Total hip arthroplasty (THA) is the standard of care for symptomatic degenerative joint disease (DJD) of the hip that is unresponsive to nonoperative treatment.
Cementless THA has demonstrated excellent mid- to long-term results.
The acetabular component obtains initial fixation through a press-fit and has a surface that allows for in- or ongrowth of bone.
The femoral component obtains initial fixation through a press-fit in either the metaphysis or diaphysis and has a surface that allows for in- or ongrowth of bone. The metaphyseal-fit prosthesis may be either wedge-shaped or fit-and-fill.
ANATOMY
The acetabulum must be exposed so that the anterior and posterior walls, superior dome and rim, and teardrop are visualized.
The proximal femur must be exposed so that the periphery of the proximal femoral neck cut is visualized.
PATHOGENESIS
DJD of the hip is the end point of many hip disorders, including osteoarthritis, inflammatory arthritis, dysplasia, osteonecrosis, trauma, and sepsis.
NATURAL HISTORY
DJD of the hip often follows a variable symptomatic course. It is unknown why it progresses more rapidly in some patients than others and why some patients are more symptomatic than others.
PATIENT HISTORY AND PHYSICAL FINDINGS
The history should be directed to determine whether the patient’s pain is extrinsic or intrinsic.
The patient’s pain may be extrinsic (eg, lumbar radiculopathy or intrapelvic pathology) and THA may fail to relieve the patient’s pain completely, even in the face of severe degenerative changes of the hip.
Pain usually is located in the groin but may be located in the medial thigh, buttock, or the medial knee.
Range of motion (ROM) should be observed. Normal ROM of the hip is an arc of motion of 120 to 140 degrees of flexion–extension, 60 to 80 degrees of abduction–adduction, and external–internal rotation of 60 to 90 degrees. Loss of motion may be due to pain, contracture, or abnormal biomechanics.
Nonoperative treatment must be optimized before consideration is given to surgery.
Leg lengths should be measured and recorded preoperatively and the patient should be counseled about reasonable postoperative expectations.
Examinations to perform include the following:
Trendelenburg test. The test is positive if the contralateral hip drops inferiorly; this may indicate that the hip abductors are compromised.
Hip flexion–internal rotation. The test is positive if the patient’s pain is recreated. Pain that is not recreated with this examination may be from an extrinsic source.
IMAGING AND OTHER DIAGNOSTIC STUDIES
Plain radiographs, including anteroposterior (AP) views of the pelvis and AP and true lateral views of the hip, should be obtained to evaluate the anatomy, assess for deformity, and devise an adequate plan preoperatively (FIG 1).
DIFFERENTIAL DIAGNOSIS
Lumbar radiculopathy
Spinal stenosis
Sacroiliac DJD
Intra-abdominal pathology
Intrapelvic pathology
Neuropathy
Meralgia paresthetica
Complex regional pain syndrome
Vascular claudication
Primary bone tumors
Metastasis
Infection
NONOPERATIVE MANAGEMENT
Acetaminophen
Nonsteroidal anti-inflammatory drugs
Glucosamine
Chondroitin sulfate
Physical therapy
SURGICAL MANAGEMENT
The primary indication for cementless THA is painful, severe DJD of the hip that has been nonresponsive to appropriate nonoperative treatment modalities.
Preoperative Planning
Preoperative planning for routine cementless primary THA can be accomplished with plain radiographs at standard magnifications.
Standard templates are available for the components and many are available for digital templating.
The acetabular component is placed so that the inferomedial edge of the cup is at the radiographic teardrop. The inclination is 35 to 45 degrees and the cup should contact the superolateral rim of the acetabulum.
The femoral component is placed so that the center of rotation is at the level of the greater trochanter. The femoral offset should be reproduced. However, the femur must be internally rotated 10 to 15 degrees on the radiograph to bring the femoral neck into the plane of the radiograph. Externally rotated femora will appear to be in coxa valga.
Proximal-fit femoral prostheses are designed to obtain fit in the metadiaphyseal region.
Diaphyseal-fit femoral prostheses are designed to obtain fit in the diaphysis.
Modular Femoral Stems
Despite good results with fully monoblock stems introduced in the 1960s, surgeons had difficulty fine-tuning leg length and soft tissue tensioning during THA. Meticulous attention to preoperative planning, neck resection levels, and cementation technique along with adjustment of soft tissue tension via repair of trochanteric osteotomy were used to restore appropriate hip biomechanics.
