CHAPTER The skin is the largest organ of the human body and through its numerous complex functions provides a protective barrier against the environment. It can protect against harmful chemicals, pathogenic organisms, and ultraviolet radiation while at the same time regulating temperature, maintaining water balance, and producing Vitamin D. A disrupted skin barrier provides a unique challenge to the clinician, because wound healing is a complex and intricate process. Management of wounds places a tremendous burden on patients’ quality of life while also contributing significantly to health care costs. An estimated 6.5 million people in the United States suffer from chronic wounds each year, amounting to an estimated 6 to 15 billion dollars in annual U.S. health care costs.1 With an aging population presenting with various comorbidities (i.e., diabetes mellitus), there has been a striking increase in the number of patients seeking treatment for wound-related problems. Because of these various factors, cutaneous wound healing has become an important topic of ongoing research and debate on an international level. There is tremendous pressure on the medical system to provide and develop cost-effective therapies. Wound healing involves many complicated and delicate interactions to successfully transition from an acute phase to the subsequent proliferation and remodeling phases. When ideal local and systemic conditions are not met, acute wounds have the potential to move from the acute to chronic wounds, requiring the clinician to have a thorough understanding of outside interventions to bring these wounds back into the healing cascade. Optimal management of wounds requires a complete understanding of the wound-healing cascade. Humans, like other mammals, are evolutionarily optimized for expedient healing in suboptimal, dirty conditions. Consequently, the end result of wound healing is scar formation with contraction and replacement of a defect with a collagen-rich matrix, as opposed to regeneration of damaged or lost tissue.2 The process of wound healing has been divided into three phases that may occur in sequence or simultaneously: 1. Inflammation 2. Proliferation 3. Maturation Hemostasis, which has been described as its own phase or as a component of inflammation, occurs initially with local vasoconstriction, activation of the extrinsic coagulation pathway, and formation of a fibrin plug that forms a scaffold for platelet aggregation. Platelets contribute to the subsequent inflammatory phase by producing cytokines (i.e., tumor growth factor-β [TGF-β] and platelet-derived growth factor [PDGF]).3 Infiltration of leukocytes follows, with neutrophils arriving within a few hours and serving both an antimicrobial function as well as a proteolytic function. Within two days, macrophages (attracted by platelets in the fibrin clot), keratinocytes at the wound edge, fibroblasts, and other monocytes arrive and perform most of the débridement of nonviable tissue necessary for the advancement of the healing process. In addition to their phagocytic role, macrophages are essential as antigen-presenting cells and possibly as sources for numerous potent growth factors. Ironically, studies have suggested that neutrophils may not be necessary for proper wound healing, but the absence of macrophages results in significant healing disruption.2 The proliferative phase begins with the arrival of fibroblasts 48 to 76 hours after injury. Within the first week, fibroblasts begin to produce extracellular matrix for granulation tissue formation by depositing type III collagen, and the collagen content of the wound increases until around 6 weeks. During this time, keratinocytes and endothelial cells proliferate, creating granulation tissue and epithelialization on the surface. Fibroblasts migrate along new vessels centrally into the wound, facilitating the complete coverage of the wound bed with granulation tissue. Remodeling and maturation is characterized by reorganization and replacement