Each one of us is a self-organizing mass of multiple cell types. From fertilization of the embryo our tissue structures develop until an adult morphology is achieved. At that point our capacity for self-organization is directed to maintaining that morphology in the face of the insults of our daily life and the processes of aging. When a given insult overwhelms our capacity to repair by regeneration the result is scar repair.
Introduction
Each one of us is a self-organizing mass of multiple cell types. From fertilization of the embryo our tissue structures develop until an adult morphology is achieved. At that point our capacity for self-organization is directed to maintaining that morphology in the face of the insults of our daily life and the processes of aging. When a given insult overwhelms our capacity to repair by regeneration the result is scar repair.
We know that tissues retain a variable ability to heal by regeneration. With respect to the skin, in all but trivial injuries the capacity for regeneration is swamped, triggering the cellular mechanisms which result in scar formation. Burn injury is notorious for aggressive scars, compromising the individual functionally, cosmetically, and psychologically.
It has been well described that to heal a wound we need a source of cells capable of differentiation into the given tissue type and an extracellular matrix capable of supporting the cell migration, proliferation, and differentiation. We and others have spent considerable resources researching these areas to improve the speed and quality of wound healing to reduce the scar. The question we ask is: “How can we harness the technology of tissue engineering (TE) of skin to provide a controlled repair to restore the original morphology?”
TE can be defined as the application of engineering principles to biological systems. The healing of skin has been the subject of writings back to ancient times with attempts to stimulate healing, protect the surface while healing, and even replace the skin surface. So our recent explorations into using TE principles in skin repair are based on a significant history. With the increasing knowledge and understanding of the skin structure and function along with the developing TE techniques can we provide a regenerative repair avoiding scar?
We know that the skin provides the barrier to the external environment as a dynamic, complex three-dimensional structure made up of cells from all embryological layers. Integral to its functions are the vessels and nerves within the cell/matrix construct. Furthermore, skin is specialized over different body sites, adapting to local functional needs demonstrated by the macroscopic differences seen between areas, e.g. the eyelid compared with the palm of hand. The varying capacity to respond to injury is seen with the greater scar potential of sternal and deltoid areas. So as we go forward in developing tissue engineering solutions to skin’s it is timely to take stock of the skin functions and interactions. How will the skin changes over the different body sites influence the TE needs? Collaboration with specialists in bioinformatics will be essential in understanding the implication of changes in genes, genetic expression, and the phenotypic expression we need to guide to in the tissue constructs.
In the therapeutic use of TE the understanding of the etiology of the skin defect and pathophysiology of the patient will identify what needs to be replicated, rebuilt, and replaced. Implementation into clinical practice hinges on the TE being problem-driven, providing a practical, timely, cost-effective solution to the clinical problem. TE of skin has developed to this point in response to clinical need of, for example, skin repair in major burns, chronic ulcers, and giant nevi. It is clear that the current “gold standard” of skin grafting will always leave a scar. The development of TE gives the opportunity to tailor the repair to the defect with the understanding that one solution will not fit all. TE offers innovative solutions providing a spectrum of clinical solutions from strategies to facilitate wound healing in situ to multilayered skin constructs including multiple cell types.
The development of TE of skin is intimately linked to the vision of facilitating scar-free healing. It has broad implications post-trauma, -surgery, and -fibrosing pathologies where the common outcome is a functional compromise due to contracture and loss of normal tissue architecture. In the developed world it is estimated that 100 million patients acquire scars each year, some of which cause considerable problems, as a result of 55 million elective operations and 25 million operations after trauma. Within this number it is estimated that there are in the region of 11 million keloid scars and four million burn scars, 70% of which occur in children.
We are living in a time where science and technology are advancing at an exponential rate. Harnessing the power of that science and technology into clinical practice presents an ever-increasing challenge. We are all aware of the latest breakthrough holding promise to improve the quality of life from the health to the environmental sectors. However, it is worth noting that the exponential growth is possible in controlled systems where experiments can be designed to investigate a single variable; here lies the greatest challenge, for example, in burn injury research. The design of clinical trials is dogged by the complexities of assessing the extent of injury, the individual’s response, and the availability of validated outcome measures.
Clinical practice is a fusion of experience and knowledge with the development of medical subspecialization directed to a targeted problem-solving approach and has facilitated great advances in clinical care. However, this should not be at the expense of a broad general knowledge gaining insight into potential links and facilitating cross-fertilization. It is essential to link the tissue engineer with the clinical specialist to ensure the opportunities, risks, and benefits of TE skin are understood to facilitate appropriate clinical trials. By collaboration between disciplines there are real opportunities for improvements in clinical care translating to improved outcomes for patients.