The primary benefit of modularity is the relative ease with which surgeons can optimize hip biomechanics, and it is for this reason that the vast majority of surgeons today use femoral implants with at least one modular junction.
Other benefits include the following:
Aids in exposure at the time of revision by removing the modular parts and retaining the osseointegrated portion of the implant
Alterations to modular parts can be easily adjusted at revision, especially in the presence of instability. This has led to clinically apparent reductions in the dislocation rates in hip revisions.8,32
Replacement of worn or damaged parts without requiring removal of the osseointegrated portion of the implant
Femoral Modularity: The Basics
Femoral modularity can exist at one or more junctions.
Head–neck: Nearly all modern femoral stems have modularity at this junction. It allows adjustment of head size and neck length.
Metaphyseal: Metadiaphyseal modular junction of the femur, distal to the femoral neck osteotomy (S-ROM [DePuy, Warsaw, IN], Emperion [Smith & Nephew, Memphis, TN], Restoration [Stryker Orthopaedics, Kalamazoo, MI]). This allows for multiple neck options for offset, version, neck–shaft angle, and neck length in addition to femoral head–neck options.
Dual neck: Double modularity of the neck with a head–neck junction and a junction proximal to the femoral neck osteotomy, unsupported by host bone (ProFemur [Wright Medical Technology, Arlington, TN]; M-L Taper with Kinectiv Technology [Zimmer, Warsaw, IN]; Anatomic Benoist Girard [ABG] [Stryker Orthopaedics], Rejuvenate [Stryker Orthopaedics]). These stems also allow for multiple neck options for offset, version, neck–shaft angle, and neck length in addition to femoral head–neck options. However, the latter two stems have been recalled.
Modularity of femoral stems occurs at a Morse style tapered junction. The trunnion compresses into the bore as it expands, creating an interference fit. In this way, it provides both axial and rotational stability.
Tapers exist in proprietary geometries, depending on the component design. It is important to know the taper type, particularly in revision situations, in order to have the proper instruments and implants available.
Potential Advantages of Modular Stems
Biomechanical goals of THA
Restore the normal hip center.
Recreate the bony anatomy.
Optimize soft tissue tension.
The use of modular femoral components may allow for optimal restoration of hip biomechanics.
Most nonmodular (monolithic) femoral stems are based on a range of normal femoral morphology. This may not allow for accurate restoration of individual biomechanics.
Noble et al39 analyzed 200 cadaveric femurs and found that there is no specific relationship between the size and shape of the metaphysis and diaphysis.
Anthropologic literature has demonstrated the effect of aging on femoral morphology. Endosteal changes occur primarily in the diaphysis, with cortical thinning and medullary expansion beginning in the fourth decade. A practical consequence of the effect of age on the size of the endosteal surface is the possible need for femoral components of varying neck length and offset for a given canal size.
Adequate soft tissue tension is created through the offset and neck length. Insufficient soft tissue tension may lead to a decreased abductor lever arm and weakness and/or instability. Excessive soft tissue tension may result in pelvic tilt, leg length inequality, and/or greater trochanteric pain syndrome.
Certain anatomic variants such as the increased femoral neck anteversion seen in developmental dysplasia of the hip, retroversion in slipped capital femoral epiphysis, coxa vara, and malunions from previous fractures can increase the difficulty in restoring proper hip biomechanics. In a study of 1000 primary THAs using the S-ROM prosthesis, Kindsfater et al29 found that the anteversion needed adjustment to enhance stability in over 47% of the cases.
Modular systems can provide up to 10,398 combinations of sizes and shapes. This enables the surgeon to adjust the prosthesis to the patient and not the patient to the prosthesis.5
Therefore, the establishment of immediate rigidity, including resistance to torsional forces, is imperative for the long-term survivorship of the prosthesis.
Ohl et al40 and Otani and Whiteside41 determined biomechanically that both proximal and distal fixation of the prosthesis resisted torsional loads better than either proximal fixation or distal fixation.
The difficulty with a nonmodular stem is that the proximal metaphyseal and proximal diaphyseal areas of human femur tend not to correlate in terms of size and geometry.33,39
Midstem modular prostheses and some proximal stem systems (S-ROM) allow the surgeon to achieve a tight fit both proximally and distally. The surgeon can independently size components that tightly fit in both anatomic areas.
Concerns
The major concern with modular femoral components is the addition of another junction. The major problems associated with these additional articulations are as follows:
Fretting/corrosion
Fracture
Dissociation
More recent concerns include the generation of metal debris and soft tissue reaction to the debris.