of the randomly oriented type III collagen typical of granulation tissue with linearly oriented type I collagen associated with scar tissue. This process continues until the ratio of type III to type I collagen is 4:1, equal to that of normal skin. Fibroblasts in the wound differentiate into myofibroblasts, theorized to cause wound contraction, which serves to reduce the size of the defect or wound. During this time, T cells play an important role in overcoming local infections. By 2 years, the process of wound maturation is complete, and the tensile strength of the new scar tissue is around 80% of the preinjury state.3 Understanding the pathophysiology contributing to a specific wound includes the contributing patient factors. Diabetes is one of the most common etiologies of chronic wounds. Long-term hyperglycemia leads to the development of neuropathy, microvascular and macrovascular disease, and capillary and arteriole basement membrane thickening. Patients often repeatedly traumatize insensate areas, and vascular disease contributes to local ischemia. Hyperglycemia further affects the physiology of the wound environment through the formation of advanced glycation end-products (AGEs), changes in cell morphology and function, and alteration of immune function and cytokine expression in the wound environment. Management of hyperglycemia in diabetic patients has been shown to have a significant impact on healing times of leg and foot ulcers.4 Chronic venous insufficiency (CVI) is another common cause of chronic wounds and is therefore important to recognize for its unique implications in wound treatment. CVI may originate from venous reflux or obstruction. Although reflux is the more prevalent cause in CVI-related ulceration, obstruction carries a higher risk of ulcer development and a more rapid disease course. Both mechanisms establish disease by elevating hydrostatic pressure in the venous system, which leads to inflammation and a subsequent increase in ambulatory venous pressure. Altered shear stress and mechanical forces placed on both the microscopic and macroscopic venous systems in CVI induce proinflammatory responses from the endothelium that directly contribute to ulcer development. Inflammation, with the subsequent expression of matrix metalloproteinases (MMPs) and cytokines, damages not only venous structures including the glycocalyx, endothelium, vein wall, and vein valve, but also surrounding tissues like the dermis. Destruction of the dermis eventually leads to skin changes and ulcer formation. A mainstay of CVI-related ulcer treatment is compression therapy. Patients may also benefit from endovascular and surgical management which target both ulcer healing and prevention of ulcer recurrence.5 A mainstay of CVI-related ulcer treatment is compression therapy. Patients may also benefit from endovascular and surgical management which target both ulcer healing and prevention of ulcer recurrence.5 A mainstay of CVI-related ulcer treatment is compression therapy. Patients may also benefit from endovascular and surgical management which target both ulcer healing and prevention of ulcer recurrence.5 A mainstay of CVI-related ulcer treatment is compression therapy. Patients may also benefit from endovascular and surgical management which target both ulcer healing and prevention of ulcer recurrence.5 Summary Box Factors Impeding Wound Healing Endogenous Exogenous • Local tissue hypoxia • Chronic metabolic disorder • Immune system dysfunction • Neoplasm • Colonization with microbes • Persistent necrosis • Trauma • Chemical/physical agents Another important consideration is patients’ nutritional status. Malnourished patients cannot meet the high metabolic demands required for wound healing and have impaired immune function.6 Patient age is another important factor to consider in wound healing; an impaired stress response has been noted with increasing age. Cells in the local wound environment experience a significant stress from inflammation, reactive oxygen