The road from the bench to the bedside is a long one and navigates the areas of regulation, commercialization, reimbursement, and clinical trial design, to name a few. Also, translational research itself is an area in need of research and audit. The investigation of drivers and barriers to the implementation of TE skin solutions is an area of increasing effort. It is vital to learn from history and understand the current situation to continue developing innovative TE solutions but also innovative solutions within health systems, to action timely translation into clinical practice.
This chapter will:
- •
explore the functions of the skin and injury responses we need to understand in order to harness the TE technology effectively
- •
identify the needs which could be supplied by TE strategies
- •
discuss currently available technologies
- •
demonstrate TE skin solutions in clinical practice
- •
highlight the areas of need for further development.
Development, anatomy, and function of skin
Skin is commonly described as a multilayered physical barrier composed chiefly of the surface cellular epidermis and relatively acellular dermis. Exploring the development, anatomy, and skin functions demonstrates its complexity and will guide our TE endeavors.
“There is no magician’s mantle to compare with the skin in its diverse roles of waterproof, overcoat, sunshade, suit of armour and refrigerator. Sensitive to the touch of a feather, to temperature and pain, withstanding the wear and tear of three score years and ten and executing its own running repairs.”
During development the initial covering of the embryo is the periderm, which is thought to have a barrier function expressing tight junctions and interacts with the amniotic fluid having surface microvilli. It is interesting to note that the periderm cells express keratins also associated with migratory epidermal cells seen in wound healing. During the first 6 weeks the epidermis becomes two-layered, with the outer periderm and the inner developing epidermis. During this time there is no dermis but a subepidermal cellular layer with deposition of basement membrane type IV collagen and laminin by 5 weeks. By 8 weeks there is evidence of vascular development.
During the embryonic fetal transition stage at 9–10 weeks several changes are seen:
- •
epidermal cells express keratins 1 and 10, associated with differentiation
- •
maturation of the basement membrane with the development of cell adhesion and expression of integrins α6 and β4
- •
rapid deposition of dermal matrix
- •
migration of melanocytes from the neural crest
- •
Langerhans’ cells are detected originating in the fetal thymus and bone marrow
- •
Merkel cells are seen initially in the epidermis and subsequently in the dermis.
The early fetal period from 11 to 14 weeks sees the development of the hair follicles within the skin. The skin adnexal structures continue to develop and mature into the mid-fetal period at 15–20 weeks. As the fetus grows in the late period, 20–40 weeks, acceleration of stratum corneum can be identified in specific regions, including the palms, soles, face, and scalp. There is a close association between keratinization and hair follicle development with the stratum corneum developing initially in the perifollicular regions. The mature stratum corneum is a structure which develops in the late fetal period; a combination of the terminally differentiated keratinocytes forming a cornified envelope and lipid extrusion from the abundant lamellar bodies of the granular layer keratinocytes.
Embryologically the neural tissue and the epidermis are derived from ectoderm. By the end of the fourth week of embryonic development the neural ectoderm has separated from the surface ectoderm forming the neural tube. The nerve endings will never, under normal conditions, be exposed to the external environment. The skin forms the interface and has developed as the interactive responsive surface. In the following weeks mesoblastic cells from the neural crest migrate into the skin as melanoblasts and the early nerve fibers develop as the vasculature migrates into the mesodermal elements destined to become dermis. A close association is seen in development with the skin, developing as a tactile organ providing the feedback information from the surface to the developing CNS. The understanding of neural plasticity of the CNS and the peripheral nerve field underpins the self-organization principle. The co-development is put forward as an explanation of the co-evolution of the human CNS and the skin as an adaptive dynamic interface.
In the fully developed skin there are cells from all three embryological layers in a complex framework of extracellular matrix (ECM). There are functions common to all skin areas, but also there has been adaptation to the specific functions of given body sites which are seen even at the early fetal stages.
The mature epidermis is composed primarily of keratinocytes arising from a layer of basal cells situated on the basement membrane. As the keratinocytes differentiate they form a stratified squamous epithelium. As the cells undergo terminal differentiation they lose their nuclei and form a highly cross-linked protein-based layer of keratin. The basal cells are in intimate contact with terminal dendrites: synapse-like structures have been described between nerve endings and keratinocytes. The melanocytes are situated in the basal layer with the melanosomes being transferred to the differentiating keratinocytes giving the color of the skin due to pigment load. The cells linking to the immune system, Langerhans’ giant cells and dendritic cells, are also present in the epidermis. The epidermis is specialized to the body sites, most noticeably with the thickened cornified layer of the sole and palm.
