32 Rhinology in Rhinoplasty
Introduction
The nose is a prominent facial feature and is therefore a major determinant of facial aesthetics. When the shape of the nose is in harmony with the balanced features of an aesthetically pleasing face, attention is directed to the beauty of the eyes. In contrast, an unfavorable nasal shape draws attention away from the eyes, and the face may appear displeasing.
Fascination with the nose in the worlds of art, anatomy, and surgery explains the great interest in rhinoplasty. The nose’s importance in defining facial beauty, its anatomical complexity, the considerable potential for functional deficits, and the important psychological implications associated with nasal deformities make rhinoplasty the most difficult and, at the same time, the most rewarding operation in facial plastic surgery.
The nose also serves important physiologic functions, which must be preserved or, at times, reconstituted if they have been compromised. Basic nasal functions include respiration, air conditioning, filtration, immune defense, and olfaction. An often underappreciated aspect of the nose’s respiratory function is the airway resistance it provides. Comfortable breathing requires a certain amount of resistance to the inspired air. The air conditioning function is responsible for warming and humidification of the inspired air, thus making it suitable for the pulmonary airways. The nose also acts as an effective barrier against airborne particles and pathogens. A number of specific and nonspecific mechanisms contribute to the immune defense of the nasal mucosa and protect humans from external pathogens. The sense of olfaction is best appreciated when it is lacking. Recognition of potentially harmful inhalants can be lifesaving, and the joy of smells and flavors greatly enhances the quality of life.
Anatomical Considerations
Detailed knowledge of nasal anatomy is crucial in order to appreciate, identify, and correctly use the surgical planes of the nose. The nose is built in layers. Exact dissection of the anatomical tissue planes allows for less traumatic surgery with minimal bleeding and postoperative scarring.
The Skin–Soft Tissue Envelope
Nasal skin quality differs from individual to individual. Thin skin may be associated with very visible irregularities from subtle underlying bony and cartilaginous undulations. Thicker skin is usually associated with porosity and a lack of tip definition. Very thick skin is commonly associated with weak underlying cartilaginous structure and resultant tip ptosis. Both extremes of skin conditions make rhinoplasty more difficult, as skin texture and thickness can only be minimally changed with various surgical maneuvers.
The superficial musculoaponeurotic layer of the face is a crucial landmark in cosmetic and reconstructive facial surgery. It extends as a distinct layer superficial to the facial muscles into the nose. Tardy and Brown 1 portrayed the muscles of the nose as encased and interconnected by the superficial musculoaponeurotic layer. The nasal muscles have been categorized into different groups on the basis of their functions ( Fig. 32.1 ). 2 The nasal musculature has important implications for both the cosmetic and the functional aspects of rhinoplasty. Overactivity of the depressor muscle group, including the depressor septi muscle, derotates the tip and results in the unfavorable appearance of a rounded and lengthened tip. This so-called U phenomenon is addressed during rhinoplasty by transecting the insertions of these muscles at the base of the columella.

Some studies suggest that voluntary and involuntary activity of the nasal muscles has an important effect on nasal airway resistance. 3 For instance, paralysis of nasal musculature alone can result in nasal airway obstruction in certain patients. The surgeon should use extra caution to preserve the anatomical integrity and neurovascular supply of these structures to minimize the potential for functional deficits. 3 , 4
The Structural Skeleton
The Valves
Proper postoperative function of the nasal valves is imperative to the success of rhinoplasty. The paired nasal valve areas represent the narrowest portion of the entire airway and account for half the total nasal airway resistance. One must recognize the distinction between the nasal valve area, the internal nasal valve angle, and the external nasal valve. The nasal valve area is the area bound by the caudal end of the septum, the returning portion of the upper lateral cartilage, the lateral nasal wall, the floor of the nose, and sometimes the head of the inferior turbinate ( Fig. 32.2 ).

The internal nasal valve angle is the slitlike area between the caudal end of the upper lateral cartilage and the nasal septum. The internal nasal valve angle should measure 10 to 15 degrees. Cole 5 described four functional components of the internal nasal valve area ( Table 32.1 ).
The external nasal valve is supported by the alar sidewall and is delineated by the nostril rim. It is defined medially by the medial crus of the alar cartilage and inferiorly by the nasal spine and the soft tissues over the nasal floor. 6 The alar sidewall is referred to in the literature as the “fibrofatty tissues of the ala.” The alae display considerable muscular activity. Phylogenetically, the nasal inlet is a derivative of a muscular sphincter, and a considerable proportion of the structural support of the external nasal valve is likely derived from alar musculature.