species, proteolytic environments secondary to bacteria, ischemia, and ischemia-reperfusion cycles. An adequate stress response is therefore necessary for the cells in the wound environment to respond appropriately. However, elderly patients are known to respond poorly to severe stresses like major burns, and aged cells have been noted to experience broadly altered gene expression that results in impaired response to stresses. Aged cells may more rapidly enter a state of replicative senescence in response to exposure to stress and cytokines. A potential way to address this issue is aggressive débridement, which removes cells in the wound margins that may have lost replicative potential and converts a chronic wound into an acute wound.7 An acute wound is one in which the normal healing process as described previously progresses normally without disruption. These wounds can be from penetrating or blunt trauma, chemical exposure, temperature exposure, envenomation, surgical or iatrogenic causes, and others. The mechanism of injury determines the nature of the damage and thus may affect the necessary interventions. Common to all types of wounds, management involves preparation of the wound for optimal healing and prevention of chronic wound development.8 Chronic wound development is the most prevalent wound-healing problem in humans. Failure to progress through the normal phases of wound healing result in persistent disruption of the skin barrier. These wounds remain in a chronic inflammatory state instead of forming and remodeling new tissue. This deregulated inflammation is promoted by unbalanced proteolytic activity and a highly pro-oxidant environment. Matrix metalloproteinase (MMP) expression from activated keratinocytes at the wound edge, fibroblasts, endothelial cells, and macrophages is induced by proinflammatory cytokines. Not only does excess MMP activity impair the production of new matrix, but it also targets and inactivates growth factors. The highly pro-oxidant environment is a consequence of reactive oxygen species (ROS) formation by leukocytes (especially neutrophils), endothelial cells, and fibroblasts. Although normally functioning to target and destroy pathogens, ROS unchecked in chronic wounds directly damage cell membranes and structural proteins, promoting the expression of proinflammatory cytokines, chemokines, and proteolytic enzymes.2 Both local and systemic factors may contribute to chronic wound development. Infection is the most commonly implicated factor.9 Other local factors include tissue hypoxia and edema, repeated trauma, history of irradiation, and presence of necrotic tissue. Systemic factors include diabetes mellitus, malnutrition, immunodeficiency, and certain medications.9 Local tissue hypoxia is caused by impaired perfusion and edema, which may result from chronic venous insufficiency (CVI), peripheral arterial occlusive diseases (PAOD), microangiopathies, and disturbed lymphatic drainage. The three most common causes of chronic wounds are CVI, diabetes mellitus, and pressure necrosis.2 Chronic wounds increase the risk for neoplastic transformation of local tissues through an unclear mechanism.2 The most common neoplasm presenting in chronic wounds is squamous cell carcinoma, suggesting that keratinocytes are especially vulnerable to malignant transformation.2 These lesions most often present as an enlarging ulceration in a preexisting chronic wound or scar (i.e., Marjolin’s ulcer). A primary tumor, especially in sun-exposed areas, or metastatic tumor can also present as a nonhealing wound. Biopsies of suspicious wounds are warranted. Any patient with a chronic wound requires a thorough history and examination before embarking on a course for therapy. Preexisting concurrent illnesses, such as diabetes, malnutrition, renal failure, and immunosuppression, need to be optimized. In 2004 a framework for creating an optimal wound bed was developed using the current understanding of wound physiology, and the principles were organized