40 Treatment of the upper extremity amputee
Synopsis
Upper extremity amputees face many unique challenges in everyday life.
The surgeon can be an active participant in patient care, both at the time of the amputation and years later to maximize function and use of the residual limb.
Prostheses may be custom-fit to recreate digits and to make amputations less discernible.
New techniques in active prostheses may allow better function of traumatized upper extremities.
Historical perspective
Putti documented the history of upper extremity prostheses in the 1930s, and this work has subsequently been translated into English.1 The field of prosthetics began soon after advancements in battlefield surgery allowed patients to survive limb amputations. Prior to cautery, surgeons were forced to allow tissue to mummify, and then to separate living tissue slowly from dead. Residual limbs developed in this manner had poor soft-tissue coverage of bone, and therefore were poorly able to tolerate the weight of a prosthesis. Advances in metal-working permitted the creation of metallic prostheses beginning in the early 1500s. A famous knight, Gottfried von Berlichingen (c. 1480–1562) was known as Gotz of the Iron Hand for his below-elbow amputation. The prosthesis he wore was strapped to the forearm with leather bands, and had movable fingers and a thumb that could be revolved and tightened around the hilt of a sword. Ambroise Paré documented the ingenious Petit Lorrain artificial hand that had fingers that moved into extension with springs, and could be held in flexion by sprockets that caught metal levers.2 The Alt-Ruppin prosthesis, fished out of a canal in the town of the same name, was constructed in the 1500s and similarly had movable fingers and a thumb. These prostheses inspired the imagination of onlookers, and demonstrated the ingenuity of armor-makers of the day.
The Ballif arm of 1812 was in improvement over the metallic arms for its softer materials and for the active control of the prosthetic hand. Rather than locking prosthetic fingers into place for a static grip, the Ballif arm utilized cables attached to the shoulder and arm to allow opening and closing of the prosthetic hand. The Civil War created a huge number of amputees in the US, leading to companies such as the AA Marks Company (1853) of New York and the JE Hanger Company (1861, still in existence today). These firms and others provided body-powered prostheses to the masses by mail order. World War II led to further refinements in body-powered prostheses with the large numbers of upper extremity amputees, coupled with significant advances in technology developed for the aviation industry.3 With minor adjustments for materials and socket designs, body-powered upper extremity prostheses have remained relatively unchanged since the 1950s. Body-powered prostheses are operated by using shoulder motion to pull on a housed cable (like a bicycle brake cable) and thus transfer power to the prosthetic joints. Generally, only one joint can be operated at a time. Thus, when using a prosthesis with multiple joints, the user unlocks only the joint he or she wishes to operate using a variety of different types of switch.
The development of externally powered prostheses powered by electricity or pressurized gas began in Germany soon after World War I. The myoelectric hand, controlled using electromyogram (EMG) signals from the residual limb, was first created by Mr. Reinhold Reiter for the Bavarian Red Cross in 1943 during World War II and published in 1948.3 Parallel efforts to create a myoelectric prosthesis began in England, the Soviet Union, and the US. The US effort was led by the Committee on Prosthetics Research and Development of the National Research Council, with the initial meeting held at Northwestern University. Technical advancements were greatly aided by the miniaturization of electric components. The first device to use transistors was the “Russian hand” of 1959. Along with the Vaduz and Bottomley hands, these single-function (open-and-close) devices received much publicity, but were rather slow and clumsy to operate. Multifunction myoelectric devices with wrist flexion/extension and pronation/supination were introduced in the mid-1960s as a means of further improving on these devices.4 Despite research efforts that spanned the globe, these multifunction prostheses were limited by the difficulty in the amputee of controlling the movements of the prosthesis.
Proponents of body-powered prostheses have debated with the proponents of externally powered system for the last half-century. However, both types of device are useful and often complementary. Body-powered prostheses are generally lighter and more robust than external systems with batteries and motors. Externally powered systems require more daily maintenance for battery changes and are more fragile than the simpler and sturdier harnesses and hooks. Functionally, body-powered prostheses tend to give the wearer a bit more feedback during use. A significant advantage for transradial and more distal myoelectric prostheses is that they can be anatomically suspended using the humeral condyles or using suction sleeves; this eliminates the need for cumbersome shoulder harnessing. Furthermore, as unilateral upper limb amputees tend to use the prosthetic limb only as a helper hand, the improved cosmetics of myoelectric devices can make up for their many drawbacks and are often preferred.3 Hybrid systems also exist that try to combine the best features of both body-powered and external-powered prostheses. One example is to have a body-powered prosthetic elbow, coupled with a myoelectric hand.