Fretting and corrosion are often linked, synergistic processes that can lead to poor outcomes for two reasons26:
The degradation process can lead to a decrease in the structural integrity of the metal.
The release of degradation products may elicit an adverse biologic reaction in the host.
Fretting
Results from micromotion between two surfaces. The movement required for disruption of the passive oxidation layer on metal can be as little as 3 to 4 nm and depends on the frequency of motion and the amount of load on the construct.
Motion leading to reduction of the oxide layer on the surface can expose the base alloy to an aqueous solution.
Exposure to the aqueous solution oxidizes the alloy into ionic form (dissolution) or reacts with oxygen to reform the oxide film (repassivation).
When this repassivation occurs in a crevice, such as that associated with a modular neck–stem interface, it depletes the local oxygen supply, creating a more acidic environment. This situation creates accelerated corrosion, pitting, and cracks.
Metals that are repeatedly stressed in a corrosive environment can experience catastrophic failure.
Fretting at the neck–stem junction in proximal modular stems occurs more frequently and to a greater extent than that of the head–neck taper.
• A retrieval of 16 dual modular stem were analyzed, 6 of which demonstrated significant fretting and crevice corrosion at the neck–stem junction. This contrasted with only 3 of the stems having any sign of corrosion at the head–neck junction.30
• Another retrieval analysis of a proximal dual modular stem found evidence of abrasion and corrosion, indicating that initiation and propagation of the fatigue crack could have been corrosion assisted. The neck in question was the largest size neck available for this implant.13
Femoral stem fractures are rare events. However, there have been several case reports of proximal modular stem fractures.3,13,22,49
Huot Carlson et al25 examined 78 retrieved modular stems and found 7 with fractures. They correlated the presence of stem fracture with the combination of small stem; large femoral neck offset; and active, heavy patients.
Atwood et al3 analyzed a retrieved proximal modular femoral stem (ProFemur Z) that experienced catastrophic failure at the modular neck less than 2 years after implantation. Analysis of the fractured stem revealed large (200 μm) crevices and areas of burnishing, which indicated that fretting occurred. There was also an absence of evidence to support simple cyclic fatigue failure.
Skendzel et al49 reported two dual modular stem failures. Both occurred in men weighing over 220 pounds whose index procedure was less than 4 years before the time of failure.
Dissociation of the modular components is another concern.
The majority of reported cases are those of femoral head–neck dissociation. These usually occur after a hip dislocation and the subsequent reduction attempt.9,47,56
Others have reported dissociation at other levels of modularity.1,50,51 Microscopic examination of the retrieved prostheses have also shown the presence of fretting at the neck–stem interface, suggesting that there may be micromotion present, leading to corrosion, dissolution of the metal, and eventual dissociation of the taper.50
Adverse reaction to metal debris (ARMD)
Collier examined the extent of corrosion at modular junctions in two studies. In one study, they found corrosion present in more than 30% of retrievals when mixed alloy head–stem combinations were used. When all cobalt–chrome and all-titanium combinations were used, corrosion was present in less than 6% and 10%, respectively.10,11
It is now well established that metallic particulate debris can initiate ARMD, a cell-mediated hypersensitivity reaction.23 ARMD may lead to destructive lesions. This was recently reported initially with metal-on-metal articulations in resurfacings and THA, but concern has been growing regarding the junctions associated with modular hip stems.19
There have been increasing reports of adverse soft tissue reactions (pseudotumor) in THAs with a metal-polyethylene articulation. Crevice corrosion at the head–neck taper (trunnionosis) is thought to be the source.12,34,35,37,52 Others have reported the presence of a pseudotumor in dual modular stems where retrieval analysis shows large degrees of fretting and corrosion at the neck–stem interface but pristine trunnions at the head–neck junction.55
The material makeup of the head–neck junction has a large effect on the degree of corrosion seen in retrieval studies. There is a higher incidence of corrosion in the presence of mixed alloy combinations. This is due to the mechanical and tribologic properties of the metals.18 When two dissimilar metals are in contact in an electrolyte solution, there is an electrical potential difference between them, resulting in a flow of electrons creating galvanic corrosion.
Ceramic heads appear to reduce the amount of taper corrosion, but they do not eliminate it. Taper corrosion occurs with a similar frequency with ceramic heads on metal tapers, but not to the same extent. The mechanism of the development of corrosion is similar, but in the presence of a ceramic head, it only occurs on the metal taper.31
Conclusions
Modularity may have a role in THA in select cases.
Such cases may include deformity, abnormal femoral morphology, or inability to achieve stability with nonmodular implants.
