavily absorbed by water and minimally absorbed by melanin. Nevertheless, even pigmented lesions respond as the laser evaporates the water-laden cells containing the pigment. 4
Probably, the first cosmetic laser was the Coherent UltraPulse® carbon dioxide laser. This carbon dioxide laser had a short-enough pulse to effectively ablate tissue without leaving major heat damage. Popularity of laser resurfacing grew rapidly in spite of the long recovery following treatment and modestly high incidence of hypopigmentation. Heating caused shortening of the collagen bundles and thus tissue shrinkage was achieved. In addition, heat damage to the tissue cells released cytokines and other tissue constituents which ultimately recruited fibroblasts and collagen production ensued. The reduction of tissue weathering, pigmentation issues, and improvement of fine lines and scars with the carbon dioxide laser caused a rapid advancement of laser use in cosmetic surgery, largely replacing many of the chemical peels and dermabrasion procedures previously done. Many competitors to the Coherent laser appeared and technology rapidly improved with the advent of computerized scanning hand pieces and other developments which speeded the resurfacing, making it more uniform and predictable.
Laser resurfacing of the skin is the most common cosmetic use of the laser in my practice. While frequently done under topical anesthesia as an outpatient procedure, it also can be combined with surgical procedures such as facelifts to complement the restoration of the youthful appearance of the skin. Although the procedure was introduced with a single spot hand piece, a great advance was seen with the introduction of the computerized pattern generator (CPG) which speeded the procedure and made it more uniform and precise.
Laser resurfacing consists of ablation of the cornified layer of the skin and heating of the superficial dermis. As explained above, heating tightens the collagen bundles and initiates production of new collagen in the superficial dermis. In addition to alleviating the weathered appearance of the skin and superficial disorders such as pigmentation issues, smoothing and thickening of the skin are produced. While immediate improvement is seen, final results may not be appreciated for six months or more due to the time required for collagen production. Patients undergoing laser resurfacing may not fully appreciate the improvement, and although healing of the skin limits the depth and intensity of a single treatment, multiple treatments can result in increased improvement.
7.3.3 Fractional Laser Resurfacing
The most recent technological advance for laser rejuvenation skin was introduced in 2004 as fractional photothermolysis. 5 Because of the disadvantages of laser resurfacing such as prolonged healing, pain, need for greater anesthesia, and occasional pigmentation issues, fractional technology has been a significant development in laser resurfacing. Although the results are not as dramatic, reduction in these disadvantages is considerable. Thus, many patients would prefer multiple treatments with fractional laser as contrasted to a single treatment with the conventional laser resurfacing. 6 , 7
Fractional laser resurfacing consists of creating numerous microscopic laser beam penetrations of the skin known as microthermal zones (MTZ). Although the number and density of these MTZ can be adjusted, the concept involves spacing them since there is a microscopic space between each thermal reaction. This is what accounts for faster healing and other advantages. Generally speaking, each MTZ can be deeper than the heating of conventional laser resurfacing. Most laser resurfacing of the skin is currently done with the fractional technique. 8 Although other wavelengths have been used with the fractional technology, carbon dioxide remains the most common and effective in most instances. The concept has been applied to other energy sources other than laser. As in many advances in technology, the concept sometimes becomes bastardized and thus claims of fractional technology may involve something completely different.
7.3.4 Other Applications of the Carbon Dioxide Laser
The carbon dioxide laser is extremely versatile and can be used for a variety of cosmetic applications. Other than resurfacing and rejuvenation of the skin surface, a focused handpiece can be used for ablating lesions, such as seborrheic keratoses, syringomas and rhinophyma. Because of the wide range of energies available with this laser and the modest ability of achieving hemostasis by defocusing the beam, the carbon dioxide laser is extremely useful in plastic surgery. A cutting mode with a focused beam can be used for surgical applications such as blepharoplasty where precision and hemostasis are critical.
7.3.5 Nd:YAG (Neodymium:Yttrium-Aluminum Garnet) and KTP Lasers
Another useful laser in the plastic surgery practice is the Neodymium:YAG (Nd:YAG) and (Potassium-Titanyl Phosphate) KTP laser combination. Although somewhat different in application, these two are together because they originate from the same laser source. Nd:YAG lasers, like the ruby and alexandrite lasers, are crystal lasers. While ruby and alexandrite lasers produce a light in the visible portion of the spectrum, the Nd:YAG is in the near infrared 1,064 nm and thus is invisible to the human eye. This wavelength allows very deep penetration because of the poor absorption by the chromophores in the superficial skin. Thus, targets such as deep, large vessels may be best treated by the Nd:YAG laser. This laser technology is also very stable and reliable. Its versatility has increased by placing a KTP crystal in the beam path of the laser which halves the original 1064 nm wavelength to 532 nm, thus placing it in the green portion of the visible light spectrum. This significantly alters the absorption characteristics of the laser energy. The laser is useful for pigmented and vascular disorders as well as hair removal (Fig. 7‑3).

7.3.6 Ruby Laser (594 nm) and Alexandrite Laser (755 nm)
Both of these crystal-based lasers serve common purposes such as pigmented lesions and hair removal and are thus lumped together for brief discussion. The ruby laser light is heavily absorbed in pigmented lesions, and thus, this laser can be used for most superficial pigmented pathologies. Both of these lasers in the Q-switched version can be used for removing tattoos and other embedded foreign bodies in the superficial dermis.