A study by Ridgway and Murakami 7 elegantly showed the important lateral cartilaginous and ligamentous extensions emanating from the lateral crus and connecting this structure to the piriform crest. Clinically, this structure acts like a firm attachment that can be identified and transected in surgery. Hence we refer to this as the “piriform ligament” ( Fig. 32.3 ). The piriform ligament limits the lateral excursion of the lateral crus during surgical maneuvers, such as the lateral suspension suture. It is therefore important to release this ligament if effective lateralization of the lateral crus and widening of the base of the nasal valve area is desired, as described below in the section “Valve Surgery.” 7

The Septum
Posteriorly, the bony nasal septum is formed by the perpendicular plate of the ethmoid bone and the vomer. Anteriorly, the quadrangular cartilage forms the cartilaginous septum, which is contiguous with the upper lateral cartilages. The five areas of the nasal septum are shown in Fig. 32.4 . For nasal surgery, areas 1 and 2 are of particular importance as these are the areas of the narrowest crosssectional diameter. The configuration and structural support of the caudal septum are of paramount importance for the nasal valve. Further posteriorly, septal spurs and/or turbinate hypertrophy are frequently clinically silent because the large diameter of the posterior nasal cavity compensates for these pathologies. Yet, issues in these more posterior areas frequently contribute to sinus disorders by disrupting sinus outflow and cannot always be ignored.

The Alar and Upper Lateral Cartilages
The interrelation of the cephalic margin of the alar cartilage with the caudal border of the upper lateral cartilage is functionally relevant. In younger patients, the alar cartilage overrides the caudal end of the upper lateral cartilage, which typically curls cephalically to varying degrees ( Fig. 32.5 ). 8 Derotation of the nasal tip, as observed with senile tip ptosis, has a constricting effect on the nasal valve. Almost invariably, reconstruction of the caudal end of the septum, rotation of the nasal tip, and cephalic advancement of the dorsal skin soft tissue envelope form the foundation of successful correction.

The Bony Skeleton
The shape of the anterior nasal spine and the width of the piriform aperture are relevant with regard to nasal valve function. Deviations of the anterior nasal spine may result in deflections of the caudal end of the septum, while widening of the spine may cause clinically important constriction of the mediobasal aspects of the nasal valve area. The bony piriform crests have important ligamentous relations with the lateral crura of the lower lateral cartilages, as shown in Fig. 32.3 . The position of the piriform crests determine the width of the alar base. Too narrow an alar base may cause clinically important constriction of the laterobasal aspects of the nasal valve area.
Support of the Cartilaginous Skeleton
The structural support of the nasal tip is crucial in maintaining functionally patent nasal valves. The caudal end of the septum is of particular importance. Luxation off the anterior nasal spine, caudal deviations, thickening of the caudal end, insufficient caudal or anterior projection, or blunting of the anterior septal angle may contribute to nasal valve collapse and obstruction. Alar collapse may result secondarily from anatomical deficiencies of the central pedestal. Clinically, these deficiencies are frequently associated with deprojection and derotation of the nasal tip.
Fig. 32.6 shows the anatomical configurations of an intact cartilaginous skeleton and one where a deficient anterior septal angle results in tip ptosis and valve collapse. Primary causes of alar collapse include weak, medially displaced, or long lateral crura, or twisted lower lateral cartilages. Secondary causes frequently involve overresected lower lateral cartilages from primary rhinoplasty. Accurate diagnosis of the contributing anatomical factors is essential for a targeted surgical approach.

The Turbinates
The turbinates consist of three functionally distinct tissue layers: the turbinate bone, the submucosa, and the mucosa. Each component can make up for variable proportions of turbinate volume. While the mucosa is the thinnest layer, both the submucosa and the bone can take up the greatest proportion of turbinate volume ( Fig. 32.7 ). The submucosa serves very important functions: it regulates airflow through alternating congestion and decongestion. Moreover, it provides energy and metabolites to warm and humidify the inspired air. The relatively thin mucosa acts as an interface and mediates the exchange of temperature and moisture with the nasal airflow. The mucosa is also responsible for ciliary function and proper flow of secretions through the nose from front to back. The turbinate bone is the functionally least important component of the turbinate. It stabilizes and supports the turbinate.