Risks of modularity include ARMD, failure of the modular junction, and/or subsequent need for reoperation.
The two materials used at the modular junction may be important in terms of adverse reactions.
Therefore, modularity should be used selectively and after careful consideration.
Positioning
The patient is positioned according to surgeon preference and in accordance with the surgical approach.
The hip should be draped to allow a wide surgical exposure should an extensile approach be required in the event of a complication.
The pelvis must be stabilized in a secure fashion to avoid pelvic tilt, which may affect the surgeon’s perception of the acetabular position.
Approach
The hip can be exposed for primary routine arthroplasty via a variety of approaches:
Anterior
Anterolateral
Direct lateral
Posterior
Two-incision
Small-incision variants of these approaches
TECHNIQUES
Acetabular Exposure
The approach to the hip is chosen according to the surgeon’s preference. The approach illustrated here is the direct lateral (modified Hardinge) approach in the supine position.
Retractors are placed in the anterior, superior, and inferior positions, thereby exposing the entire periphery of the acetabulum (TECH FIG 1).
The labrum is resected.
The soft tissue in the cotyloid fossa is removed, allowing exposure of the medial wall and teardrop.
Acetabular Preparation
Before reaming, the entire periphery of the acetabulum, medial wall, and teardrop must be directly visualized (TECH FIG 2).
The initial reaming must be done with moderate pressure until the quality of bone is assessed.
The goal of the initial reaming is to medialize the reamer fully. The cotyloid fossa should be eliminated without penetrating the medial wall.
Reaming then proceeds sequentially. The goal is to recreate the center of rotation by placing the inferomedial aspect of the socket at the level of the teardrop with the component inclined at 35 to 45 degrees, with 10 to 20 degrees of anteversion and good initial fixation obtained through a press-fit.
The templated size should be used as a guide; intraoperatively, an increase or decrease in cup diameter may be found to be appropriate.
Failure to recognize the need for a different cup diameter may lead to iatrogenic fracture or a failure to achieve initial fixation.
The bony bed should be bleeding but not devoid of sclerotic bone.
The pelvis must remain in a stable position to avoid malpositioning of the acetabular component.
Acetabular Component Implantation
The position of the pelvis is reassessed. Any tilt is corrected.
The trial component or reamer is used to assess bone coverage of the component and position (TECH FIG 3). If the trial or reamer is not seated properly, then further reaming may be necessary. If deemed appropriate, implantation of the actual component may proceed.
The actual implant should be 1 to 2 mm larger than the last reamer. The surgeon must know the actual diameter of the implant, taking into account any rim or coating.
Implants that are larger than the size of the last reamer by 4 mm or more are associated with risk of fracture.
The acetabular component is then implanted, with care taken to medialize the implant. The inferomedial aspect of the cup should be at the level of the teardrop in 35 to 45 degrees of abduction and 10 to 20 degrees of anteversion.
A trial liner or the actual liner is then inserted.
Femoral Exposure
The femur is exposed by elevating it out of the wound with a retractor (Bennett or double-footed).
The periphery of the femur is exposed with another retractor (TECH FIG 4).
The soft tissues must be protected so that iatrogenic damage by reamers or broaches is avoided.
Femoral Preparation (Proximal-Fit Prosthesis)
The femur is prepared as delineated by the surgical protocol for each prosthesis (TECH FIG 5). The surgeon should be familiar with the prosthesis and all of the available options and idiosyncrasies.
The proximal-fit prosthesis usually requires a starter reamer, which is used as a canal finder. In addition, the reamer should be lateralized to avoid broaching and subsequent varus positioning of the implant.
The femur then is broached sequentially, with care taken to lateralize the broach. Broaching is complete when the broach ceases to advance, the pitch of impaction increases, and good cortical contact is obtained.
Improper broaching can lead to fracture, malposition, or undersizing.
The component should be anteverted 10 to 15 degrees.
The greater trochanter can be used as a reference to recreate the center of rotation.
It may be necessary to adjust the neck cut to allow for further seating of the prosthesis.
The templated size should be used as a guide and an increase or decrease in stem size intraoperatively may be appropriate.
Failure to recognize the need for a different size may lead to iatrogenic fracture or failure to achieve initial fixation.
A standard or varus neck is selected based on the soft tissue tension and the patient’s anatomy.
Femoral Component Implantation (Proximal-Fit Prosthesis)
The implant chosen usually matches the size of the last broach.
The proximal-fit femoral component is inserted, with care taken to avoid varus positioning (TECH FIG 6).