A number of lasers can be Q-switched, thus changing the effect on tissue. The Q-switched lasers have electronic shutters that allow extremely short (nanosecond) bursts of energy which generally are very high peak power. As stated above, according to the theory of SPTL, the heat is largely confined to the target, thus preventing damage to the surrounding tissue. The high peak power causes rapid buildup of heat within the target, thus creating “microexplosions” which disrupt the tissue target such as tattoo pigment and melanin. It may also require a different delivery system such as a mirrored waveguide rather than a fiber-optic delivery system as with the longer pulsed laser energies.
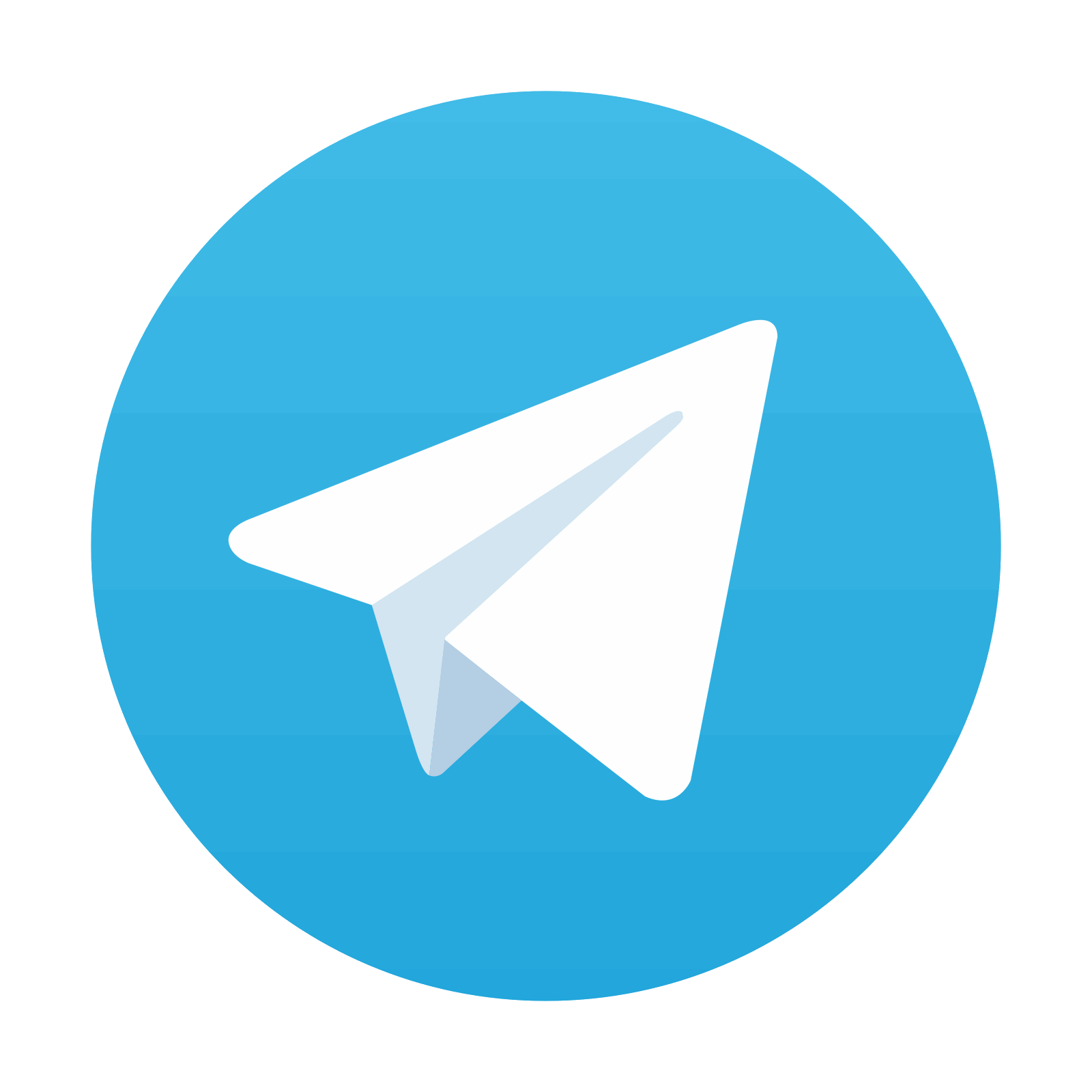
Stay updated, free articles. Join our Telegram channel

Full access? Get Clinical Tree
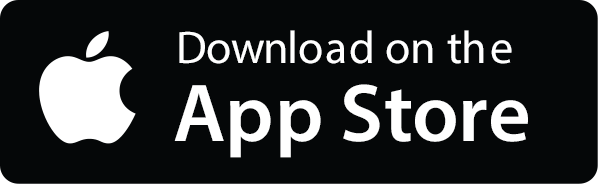
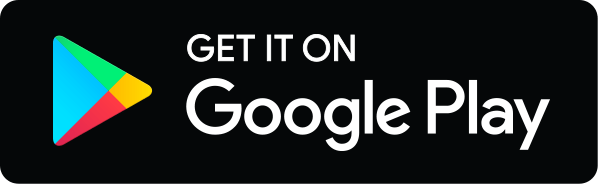