into the acronym “TIME.”10,11 (Box 14.1). These guidelines help to direct the removal of exogenous barriers to healing so that an appropriate wound bed may be prepared before wound closure attempts.12 T = Tissue management I = Infection/inflammation control M = Moisture balance E = Edge of wound The category of tissue management focuses on wound débridement. Débridement, introduced by Pierre Joseph Desault in 1790, is the freshening of the edges of a wound by excising all necrotic or nonviable tissue.9 Desault performed débridement with the goal of preparing a wound for primary closure; however, current practice often uses débridement to promote the development of an optimal wound bed for healing by secondary intention or surgical closure at a later stage. Necrotic and devitalized tissues, which slow wound healing and are a medium for bacterial growth, are normally removed during the inflammatory phase of healing. Therefore interventions to facilitate débridement supplement the normal healing response in settings of excessive devitalized tissue burden. In addition to the removal of necrotic tissue, débridement involves the removal of wound coatings and foreign bodies. These devitalized and infected tissues are hypothesized to promote the persistence of the inflammatory phase, which via its progression is necessary to both fight the infection and degrade these tissues. As mentioned previously, infection is the most commonly implicated reason for impaired wound healing. Shortly after creation, every wound becomes colonized with bacteria. Four levels of bacterial burden have been described: contamination, colonization, critical colonization, and infection. Lower levels of bacteria may constitute a “physiological wound flora” that has a positive impact on the wound environment through the presence of bacterial proteases and stimulation of neutrophils. Critical colonization is described at a level in which the physiologic response against the bacteria is overwhelmed before developing into gross infection. The traditionally cited threshold for infection is 1.0 × 105 colony-forming units per gram of tissue (CFU/g). Clinical criteria such as healing grade, exudation, wound odor, and other typical signs of infection have been established to differentiate critical colonization from infection. It has been noted, however, that wounds with bioburden greater than 106 CFU/g may lack clinical signs of infection. Therefore the quantity of bacteria in a wound may be less important than the virulence of the species of bacteria present.13 Absolute bacterial burden is an important negative prognostic predictor of wound healing. It is hypothesized that the “collective pathogenic effect” of the broad bacterial flora or the specific virulence of individual bacteria plays a significant role in disrupting healing. A feature of the bacterial colonization in wounds is the formation of biofilms. These biofilms are characterized by a heterogeneous aggregate of bacteria embedded in a glycocalyx matrix. The biofilms’ structure serves as a barrier to antimicrobial therapies. Consequently, biofilms exert significant negative influence on the wound environment. Management and eradication of biofilms are integral steps toward healing. The use of antibiotics to fight wound bioburden is contraindicated because of the common presence of antibiotic resistance, pathogen selection, the risk for contact dermatitis in topical applications, and a lack of evidence for their benefit in wound healing.10 Antibiotics are required in the case of invasive infection associated with a wound. Generally, this presents as a periwound cellulitis. Preferred antimicrobial agents are further discussed later in this chapter. Clinicians must remember that the presence of microbes in a chronic wound does not necessarily necessitate antibacterial therapy. These therapies should only be employed when the bioburden is suspected to exceed critical colonization and thus create a significant barrier to healing. Maintenance of