The implant is impacted until it ceases to advance, the pitch of impaction increases, there is good cortical contact, and the implant has reached the level of the last broach.
Soft Tissue Tension/Leg Length Determination
The hip is trial reduced and assessed for soft tissue tension, stability, ROM, impingement, and leg length.
The soft tissue tension should allow for no more than 1 to 2 mm of toggle (TECH FIG 7).
The hip should be stable within the patient’s physiologic ROM. If instability exists, the position of the components must be reassessed.
The ROM should be physiologic for the patient.
Impingement must be assessed and rectified with removal of any remaining osteophytes.
Increased offset may aid in decreasing impingement.
Proper component position must be verified to exclude positioning as a source of impingement.
The patient’s leg length is assessed either directly through palpation of the heels or malleoli or indirectly through the use of a pin placed in the ilium and a marker placed on the femur.
One must be careful to position the limb accurately to avoid inducing error during the measurement process.
Closure
The wound is thoroughly irrigated.
Drains are placed at the discretion of the surgeon.
The capsule is closed meticulously, especially if a posterior approach is used.
The soft tissues are closed with absorbable suture.
The skin is closed according to surgeon preference.
PEARLS AND PITFALLS | |
Preoperative planning |
|
Intraoperative decision making |
|
Implantation |
|
Soft tissues |
|
POSTOPERATIVE CARE
Weight bearing after cementless THA is controversial. Some surgeons routinely restrict weight bearing for 6 weeks, whereas others allow weight bearing as tolerated.
Hip precautions are prescribed according to the approach.
Posterior approaches avoid flexion, internal rotation, and adduction, whereas anterior approaches avoid extension, external rotation, and adduction.
The hip precautions are discontinued at 6 weeks.
Some surgeons who perform the anterior approach have discontinued the use of traditional hip precautions.46
The patient ambulates with the aid of crutches or a walker for several weeks and progresses to use of a cane. Generally, the cane is discontinued at 6 weeks.
OUTCOMES
The survivorship of cementless THA components generally has been excellent, although isolated reports of high failure rates exist for certain designs, which have subsequently been abandoned. Most modern, uncemented acetabular and femoral components have a reported survivorship of 95% to 100% at mid- to long-term follow-up.2,4,6,7,14–17,20,21,24,27,28,36,38,42–45,48,53
Overall survival of cementless acetabular components has ranged from 83% to 99.1% at 8.5 to 16.3 years of follow-up.2,6,14,16,21,24,28,38,48,54
The reported survivorship of cementless femoral components has ranged from 82% to 100% at 6.6 to 17.5 years of follow-up.2,4,15,17,20,27,28,36,42–45,53
The main limitation to long-term clinical success has been wear and subsequent osteolysis.
COMPLICATIONS
Iatrogenic fracture
Stress shielding of proximal bone
Blood loss
Infection
Neurovascular injury
Anesthetic and medical complications
Loosening
Osteolysis
REFERENCE
1. Abdel-Aal AM. Dissociation of modular total hip arthroplasty at different levels due to subsidence of cementless stems. A report of three cases. Orthopedics 2008;31:82.
2. Archibeck MJ, Berger RA, Jacobs JJ, et al. Second-generation cementless total hip arthroplasty: eight to eleven-year results. J Bone Joint Surg Am 2001;83:1666–1673.
3. Atwood SA, Patten EW, Bozic KJ, et al. Corrosion-induced fracture of a double-modular hip prosthesis: a case report. J Bone Joint Surg Am 2010;92(6):1522–1525.
4. Bojescul JA, Xenos JS, Callaghan JJ, et al. Results of porous-coated anatomic total hip arthroplasty without cement at fifteen years: a concise follow-up of a previous report. J Bone Joint Surg Am 2003;85: 1079–1083.
5. Buly R. The S-ROM stem: versatility of stem/sleeve combinations and head options. Orthopedics 2005;28(9 suppl):S1025–S1032.
6. Callaghan JJ, Savory CG, O’Rourke MR, et al. Are all cementless acetabular components created equal? J Arthroplasty 2004;19(4 suppl 1):95–98.
7. Capello WN, D’Antonio JA, Feinberg JR, et al. Ten-year results with hydroxyapatite-coated total hip femoral components in patients less than fifty years old: a concise follow-up of a previous report. J Bone Joint Surg Am 2003;85:885–889.
8. Carter A, Mortazavi SMJ, Sheehan E, et al. Revision for instability: what are the predictors of failure? In: 78th Annual Meeting Proceedings. Rosemont, IL: American Academy of Orthopedic Surgeons, 2011:500–501.