The dermis is attached to the epidermis at the dermal–epidermal junction by the basement membrane morphologically arranged as the rete pegs, which are exaggerated at glaberous skin areas. The dermis is mainly connective tissue, predominantly collagen, with elastin seen in the superficial papillary dermis. The fibroblast is the cell that produces the ECM, which is specialized over differing body sites with areas such as the groin and axilla being more elastic thanthe thicker dermis of the back. Cells of hematopoietic origin such as lymphoctyes and macrophages migrate into the dermis and are involved in surveillance. The neural and vascular networks maintain the skin and facilitate the functions of the dynamic interactive skin interface.
The investigation of wound healing in parallel with an understanding of skin development and functions gives us the opportunity to further develop innovative methods of TE. The skin has developed specifically in relation to the multiple functions it performs. As an active organ it is responsive to changes in the external and internal environment, pivotal in maintaining the body’s homeostasis. Our knowledge of skin functions is still growing and includes:
- •
semi-permeable barrier, overcoat, suit of armor
- •
thermoregulation, refrigerator
- •
antibacterial, waterproof
- •
UV protection, sunshade
- •
sensory receptor, sensitive to the touch of a feather, to temperature, and to pain
- •
self-regenerating, withstanding the wear and tear of a lifetime
- •
capable of rapid repair, executing its own running repairs
- •
immune modulation
- •
psychological interaction
- •
vitamin production.
The loss of skin integrity can result in severe morbidity and even mortality. The body needs a barrier to the atmosphere to maintain homeostasis. The production of the stratum corneum can be mimicked in tissue culture by exposing a sheet of keratinocytes in culture to an air–liquid interface, but it requires maturation in situ to fully develop the “smart material” of the anucleated cell bodies and the extracellular lipoproteins moisturized by vitamin E-producing sebum.
The keratinocytes produce surface proteins which are antimicrobial in the first line of defense against the colonizing bacteria on the skin surface. The expression of these proteins changes as the keratinocyte is stressed, as in wounding or culturing, such that protection from microbial invasion is highlighted.
The multiple and specific sensory inputs to the skin are pivotal in the regulation of the body’s temperature, immune responses, and the psychological responses via neural and neuroendocrine control systems. Recent animal studies have highlighted the sensory role of the skin in normal development, with touch being associated with growth potential of the internal solid organs.
The skin is also profoundly influenced by the pathophysiology of the individual, with cutaneous changes in anatomy and physiology being linked to many disease processes.
It is with this expanding body of knowledge with respect to the skin that we engage the fields of TE to provide solutions for skin defects to maintain function and avoid scarring. To do so we also need a working knowledge of the skin response to injury and tissue loss; the processes of wound healing.
Briefly, wound healing in skin is a complex series of cascading events which has been described in three overlapping stages from the initial inflammation to tissue formation and subsequent tissue remodeling.
The initial response is clot formation to achieve hemostasis. The activation of platelets releases the contents of their alpha granules, resulting in the activation of the clotting cascade and the release of adhesive proteins forming the matrix of the clot, e.g. fibrin and chemotactic factors and growth factors into the wounded area. The coagulation pathway links to the activation of the complement pathway’s facilitation of the recruitment of neutrophils needed to facilitate the inflammatory response with the removal of cellular debris and microorganisms.
The in-growth of new vessels as granulation tissue is initiated and the keratinocytes at the wound edge mobilize to commence re-epithelialization. Macrophages migrate to the wound, releasing multiple protein growth factors as the wound response progresses to the repair phase. Both hemopoietic and mesenchymal stem cells from the circulation are attracted into the wound with fibroblasts producing ECM, some as myofibroblasts associated with wound contraction.
There is an increased interest in the interactions between the keratinocytes and underlying fibroblasts as the matrix is remodeled and the new basement membrane develops. As the keratinocyte is exposed to collagen it secretes collagenase and as the basement membrane integrity is restored the cells revert to their normal phenotype in the situation where healing is achieved without scarring.
In situations of more extensive tissue damage the fibroblast is of a scar phenotype with the production of disordered collagen. The interactions between the ECM and the fibroblasts respond to the changes as healing progresses from tissue repair to remodeling. The initial migratory fibroblast transitions to the profibrotic phenotype producing ECM proteins. In the remodeling phase the cell number within the dermis or scar falls as the cells undergo apoptosis.