a moist wound bed is of the utmost importance when promoting optimal wound healing. In 1962 George Winter demonstrated that the rate of epithelialization and healing was two to three times faster in wounds for which moisture was maintained and scab formation was prevented compared with wounds that were allowed to dry and form scabs.14 Since then, wound moisture has been demonstrated to positively impact granulation tissue formation, epithelialization, degree of skin maceration, susceptibility for bacterial colonization, and patient comfort. The proteins and cells of a healthy wound bed require a moist environment to properly perform their functions. As part of the physiological wound response, all wounds exude fluid at some stage during healing. This exudate is pale amber in color and lacks odor and is mostly water with traces of the following: • Electrolytes • Nutrients • Proteins • Inflammatory mediators • Growth factors • Leukocytes The quantity of exudate varies with the nature of the wound, the stage of healing, and presence of complications like infection. The physiological role of exudate is to provide moisture and nutrients, enable diffusion of cytokines and growth factors, promote autolytic débridement, and enable migration of cells.14 Problems arise with unmanageable volumes of exudate or changes in the content of the fluid. Complications related to excess exudate include periwound skin maceration, delayed healing, odor, infection, and a need for increased frequency of dressing changes. Chronic wound exudate, in particular, has been noted to cause contact dermatitis and allergic reactions in periwound skin, reflecting a change from the normal fluid composition.14 Although granulation tissue formation and remodeling provide a collagen matrix to restore tensile strength and vascular supply to the skin, complete surface coverage with epithelium is required to restore the functionality of the skin barrier. Epithelialization occurs from the wound edges and skin appendages as part of the proliferative phase of healing. Consequently, addressing the edge of the wound promotes successful epithelial coverage. Required for successful epithelial migration are a granular tissue base and a moist environment. Because wound secretion decreases with granulation tissue formation, moisture may need to be supplemented with dressings. Often, epithelialization fails to occur in chronic wounds despite adequate moistening. In such cases, biologically active skin replacement therapies can promote epithelial coverage of the wound. The most efficient and effective method of preparing a wound bed for healing is through adequate débridement. Necrotic tissue provides substrate for proliferating bacteria that compete with host cells for nutrients and oxygen necessary for healing. The removal of this tissue, therefore, is essential to promote healing. Several approaches have been advocated to facilitate débridement: surgical, biologic, enzymatic, mechanical, and autolytic (Box 14.2). Surgical débridement is the standard of care for chronic wounds and can, when performed properly, move rapidly and definitively through the TIME sequence.9,11,12 Benefits of surgical débridement include direct removal of all devitalized tissue and reduction of bioburden. The two general types of surgical débridement include classic sharp surgical excision and hydrodissection. Surgical excision is performed by direct removal of necrotic tissue. For almost all wounds, the lighting and anesthesia afforded by an operating room are necessary for adequate débridement. Devitalized tissue is removed until healthy, bleeding tissue is appreciated; depending on the extent of the wound, removal of nonviable fascia, muscle, and bone may be required. In fact, amputation is the ultimate form of surgical débridement, reserved for digits and limbs that are not functionally salvageable. Determining the margin of healthy tissues may prove difficult in the normally less vascular subcutaneous tissues, because the presence