While the interface function of the nasal mucosa is without doubt important, the terms “mucosa sparing” or “submucosal” have led to an important misconception in the study of turbinate surgery. These terms are frequently utilized to denote presumably less invasive techniques of turbinate volume reduction. The authors argue that the submucosa is equally deserving of a conservative approach, because it is crucial in maintaining the energy-intensive physiologic functions of the nose, including warming and humidification.
Turbinate reduction techniques like laser or electrocoagulation, shaver treatment, and others cause significant damage to the submucosa. While it is widely accepted that a critical loss of perfused endonasal soft tissue can result in chronic atrophic rhinitis, no data are available to quantify how much endonasal mucosa can be safely sacrificed without longterm risk of clinically apparent loss of function. Such data are equally deficient for the submucosa. The authors therefore advocate preservation of mucosa and submucosa when performing reductive turbinate surgery. This is achieved in the authors’ practice by simple outfracture or selective resection of turbinate bone in the great majority of cases, as outlined in the section “Turbinate Surgery.”
Histology of the Endonasal Epithelium
Various types of epithelia line different aspects of the nasal cavity. In the vestibule, moderately keratinized squamous epithelium predominates. Immediately posterior, a transition zone about 1 cm wide may be lined with squamous epithelium, transitional epithelium, or pseudostratified columnar epithelium, followed by the typical pseudostratified, ciliated, columnar epithelium with goblet cells of the middle and posterior third of the nasal cavity. The cilia beat with a frequency of 10 to 20 Hz to move the mucous blanket with variable velocity between 2.3 and 23.6 mm/min. 1 The olfactory cleft is covered by olfactory mucosa, an area of about 200 to 400 mm2, and is much thicker than the surrounding respiratory epithelium. The olfactory epithelium is pseudostratified and columnar, and contains the bipolar olfactory sensory neurons. The four main cell types identified in the olfactory mucosa are ciliated olfactory receptors, microvillar cells, sustentacular cells, and basal cells.
The Paranasal Sinuses
A thorough discussion of the anatomy of the paranasal sinuses is beyond the scope of this chapter. However, for successful rhinoplasty, it is essential to be able to accurately diagnose and treat concomitant paranasal sinus pathology. A complete review is available in the excellent texts by Draf, Kennedy, Stammberger, and Hosemann. 9 , 10 , 11 , 12
Nasal Physiology
Nasal Cycle
Without a sound understanding of the nasal cycle, the clinician may be easily misled in the diagnostic workup of nasal obstruction. The nasal cycle is a function of the nasal submucosa. It was described by Kayser in 1895 and is present in 72 to 80% of the human population. With remarkable regularity, cyclical changes in the crosssectional lumen of the nasal cavities reciprocate approximately every 3 to 4 hours. These changes generally go unnoticed by the patient, because the combined nasal airway resistance of both sides remains constant. 13 It is important to understand that the nasal cycle may result in near-total unilateral nasal obstruction on inspection or imaging, a finding that must not be confused with pathologic nasal obstruction. The function of the nasal cycle has been hypothesized to allow for regeneration of the nasal mucosa on the obstructed side. This would suggest that warming, humidification, and other functions challenge the nasal (sub-)mucosa so greatly that these functions cannot be maintained in an uninterrupted manner. Unfortunately, no data are available to quantify the functional residual capacity of the nose. It is therefore unknown how much submucosa or mucosa can be lost before function of the nose is compromised. Further research is needed to better elucidate this important issue as well as the purposes and meaning of the nasal cycle.
