History.
Prior to the 20th century, there were occasional observations on ‘aurantiasis’ and ‘carotenosis cutis’ as forms of xanthodermia that were different from jaundice. In 1926, Greene & Blackford [3] showed that it was possible to distinguish carotenaemia from jaundice by shaking serum with equal volumes of petroleum ether and absolute alcohol. Carotene colours the upper petroleum ether layer whereas bile pigments are found in the middle alcohol layer. The food rationing of the Second World War provided the background to a series of observations on dietary carotenaemia. In the UK, there was a successful campaign promoting the consumption of root vegetables (particularly carrots and swedes) and, in 1941, Almond & Logan [4] described four housewives who developed orange pigmentation of their palms and nasolabial folds following the ingestion of 2.7 kg of carrots weekly for 7 months. One of the four women was breastfeeding an infant and this infant also developed pigmentation, which disappeared following a change to bottle feeding. In 1958, Lord Cohen of Birkenhead [5] reported 50 cases of xanthodermia diagnosed in the war years in the UK and associated with a raw carrot intake of 1.8–3.6 kg daily. Xanthodermia appeared after 6–8 months, but if carrots were omitted from the diet the yellowish tinge faded in 2–6 weeks. Lord Cohen also described carotenaemia in hyperlipidaemic states (including diabetes mellitus, the nephrotic syndrome and myxoedema). He was the first to describe a case of carotenaemia with low plasma vitamin A concentration and postulate that this was due to an inborn error in the conversion of β-carotene to vitamin A [5].
Aetiology and Pathogenesis.
A normal intake of β-carotene is 900–1200 µg/day. Ingested carotene is largely converted to vitamin A (retinol) in the intestinal mucosa by carotenoid 15,15′-mono-oxygenase and retinaldehyde reductase (see Fig. 171.1). A small proportion is absorbed unchanged. The absorption of β-carotene is increased in subjects taking a high-fat diet. The highly lipid-soluble pigment is present in the lipoprotein fractions of plasma (particularly low-density lipoprotein, LDL) and there is a close correlation between serum lipoprotein levels and serum β-carotene concentration [6]. Carotenaemia or hypercarotenaemia can therefore arise as a result of excessive ingestion of β-carotene, defective conversion to vitamin A (metabolic carotenaemia) or hyperlipidaemia.
Excessive Ingestion of Carotene
Foods with a high content of β-carotene [7] are listed in Table 171.2. Ingestion of large amounts of any of these carotene-rich foods could give rise to carotenaemia. It is important to remember that β-carotene is also used as a food additive; its presence is usually declared on the package (as β-carotene or E160). Prince & Frisoli [8] have studied the accumulation of β-carotene in the plasma and skin in adult volunteers given a large daily dose of the pigment. The plasma concentration rises to a plateau over 9–10 days. Accumulation in the skin takes a further 2 weeks. On cessation of administration, plasma carotene concentrations decay, with a half-life of around 15 days [8].
Table 171.2 Carotene content of foods
Moderate (50–250 µg/100 g) | High (300–600 µg/100 g) | Very high >600 µg/100 g) |
Vegetables | ||
Aubergine | Asparagus | Cabbage (Savoy) |
Baked beans | Broccoli | Carrots |
Broad beans | Brussels sprouts | Chilli (red) |
Cauliflower | Courgette | Cress |
Celery | Green beans | Curly kale |
Fennel | French beans | Mange-tout |
Marrow | Leeks | Peppers (red) |
Peas (sugarsnap) | Peas (tinned/frozen/fresh) | Parsley |
Peppers (green/yellow) | Petit pois | Pumpkin |
Runner beans | Squash (acorn) | Spinach |
Swede | Spring greens | |
Sweetcorn | Squash (butternut) | |
Sweet potato (white flesh) | Sweet potato (orange flesh) | |
Tomato (fresh/tinned)* | ||
Tomato purée* | ||
Watercress | ||
Yam (yellow flesh) | ||
Fruit | ||
Apricot (canned in syrup) | Peach (dried) | Apricot (dried) |
Banana | Plum | Melon (canteloupe, flesh) |
Blackberry | Watermelon* | |
Blackcurrant | ||
Orange |
* Major carotenoid is lycopene.
Hyperlipidaemia
The concentration of β-carotene in the blood rises when the lipoprotein concentrations rise. The conditions which have been documented as giving rise to carotenaemia include diabetes mellitus (which usually gives rise to increased plasma very low-density lipoprotein or VLDL), hypothyroidism (increased LDL) and the nephrotic syndrome (increased LDL and VLDL) [5]. Other causes of hyperlipoproteinaemia in childhood include obstructive jaundice, hepatic glycogenoses, renal dialysis, acute porphyria, cholesterol ester storage disease and the primary hyperlipidaemias [9]. It has also been reported in some patients with anorexia nervosa [10]. The mechanisms responsible for hyperlipidaemia in these conditions are beyond the scope of this chapter. In hypothyroidism the conversion of β-carotene to vitamin A is reduced so that this is an additional mechanism for the carotenaemia.
Metabolic Carotenaemia
The combination of an elevated serum carotene concentration and low serum vitamin A suggests a defect in one of the enzymes responsible for conversion of carotene to vitamin A. To date, one such disorder has been described at a molecular genetic level and others require further clarification.
Carotenoid 15,15′-Mono-Oxygenase (BCMO1 or CMO1); Gene Locus 16q21-q23.