of punctuate bleeding may not be a reliable indicator of viability. Débridement should always be followed by thorough irrigation to remove any remaining debris. This method is relatively nonselective for devitalized tissue. Surgical • Classic sharp surgical excision • Hydrodissection • Low-frequency ultrasonic débridement Nonsurgical • Biologic • Enzymatic • Mechanical • Autolytic Tangential hydrosurgery is a recent innovation that provides an alternative to the labor-intensive process of classic surgical excision. A powerful, coherent stream of saline moves parallel to the wound surface within the tip of a handheld device and ablates devitalized tissues in the wound surface. The negative pressure generated by the moving fluid, a consequence of the Venturi effect, then removes these tissues.9 This technology provides great control in removing precise layers of tissue. The surgeon can easily recognize normal tissue when it appears in the field. The hydrojet works best in soft tissue but does not débride through tissues such as fascia or tendons. In addition, it has proved particularly useful in concavities like those in pressure ulcers and joint spaces as well as tight spaces such as breast capsules. Low-frequency ultrasound is an interesting new tool for surgical débridement.15 The tip of the handpiece delivers a high-intensity, low-frequency, ultrasound that is transmitted by water vapor. The energy implodes necrotic tissue, bacteria, and biofilm by means of a bubble cavitation effect. Rapidly oscillating energy waves, 22.5 kilohertz, cause vacuum bubbles to occur in the low-pressure phase. These bubbles then collapse in the high-pressure phase, causing the release of sufficient energy to implode the affected tissue. The end result is a very controlled débridement of all tissue types.16–18 Biologic débridement uses maggots that have been irradiated to prevent metamorphosis into mature flies. Maggots perform débridement by secreting enzymes that selectively digest necrotic tissue. The process is painless for the patient, but sensate patients will feel movement of the larvae. This method is suited for patients who require aggressive débridement but cannot tolerate an operation because of medical comorbidities. However, partly because of psychosocial issues among patients and nursing staff, use of maggots has failed to gain significant popularity in the United States.9,12 Enzymatic débridement uses preparations of proteolytic enzymes that can be applied to a wound to supplement physiological débridement. Enzymes commonly used include papain with urea and collagenase. These enzymes selectively digest devitalized tissue and halt the development of eschar and biofilms, and they are suited to wounds with minimal necrotic loads. These enzymes require a moist environment to properly function and therefore may be combined with dressings that maintain moisture to improve function. Drawbacks to this approach include labor intensiveness, a protracted process, and possibly pain. Bacterial burden must be controlled when using enzyme preparations because proteases released by the bacteria can degrade the enzymes.19 Caution should be exercised, however, when combining antimicrobial dressings with enzymatic débridement, because compatibility between the enzyme and the antimicrobial agent is a concern. For example, silver-containing dressings must be avoided when using papain, because the silver cations inactivate the protease.9 Mechanical débridement is characterized by the physical removal of necrotic tissue. Methods to accomplish this include the following: • Wet-to-dry dressings • Wound irrigation • Hydrotherapy • Negative-pressure wound therapy When using wet-to-dry dressings, necrotic tissue is torn from the wound in a random manner that produces considerable pain for the patient. This method is relatively nonselective for devitalized tissues compared with other options. In addition, wet-to-dry dressings promote evaporative cooling of the wound surface, and wound temperatures