9. Chu CM, Wang SJ, Lin LC. Dissociation of modular total hip arthroplasty at the femoral head-neck interface after loosening of the acetabular shell following hip dislocation. J Arthroplasty 2001;16:806–809.
10. Collier JP, Mayor MB, Jensen RE, et al. Mechanisms of failure of modular prostheses. Clin Orthop Relat Res 1992;285:129–139.
11. Collier JP, Mayor MB, Williams IR, et al. The tradeoffs associated with modular hip prostheses. Clin Orthop Relat Res 1995;311:91–101.
12. Cooper HJ, Della Valle CJ, Berger RA, et al. Corrosion at the head-neck taper as a cause for adverse local tissue reactions after total hip arthroplasty. J Bone Joint Surg Am 2012;94(18):1655–1661.
13. Dangles CJ, Altstetter CJ. Failure of the modular neck in a total hip arthroplasty. J Arthroplasty 2010;25(7):1169.e5–1169.e7.
14. Della Valle CJ, Berger RA, Shott S, et al. Primary total hip arthroplasty with a porous-coated acetabular component. A concise follow-up of a previous report. J Bone Joint Surg Am 2004;86:1217–1222.
15. Della Valle CJ, Paprosky WG. The middle-aged patient with hip arthritis: the case for extensively coated stems. Clin Orthop Relat Res 2002;(405):101–107.
16. Duffy GP, Prpa B, Rowland CM, et al. Primary uncemented Harris-Galante acetabular components in patients 50 years old or younger: results at 10 to 12 years. Clin Orthop Relat Res 2004;427:157–161.
17. Engh CA Jr, Claus AM, Hopper RH Jr, et al. Long-term results using the anatomic medullary locking hip prosthesis. Clin Orthop Relat Res 2001;393:137–146.
18. Gilbert JL, Buckley CA, Jacobs JJ. In vivo corrosion of modular hip prosthesis components in mixed and similar metal combinations. The effect of crevice, stress, motion, and alloy coupling. J Biomed Mater Res 1993;27(12):1533–1544.
19. Gill IP, Webb J, Sloan K, et al. Corrosion at the neck-stem junction as a cause of metal ion release and pseudotumour formation. J Bone Joint Surg Br 2012;94:895–900.
20. Grant P, Nordsletten L. Total hip arthroplasty with the Lord prosthesis. A long-term follow-up study. J Bone Joint Surg Am 2004;86:2636–2641.
21. Grubl A, Chiari C, Gruber M, et al. Cementless total hip arthroplasty with a tapered, rectangular titanium stem and a threaded cup: a minimum ten-year follow-up. J Bone Joint Surg Am 2002;84:425–431.
22. Grupp TM, Weik T, Bloemer W, et al. Modular titanium alloy neck adapter failures in hip replacement—failure mode analysis and influence of implant material. BMC Musculoskelet Disord 2010;11:3.
23. Hallab NJ, Anderson S, Caicedo M, et al. Immune responses correlate with serum-metal in metal-on-metal hip arthroplasty. J Arthroplasty 2004;19(8):88–93.
24. Herrera A, Canales V, Anderson J, et al. Seven to 10 years followup of an anatomic hip prosthesis: an international study. Clin Orthop Relat Res 2004;423:129–137.
25. Huot Carlson J, Citters D, Currier J, et al. Femoral stem fracture and in vivo corrosion of retrieved modular femoral hips. J Arthroplasty 2012;27(7):1389–1396.
26. Jacobs JJ, Gilbert JL, Urban RM. Corrosion of metal orthopaedic implants. J Bone Joint Surg Am 1998;80:268–282.
27. Kim YH, Kim JS, Oh SH, et al. Comparison of porous-coated titanium femoral stems with and without hydroxyapatite coating. J Bone Joint Surg Am 2003;85:1682–1688.
28. Kim YH, Oh SH, Kim JS. Primary total hip arthroplasty with a second-generation cementless total hip prosthesis in patients younger than fifty years of age. J Bone Joint Surg Am 2003;85:109–114.
29. Kindsfater KA, Politi JR, Dennis DA, et al. The incidence of femoral component version change in primary THA using the S-ROM femoral component. Orthopedics 2011;11:34–38.
30. Kop AM, Swarts ES. Corrosion of a hip stem with a modular neck taper junction. J Arthroplasty 2009;24(7):1019–1023.
31. Kurtz M, Kocagöz BS, Hanzlik MS, et al. Do ceramic femoral heads reduce taper fretting corrosion in hip arthroplasty? A retrieval study. Clin Orthop Relat Res 2013;471:3270–3282.