A knowledge of the wound-healing progression over time allows the TE skin solution to be clinically integrated into the process and may be directed at a number of target strategies: the control of cells in growth, the genetic manipulation of cells to express a given phenotype, seeding of the retained dermis with cells from the dermal–epidermal junction, removal of the full thickness of the area of compromised skin, and replacement with a TE construct.
Development, anatomy, and function of skin
Skin is commonly described as a multilayered physical barrier composed chiefly of the surface cellular epidermis and relatively acellular dermis. Exploring the development, anatomy, and skin functions demonstrates its complexity and will guide our TE endeavors.
“There is no magician’s mantle to compare with the skin in its diverse roles of waterproof, overcoat, sunshade, suit of armour and refrigerator. Sensitive to the touch of a feather, to temperature and pain, withstanding the wear and tear of three score years and ten and executing its own running repairs.”
During development the initial covering of the embryo is the periderm, which is thought to have a barrier function expressing tight junctions and interacts with the amniotic fluid having surface microvilli. It is interesting to note that the periderm cells express keratins also associated with migratory epidermal cells seen in wound healing. During the first 6 weeks the epidermis becomes two-layered, with the outer periderm and the inner developing epidermis. During this time there is no dermis but a subepidermal cellular layer with deposition of basement membrane type IV collagen and laminin by 5 weeks. By 8 weeks there is evidence of vascular development.
During the embryonic fetal transition stage at 9–10 weeks several changes are seen:
- •
epidermal cells express keratins 1 and 10, associated with differentiation
- •
maturation of the basement membrane with the development of cell adhesion and expression of integrins α6 and β4
- •
rapid deposition of dermal matrix
- •
migration of melanocytes from the neural crest
- •
Langerhans’ cells are detected originating in the fetal thymus and bone marrow
- •
Merkel cells are seen initially in the epidermis and subsequently in the dermis.
The early fetal period from 11 to 14 weeks sees the development of the hair follicles within the skin. The skin adnexal structures continue to develop and mature into the mid-fetal period at 15–20 weeks. As the fetus grows in the late period, 20–40 weeks, acceleration of stratum corneum can be identified in specific regions, including the palms, soles, face, and scalp. There is a close association between keratinization and hair follicle development with the stratum corneum developing initially in the perifollicular regions. The mature stratum corneum is a structure which develops in the late fetal period; a combination of the terminally differentiated keratinocytes forming a cornified envelope and lipid extrusion from the abundant lamellar bodies of the granular layer keratinocytes.
Embryologically the neural tissue and the epidermis are derived from ectoderm. By the end of the fourth week of embryonic development the neural ectoderm has separated from the surface ectoderm forming the neural tube. The nerve endings will never, under normal conditions, be exposed to the external environment. The skin forms the interface and has developed as the interactive responsive surface. In the following weeks mesoblastic cells from the neural crest migrate into the skin as melanoblasts and the early nerve fibers develop as the vasculature migrates into the mesodermal elements destined to become dermis. A close association is seen in development with the skin, developing as a tactile organ providing the feedback information from the surface to the developing CNS. The understanding of neural plasticity of the CNS and the peripheral nerve field underpins the self-organization principle. The co-development is put forward as an explanation of the co-evolution of the human CNS and the skin as an adaptive dynamic interface.
In the fully developed skin there are cells from all three embryological layers in a complex framework of extracellular matrix (ECM). There are functions common to all skin areas, but also there has been adaptation to the specific functions of given body sites which are seen even at the early fetal stages.
The mature epidermis is composed primarily of keratinocytes arising from a layer of basal cells situated on the basement membrane. As the keratinocytes differentiate they form a stratified squamous epithelium. As the cells undergo terminal differentiation they lose their nuclei and form a highly cross-linked protein-based layer of keratin. The basal cells are in intimate contact with terminal dendrites: synapse-like structures have been described between nerve endings and keratinocytes. The melanocytes are situated in the basal layer with the melanosomes being transferred to the differentiating keratinocytes giving the color of the skin due to pigment load. The cells linking to the immune system, Langerhans’ giant cells and dendritic cells, are also present in the epidermis. The epidermis is specialized to the body sites, most noticeably with the thickened cornified layer of the sole and palm.