around 10°C cooler than normal body temperatures have been reported. This local hypothermia has notable negative effects on wound healing through local vasoconstriction, hypoxia, diminished phagocytosis, impaired leukocyte mobility, and increased affinity of hemoglobin for oxygen. Furthermore, gauze is not a physical obstacle to pathogens, and it must be changed two to three times daily to maintain a moist environment. Autolytic débridement therapies attempt to optimize the wound environment for the body’s native, physiological débridement mechanisms. Débridement, as previously mentioned, is an essential part of the wound-healing process and is normally performed by proteolytic enzymes and macrophages. Dressings that promote autolytic débridement are occlusive or semiocclusive dressings, hydrocolloids, and moisture interactive dressings, which share the feature of maintaining wound moisture. They may be used alone, subsequent to surgical débridement, or in conjunction with other débridement options. This method is nonspecific, uncontrolled, and time and labor intensive. In addition, open wounds with persistent necrotic tissue are at risk for development of invasive infection. Autolytic débridement may therefore benefit patients whose wounds have minimal necrotic load and who cannot tolerate more aggressive débridement. Use of a variety of materials and substances for dressing wounds has been documented for thousands of years.20 Recently, remarkable progress has been made combining biomaterials and material science with knowledge of wound physiology to facilitate the creation of an optimal wound bed. Modern dressings may be impregnated with antimicrobial agents, capable of removing excess exudate, and providing moisture when needed. In addition, dressings are now being developed that can differentially respond to varying wound environments. Choosing an appropriate dressing for a wound ultimately requires considering the dressing types that optimize the TIME characteristics, patient comfort, ease of implementation, and cost (Box 14.3). Various dressings and materials have been developed to target infection and inflammation in the wound bed. A common feature is the limited application of antibiotic medications to the dressings. Antibiotics are typically avoided when treating chronic wounds because of the risk for selection of resistant pathogen strains, as well as sensitization of the patient to the antibiotic agent. Antimicrobials that have demonstrated little to no selection for resistant pathogens are chlorhexidine, honey, octenidine, polyhexanide, povidone iodine, and silver in vitro.13 Ionic silver is a common component of many currently available antimicrobial dressings. Ionic silver acts by binding and disrupting protein disulfide bridges and arresting microbial metabolic processes. Silver has a broad spectrum of activity, covering yeast, viruses, anaerobic and aerobic bacteria, and gram-positive and gram-negative bacteria. A number of dressings impregnated with silver are widely available (Fig. 14.1).
14
Wound Management
Avoiding Unfavorable Results and Complications in Wound Management
Understanding the Wound-Healing Cascade
Patient-Specific Considerations
Chronic venous insufficiency (CVI)
Peripheral arterial occlusive diseases (PAOD)
Microangiopathy
Disturbed lymphatic drainage
Metabolic disorder
Malnutrition
Primary tumors
Metastases
Chemical agents
Drugs
Ionizing radiation
Chronic mechanical forces
Thermal
Acute Wound Versus Chronic Wound
Acute Wound
Chronic Wound
Managing Unfavorable Results and Complications in Wound Management
Evaluation of Wound Care Needs
Tissue Management
Infection and Inflammation Control
Moisture Balance
Edge of Wound
Wound Débridement
Advanced Dressings and Surgical Coverage of Wounds
Advanced Dressings
Materials
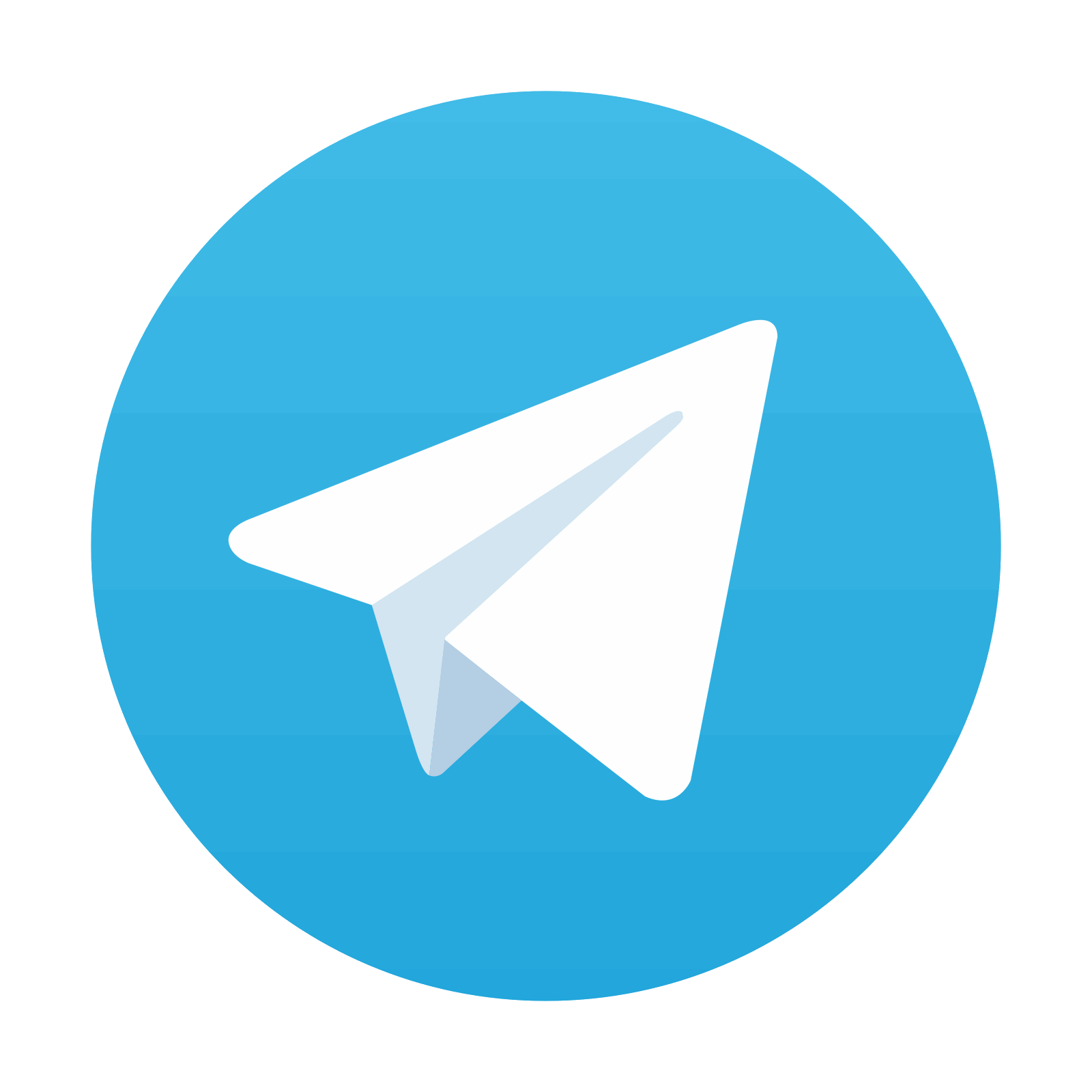
Stay updated, free articles. Join our Telegram channel

Full access? Get Clinical Tree
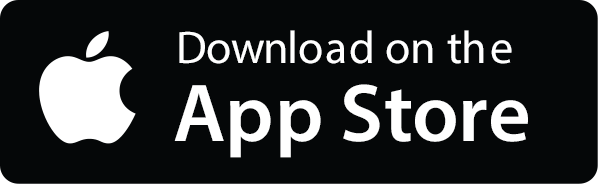
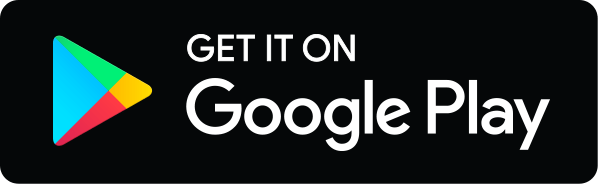