Carotenoid 15,15′-mono-oxygenase (CMO) catalyses the first step in the conversion of dietary provitamin A carotenoids to vitamin A and a single patient with a confirmed mutation in this gene has been reported [11]. This subject, who did not ingest excess dietary carotene, had yellow discoloration of the skin but was otherwise healthy and had elevated serum β-carotene and low to normal serum vitamin A. A single T170M heterozygous mis-sense mutation was identified resulting in a 90% loss of function of the CMO1 enzyme. Although the function of this threonine residue is not understood, substitution of a small hydrophilic threonine with a large hydrophobic methionine residue severely attenuates enzyme activity. As the mutation was only found in a single allele, other genes known to be involved in carotenoid metabolism and vitamin A synthesis were also analysed in this subject and were found to be normal (carotenoid 9′,10′-mono-oxygenase, cellular retinol binding protein I and II and retinol dehydrogenase types 11, 12 and 14). Thus haploinsufficiency of CMO1
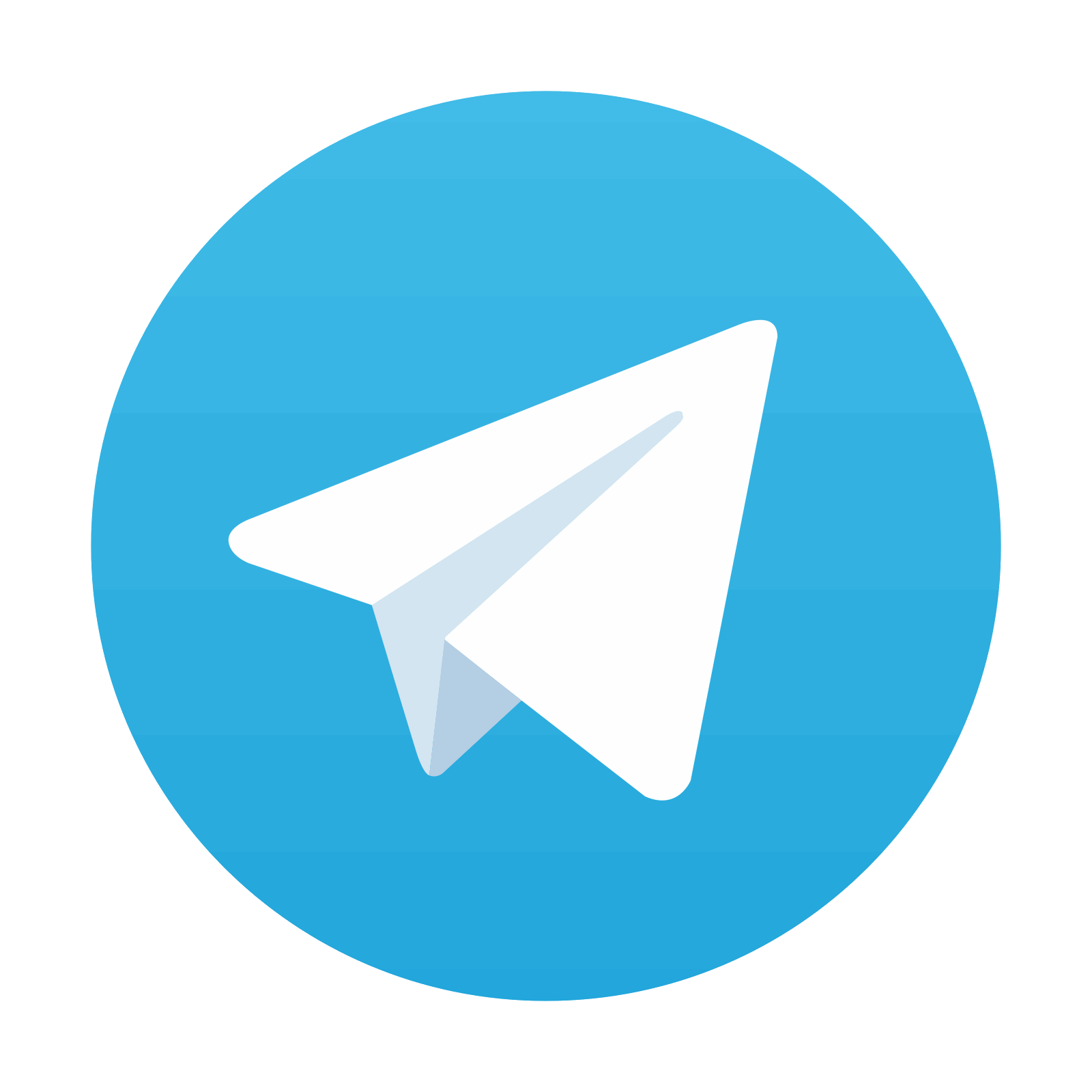
Stay updated, free articles. Join our Telegram channel

Full access? Get Clinical Tree
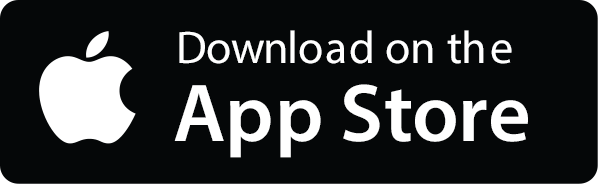
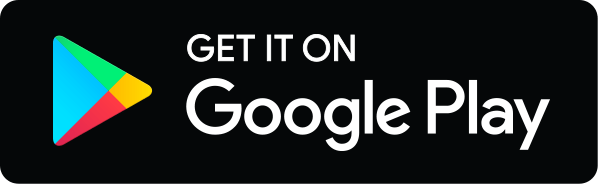