32. Lachiewicz PF, Soileau E, Ellis J. Modular revision for recurrent dislocation for primary or revision total hip arthroplasty. J Arthroplasty 2004;19(4):424–429.
33. Laine HJ, Lehto MUK, Moilanen T. Diversity of the proximal femoral medullary canal. JA Arthroplasty 2000;15:86–92.
34. Leigh W, O’Grady P, Lawson E, et al. Pelvic pseudotumor: an unusual presentation of an extra-articular granuloma in a well-fixed total hip arthroplasty. J Arthroplasty 2008;23:934–938.
35. Mao X, Tay GH, Godbolt DB, et al. Pseudotumor in a well-fixed metal-on-metal polyethylene uncemented hip arthroplasty. J Arthroplasty 2012;27(3):493–938.
36. Marshall AD, Mokris JG, Reitman RD, et al. Cementless titanium tapered-wedge femoral stem: 10- to 15-year follow-up. J Arthroplasty 2004;19:546–552.
37. Meftah M, Nicolaou N, Rodriguez JA. Metal allergy response to femoral head-neck corrosion after total hip replacement. Curr Orthop Pract 2010;21:530.
38. Moskal JT, Jordan L, Brown TE. The porous-coated anatomic total hip prosthesis: 11- to 13-year results. J Arthroplasty 2004;19:837–844.
39. Noble PC, Alexander JW, Lindahl LJ, et al. The anatomic basis of femoral component design. Clin Orthop Rel Res 1988;235:148–165.
40. Ohl MD, Whiteside LA, McCarthy DS, et al. Torsional fixation of a modular femoral hip component. Clin Orthop Relat Res 1993;287: 135–141.
41. Otani T, Whiteside LA. Failure of cementless fixation of the femoral component in total hip arthroplasty. Orthop Clin North Am 1992;23: 335–346.
42. Park MS, Choi BW, Kim SJ, et al. Plasma spray-coated Ti femoral component for cementless total hip arthroplasty. J Arthroplasty 2003;18:626–630.
43. Parvizi J, Keisu KS, Hozack WJ, et al. Primary total hip arthroplasty with an uncemented femoral component: a long-term study of the Taperloc stem. J Arthroplasty 2004;19:151–156.
44. Parvizi J, Sharkey PF, Hozack WJ, et al. Prospective matched-pair analysis of hydroxyapatite-coated and uncoated femoral stems in total hip arthroplasty: a concise follow-up of a previous report. J Bone Joint Surg Am 2004;86:783–786.
45. Parvizi J, Sullivan T, Duffy G, et al. Fifteen-year clinical survivorship of Harris-Galante total hip arthroplasty. J Arthroplasty 2004;19:672–677.
46. Peak EL, Parvizi J, Ciminiello M, et al. The role of patient restrictions in reducing the prevalence of early dislocation following total hip arthroplasty. A randomized, prospective study. J Bone Joint Surg Am 2005;87:247–253.
47. Pellicci PM, Hass SB. Disassembly of a modular femoral component during closed reduction of the dislocated femoral component. A case report. J Bone Joint Surg Am 1990;72:619–620.
48. Robertson A, Lavalette D, Morgan S, et al. The hydroxyapatite-coated JRI-furlong hip. Outcome in patients under the age of 55 years. J Bone Joint Surg Br 2005;87:12–15.
49. Skendzel JG, Blaha JD, Urquhart AG. Total hip arthroplasty modular neck failure. J Arthroplasty 2011;26(2):338.e1–338.e4.
50. Sporer SM, DellaValle C, Jacobs J, et al. A case of disassociation of a modular femoral neck trunion after total hip arthroplasty. J Arthroplasty 2006;21(6):918–921.
51. Star MJ, Colwell CW Jr, Donaldson WF, et al. Dissociation of modular hip arthroplasty components after dislocation: a report of three cases at differing dissociation levels. Clin Orthop Relat Res 1992;278:111–115.
52. Svensson O, Mathiesen EB, Reinholt FP, et al. Formation of a fulminant soft-tissue pseudotumor after uncemented hip arthroplasty. A case report. J Bone Joint Surg Am 1988;70:1238–1242.
53. Teloken MA, Bissett G, Hozack WJ, et al. Ten to fifteen-year follow-up after total hip arthroplasty with a tapered cobalt-chromium femoral component (trilock) inserted without cement. J Bone Joint Surg Am 2002;84:2140–2144.