Aesthetic prostheses
Aesthetic prostheses refer to a device used to camouflage a limb deformity, while functional prostheses refer to devices that are operational.5 Aesthetic prostheses assist patients in issues of body image and self-esteem, with possible functional gains as well. These secondary functional gains are a due to a newfound social openness and ability to expose the limb, rather than to a subconscious desire to avoid detection of the limb deformity. Patients can appear fairly normal in public with aesthetic prostheses (Figs 40.1 and 40.2). Most of these prostheses are custom-made and require a high level of expertise on the part of the prosthetist. Because intact skin is used to secure the prosthetics in place, some amount of sensory feedback of the residual limb will be lost, and this is one drawback to these devices. Osseointegration of aesthetic prostheses into bone has been studied at length because it will improve the tolerance of the soft tissues to the prosthesis, limit the amount of soft tissues covered, and permit the use of stiffer materials in the devices for possible secondary functional gains. The bone–soft-tissue–implant interface for osseointegration of upper limb prosthetics remains problematic.6
Control of upper extremity prosthetic devices
A prosthesis is only as good as the control signals that it receives from the user. The goal of any prosthesis is to be moved and positioned in space smoothly, quickly, intuitively, and with minimal exertion and mental fatigue to accomplish this task.7 Multifunctional prostheses create new unique problems for control, because signaling will need to be directed specifically between one function and another. For example, prostheses with both a terminal device and a prosthetic elbow will need to switch from “hand” to “elbow” and then back to “hand” again to accomplish almost every task. This will cause the device to appear jerky and slow in its use.
Kuiken et al. in 1995 demonstrated a new strategy, now called “targeted muscle reinnervation” (TMR), for control of myoelectric prostheses. Rather than using the “wrong” signals from nearby and functionless muscles, nerve transfers were performed to amplify the nerve signal that had previously controlled the limb. After successful neurotization, the muscle would serve to amplify the signal of the amputated nerve, and this EMG signal would be detected transcutaneously.8 The downside of this approach is that the amputee would need a surgical procedure to perform the nerve transfer, and that the intact EMG signal from that muscle would be lost. Kuiken et al. reported this procedure in humans in shoulder disarticulation patients in 2004,9 and O’Shaughnessy et al. reported the same procedure in transhumeral amputees in 2008.10 Continuing the example of a transhumeral amputee with a myoelectric prosthesis, the median nerve could be transferred to the motor nerve of one segment of the biceps muscle and the distal radial nerve could be transferred to the motor nerve of one segment of the triceps muscle. Segments of the biceps and triceps would be left intact during the surgical dissection to maintain the native innervation from the musculocutaneous nerve and the proximal radial nerve, respectively. Therefore, TMR preserves two control areas for prosthetic elbow function, and creates two new control signals for prosthetic hand function. Targeted reinnervation allows for simultaneous movement of both prosthetic joints. The control paradigm is intuitive, because nerves that previously had controlled native joints have regained their function to control the prosthetic joint. The musculocutaneous nerve activates one head of the biceps to cause prosthetic elbow flexion. One intact branch of the proximal radial nerve serves to extend the prosthetic elbow. After neurotization, the median nerve once again causes the terminal device to close with its signal to a segment of the biceps, and the distal radial nerve causes the terminal device to open after causing a segment of the triceps to contract. TMR has been successfully performed clinically in over 40 patients with proximal arm amputations with very high success rates.
Other strategies for prosthetic control are still in the investigative phase, but show promise. Implantable myoelectric sensor systems that transmit EMG signals out to a detecting device could improve the EMG signal quality and consistency, as well as provide control signals from small muscle bodies such as the individual finger extensors of the forearm.11 Advanced computer programs are being developed to decode EMG signals and enable intuitive control of multiple functions. Direct signal acquisition from the amputated nerves using a variety of implanted electrodes has been investigated.12 Prosthetic control using fine wire electrodes was recently demonstrated in an acute experiment in humans.13 Direct recording and stimulation of the cerebral cortex have been performed in monkeys in order to effect peripheral movement. This brain–machine interface, if successful, could have wide applicability not only for amputees, but also for patients with spinal cord injuries.14
Prosthetic implications of upper limb amputation surgery
Finger amputation
Patients undergoing reconstructive upper extremity surgery do best when the surgeon “think[s] nerve.”15 Patients can easily exclude a painless stiff digit, whereas they are greatly troubled by a mobile painful digit with a symptomatic neuroma. Patients with wounds of the fingers should be evaluated as to which nerves are irreparably cut, and which digital nerves are intact. If a major portion of one of the two digital nerves is intact, then a flap should be performed for wound closure, rather than a shortening amputation with division of both digital nerves. The digital nerves to the finger pulp trifurcate approximately 2 mm distal to the volar distal interphalangeal skin crease. A branch travels to the nail fold, one branch travels to the volar tip, and the third branch innervates the volar pad. Amputations at or proximal to the trifurcation have two cut digital nerves, and therefore a revision amputation will not cut a new nerve to achieve wound closure. In Figures 40.3 and 40.4, the ulnar digital nerve to the ring finger is intact, while the radial digital nerve is cut. The cross-finger flap is performed to achieve wound closure, but more importantly, to avoid the necessity of dividing an intact digital nerve in a revision amputation.