Olfaction
The sense of olfaction combines neural input from the olfactory, trigeminal, glossopharyngeal, and vagus nerves. For a particular odorant to stimulate the olfactory nerve, it must be transported by endonasal airflow toward the olfactory cleft. This transport appears to be enhanced during sniffing, as inspired airflow is increased and may be directed better to the olfactory epithelium. Retrograde airflow is also suitable to transport airborne molecules derived from food in the pharynx toward the olfactory epithelium. Thus, the sense of olfaction can contribute to the flavor of food. Olfaction is temporarily compromised after rhinoplasty and recovers within 6 weeks to 6 months after surgery. 14
Filtering Function
The nasal mucosa efficiently protects the lower airways from inhalation of particulate matter. Multiple studies have investigated both deposition (uptake, absorption, retention) and regional distribution in the nose. Particle size, shape, specific weight, and aerodynamic properties determine the degree of particle deposition on the nasal mucosa. About 60% of particles 1 µm in diameter are deposited in the nasal cavity, and the deposition of larger particles is more complete. 15
Two major deposition sites have been identified in the nose. As a result of the transition from laminar to turbulent flow, particles are deposited on the mucosa posterior to the nasal valve. The direction of the endonasal airflow subsequently directs particles to the second site of predominant deposition, the anterior aspect of the middle turbinate. 16 The endonasal distribution of nasal tumors has been correlated with these particle deposition patterns. This deposition pattern may also explain the preponderance of middle turbinate edema in allergic rhinitis patients, and the common initial formation of nasal polyps most commonly at the middle turbinate region.
Humidifying Capacity
The relatively small surface area of the nasal mucosa (120 cm2) and the underlying submucosa are faced with the formidable task of humidifying and warming about 14,000 L of inspired air that pass through the nasal cavities of a normal active adult in 1 day. The submucosa allows for energy and metabolites to be transported to the mucosa, which acts as the interface for exchange. Air is heated by conduction, convection, and radiation. The heat exchange is efficient, because the blood flow is in the opposite direction to the incoming airflow. In comfortable room conditions, about 280 kJ of energy are required to warm the air to 32°C in the rhinopharynx. To saturate this volume of inspired air with humidity, 1,400 kJ of energy and 600 g of water are expended. On expiration, a considerable proportion of warmth and humidity are extracted from the air to conserve energy. 17 , 18 , 19
Airflow, Resistance, and Its Regulation
The nose acts as a dynamic resistor to inspired air. The speed of the inspired air at the entrance of the nasal cavity is about 2 to 3 m/s and rises to about 12 to 18 m/s at the internal nasal valve. Here, the airflow makes an upward angulation of approximately 60 degrees, hence the term “upstream resistors” to describe the area of the nasal valve. Posterior to the nasal valves the speed diminishes to 2 to 3 m/s, and the flow becomes more horizontal and eventually tilts downward toward the choanae. More air passes through the middle meatus than the inferior meatus. As the airflow becomes turbulent, warming and humidifying of the inspired air is enhanced. When entering the nasopharynx, the airflow tilts downward, becomes laminar, and increases in velocity to 3 to 4 m/s. These generalizations of intranasal airflow have been concluded from predominantly static models. It is important to understand that the regulation of nasal resistance is a dynamic process.
A liquid or gas flowing through a tube increases its velocity and diminishes the transmural pressure at an area of constriction. This phenomenon, referred to as the Bernoulli effect, explains the observation that the nasal valve collapses to varying degrees on inspiration. When nasal valve collapse becomes premature, pathologic airway obstruction ensues. Three factors determine the collapsibility of the nasal valves: the overall crosssectional area of the nasal valve, the shape of the nasal valve area, and the resilience of the structural skeleton. The smaller the nasal valve area is, the greater the velocity of the inspired airflow and the negative transmural pressure become; the more slit-like the nasal valve area is, the more prone it is to collapse; the weaker the skeletal structures are, the easier they will collapse. The theoretical ideal would be a nasal valve with a more rounded, large valve area and a structurally firm skeleton.
The alae represent the softest part of the nasal tip, followed by the columella, interdomal region, and the anterior septal angle. 20 The midpoint of the ala is the location most prone to collapse. This is due to leverage—this point is most distant from the structural fulcra of the nasal valve: the anterior septal angle and the alar base.
Further studies have shown that the nasal muscles profoundly influence the structural resilience of the nasal valve. An involuntary resting tone adds stiffness to the ala, and this is further increased by voluntary activation of the nasal musculature (flaring). As evidenced by rhinomanometric measurements, the involuntary (resting) muscle tone decreases nasal airway resistance. Voluntary flaring further opens the nasal airway. 3 This phenomenon explains why people with cranial nerve VII paralysis may suffer from new-onset nasal obstruction.