54. Udomkiat P, Dorr LD, Wan Z. Cementless hemispheric porous-coated sockets implanted with press-fit technique without screws: average ten-year follow-up. J Bone Joint Surg Am 2002;84:1195–2000.
55. Werner SD, Bono JV, Nandi S, et al. Adverse tissue reactions in modular exchangeable neck implants: a report of two cases. J Arthroplasty 2013;28:543.e13–543.e15.
56. Woolson ST, Potteroff GT. Disassembly of a modular femoral prosthesis after dislocation of the femoral component. A case report. J Bone Joint Surg Am 1990;72:624–625.
< div class='tao-gold-member'>
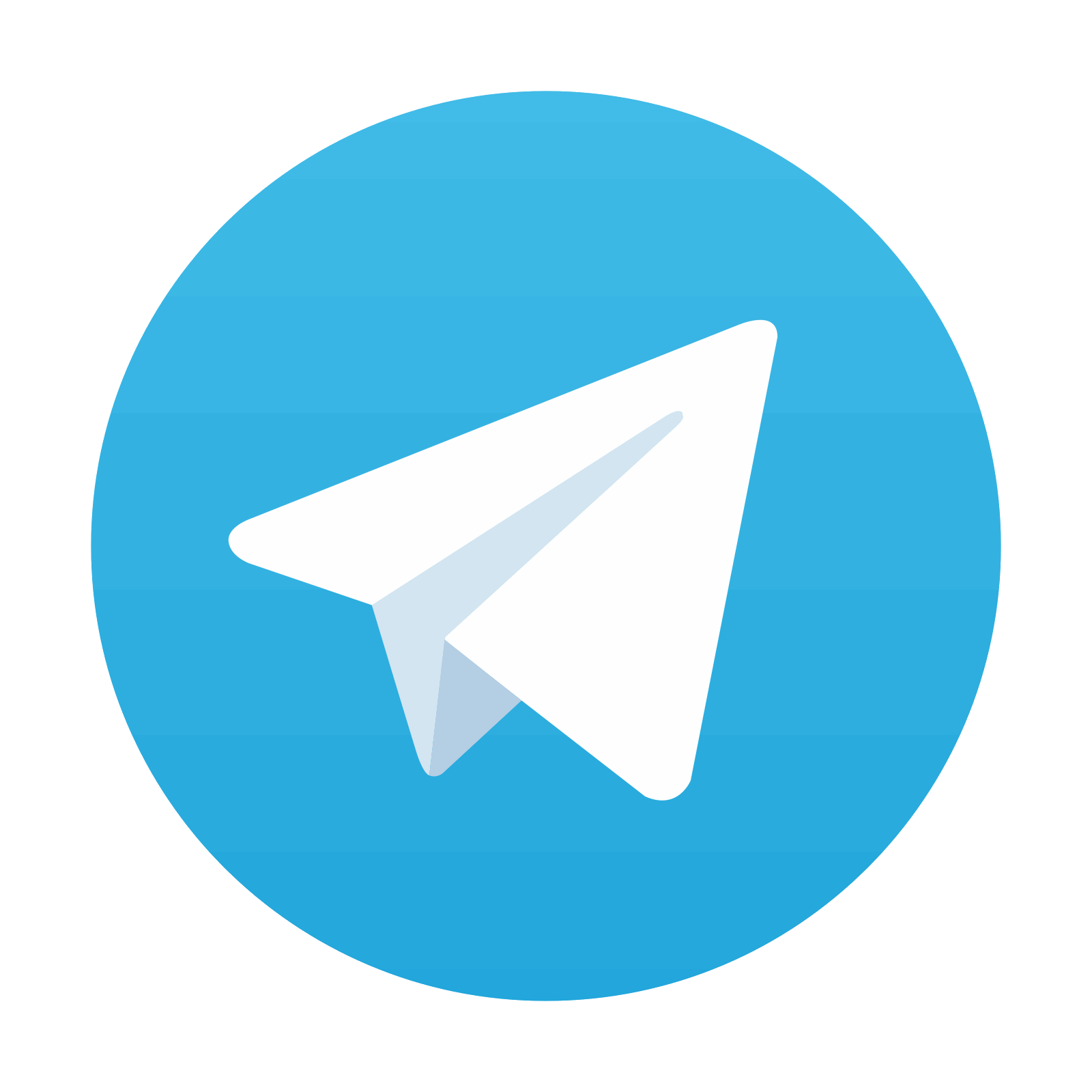