Partial hand
Again, “think[ing] nerve,” loss of the ulnar, radial, or dorsal aspect of the hand with some intact volar nerves should undergo flap reconstruction to obviate the need to divide nerves that are in continuity. Typically, this will be either a free flap, or a pedicled groin flap as a second choice. If the majority of the digital nerves are divided, then the next decision is if there is a functional and stable wrist joint. Maintenance of the wrist will allow the residual limb to be an excellent helper hand without a prosthesis (Figs 40.5–40.7). In some avulsive injuries, the dorsal wrist extensors have been removed by the injury, and it is worthwhile reinserting extensor tendons to the residual carpal bones with bone anchors and a flap for coverage. Muscle flaps are preferable to skin flaps, as the associated eventual atrophy will facilitate the placement of passive finger prostheses that can assist in grasp.16
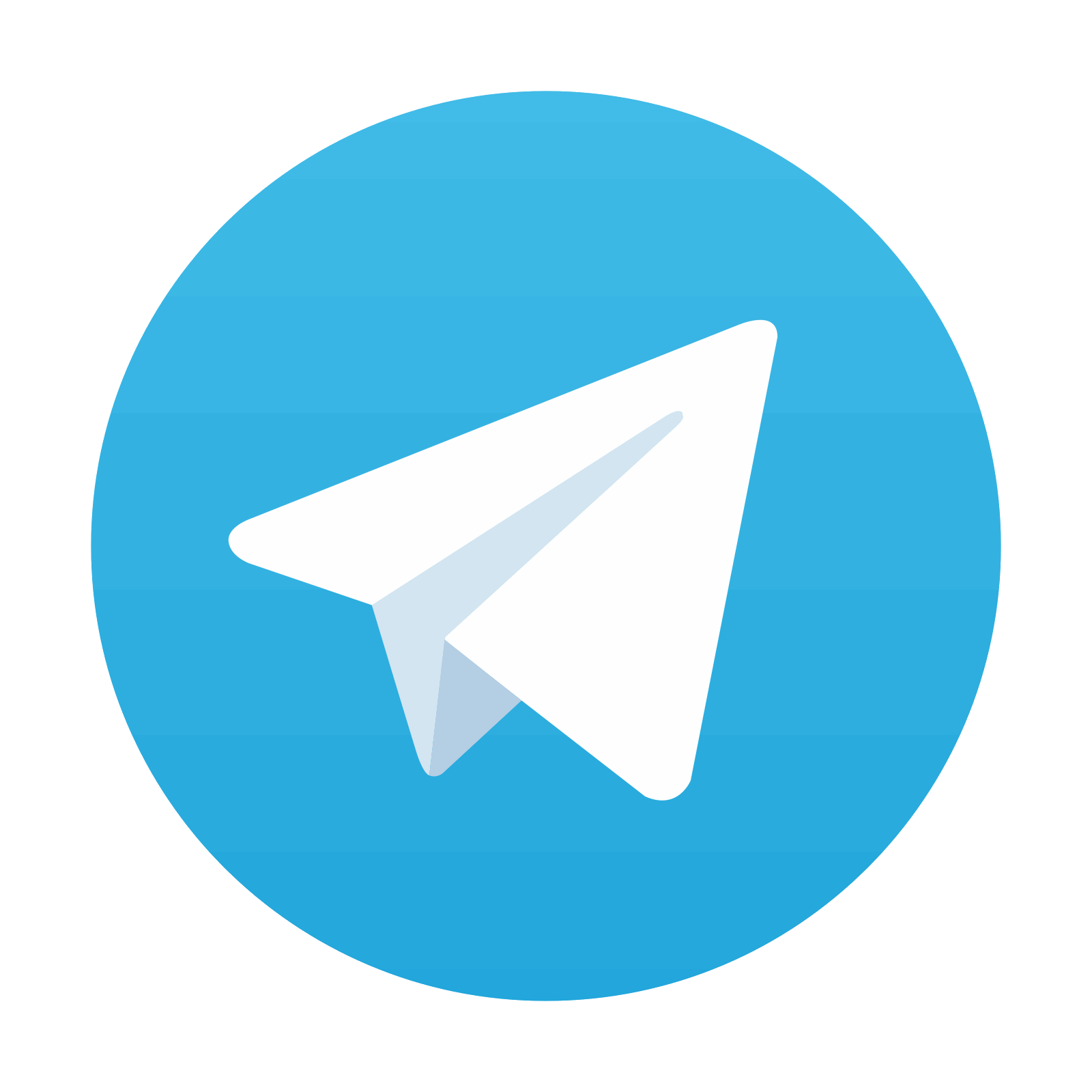
Stay updated, free articles. Join our Telegram channel

Full access? Get Clinical Tree
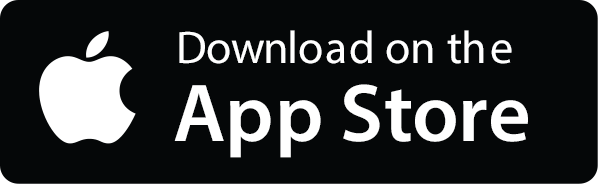
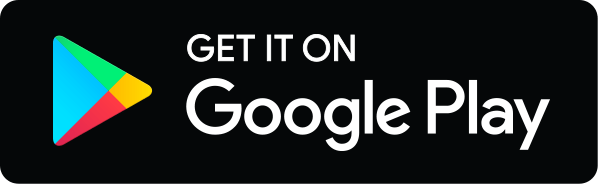