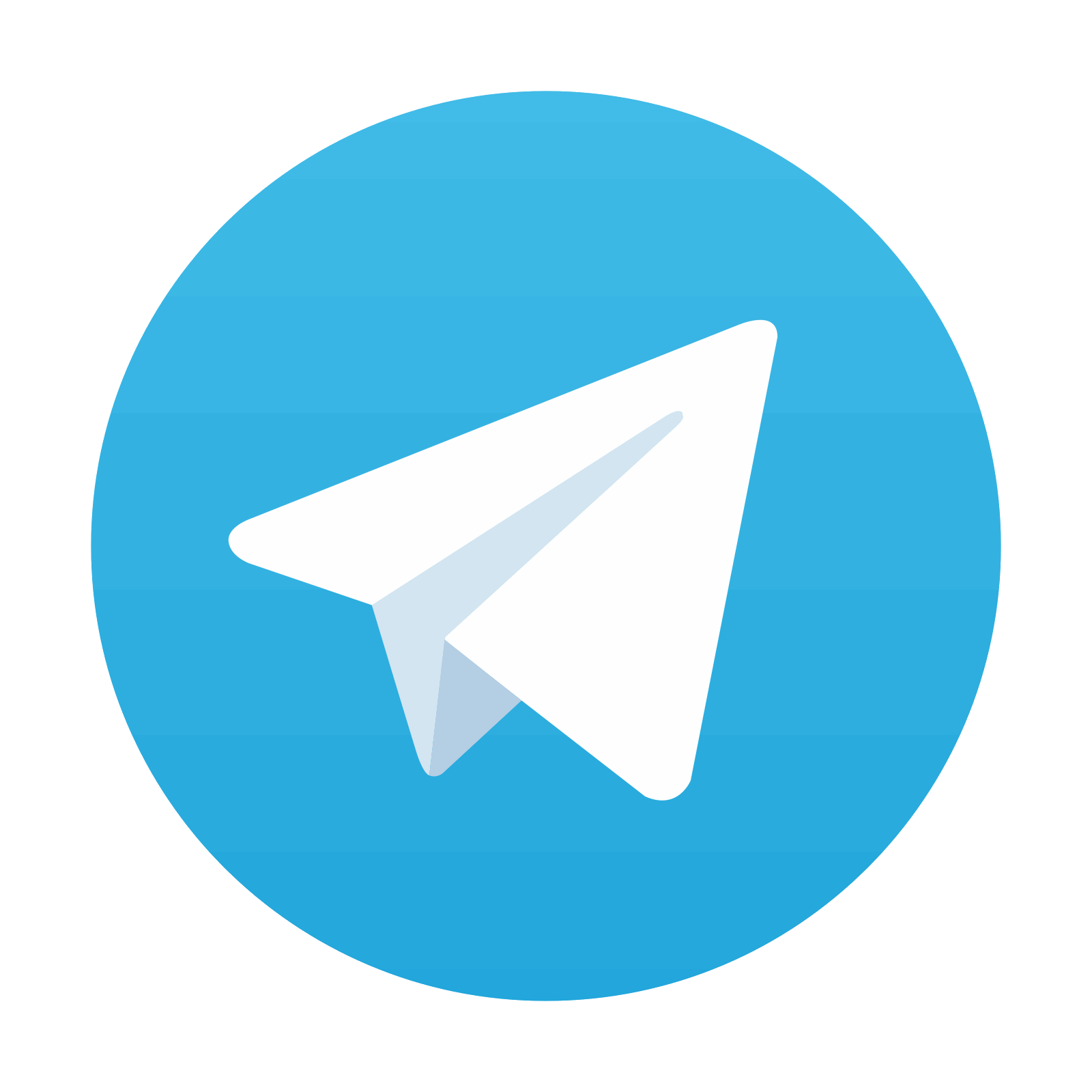
Stay updated, free articles. Join our Telegram channel

Full access? Get Clinical Tree
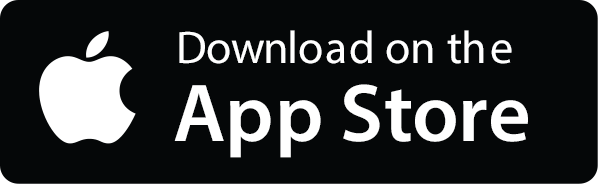
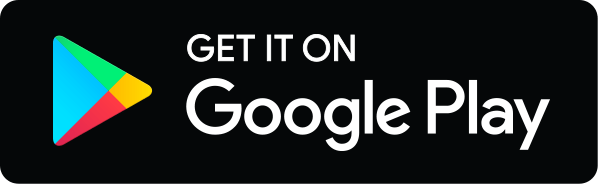