The dermis is attached to the epidermis at the dermal–epidermal junction by the basement membrane morphologically arranged as the rete pegs, which are exaggerated at glaberous skin areas. The dermis is mainly connective tissue, predominantly collagen, with elastin seen in the superficial papillary dermis. The fibroblast is the cell that produces the ECM, which is specialized over differing body sites with areas such as the groin and axilla being more elastic thanthe thicker dermis of the back. Cells of hematopoietic origin such as lymphoctyes and macrophages migrate into the dermis and are involved in surveillance. The neural and vascular networks maintain the skin and facilitate the functions of the dynamic interactive skin interface.
The investigation of wound healing in parallel with an understanding of skin development and functions gives us the opportunity to further develop innovative methods of TE. The skin has developed specifically in relation to the multiple functions it performs. As an active organ it is responsive to changes in the external and internal environment, pivotal in maintaining the body’s homeostasis. Our knowledge of skin functions is still growing and includes:
- •
semi-permeable barrier, overcoat, suit of armor
- •
thermoregulation, refrigerator
- •
antibacterial, waterproof
- •
UV protection, sunshade
- •
sensory receptor, sensitive to the touch of a feather, to temperature, and to pain
- •
self-regenerating, withstanding the wear and tear of a lifetime
- •
capable of rapid repair, executing its own running repairs
- •
immune modulation
- •
psychological interaction
- •
vitamin production.
The loss of skin integrity can result in severe morbidity and even mortality. The body needs a barrier to the atmosphere to maintain homeostasis. The production of the stratum corneum can be mimicked in tissue culture by exposing a sheet of keratinocytes in culture to an air–liquid interface, but it requires maturation in situ to fully develop the “smart material” of the anucleated cell bodies and the extracellular lipoproteins moisturized by vitamin E-producing sebum.
The keratinocytes produce surface proteins which are antimicrobial in the first line of defense against the colonizing bacteria on the skin surface. The expression of these proteins changes as the keratinocyte is stressed, as in wounding or culturing, such that protection from microbial invasion is highlighted.
The multiple and specific sensory inputs to the skin are pivotal in the regulation of the body’s temperature, immune responses, and the psychological responses via neural and neuroendocrine control systems. Recent animal studies have highlighted the sensory role of the skin in normal development, with touch being associated with growth potential of the internal solid organs.
The skin is also profoundly influenced by the pathophysiology of the individual, with cutaneous changes in anatomy and physiology being linked to many disease processes.
It is with this expanding body of knowledge with respect to the skin that we engage the fields of TE to provide solutions for skin defects to maintain function and avoid scarring. To do so we also need a working knowledge of the skin response to injury and tissue loss; the processes of wound healing.
Briefly, wound healing in skin is a complex series of cascading events which has been described in three overlapping stages from the initial inflammation to tissue formation and subsequent tissue remodeling.
The initial response is clot formation to achieve hemostasis. The activation of platelets releases the contents of their alpha granules, resulting in the activation of the clotting cascade and the release of adhesive proteins forming the matrix of the clot, e.g. fibrin and chemotactic factors and growth factors into the wounded area. The coagulation pathway links to the activation of the complement pathway’s facilitation of the recruitment of neutrophils needed to facilitate the inflammatory response with the removal of cellular debris and microorganisms.
The in-growth of new vessels as granulation tissue is initiated and the keratinocytes at the wound edge mobilize to commence re-epithelialization. Macrophages migrate to the wound, releasing multiple protein growth factors as the wound response progresses to the repair phase. Both hemopoietic and mesenchymal stem cells from the circulation are attracted into the wound with fibroblasts producing ECM, some as myofibroblasts associated with wound contraction.
There is an increased interest in the interactions between the keratinocytes and underlying fibroblasts as the matrix is remodeled and the new basement membrane develops. As the keratinocyte is exposed to collagen it secretes collagenase and as the basement membrane integrity is restored the cells revert to their normal phenotype in the situation where healing is achieved without scarring.
In situations of more extensive tissue damage the fibroblast is of a scar phenotype with the production of disordered collagen. The interactions between the ECM and the fibroblasts respond to the changes as healing progresses from tissue repair to remodeling. The initial migratory fibroblast transitions to the profibrotic phenotype producing ECM proteins. In the remodeling phase the cell number within the dermis or scar falls as the cells undergo apoptosis.
A knowledge of the wound-healing progression over time allows the TE skin solution to be clinically integrated into the process and may be directed at a number of target strategies: the control of cells in growth, the genetic manipulation of cells to express a given phenotype, seeding of the retained dermis with cells from the dermal–epidermal junction, removal of the full thickness of the area of compromised skin, and replacement with a TE construct.
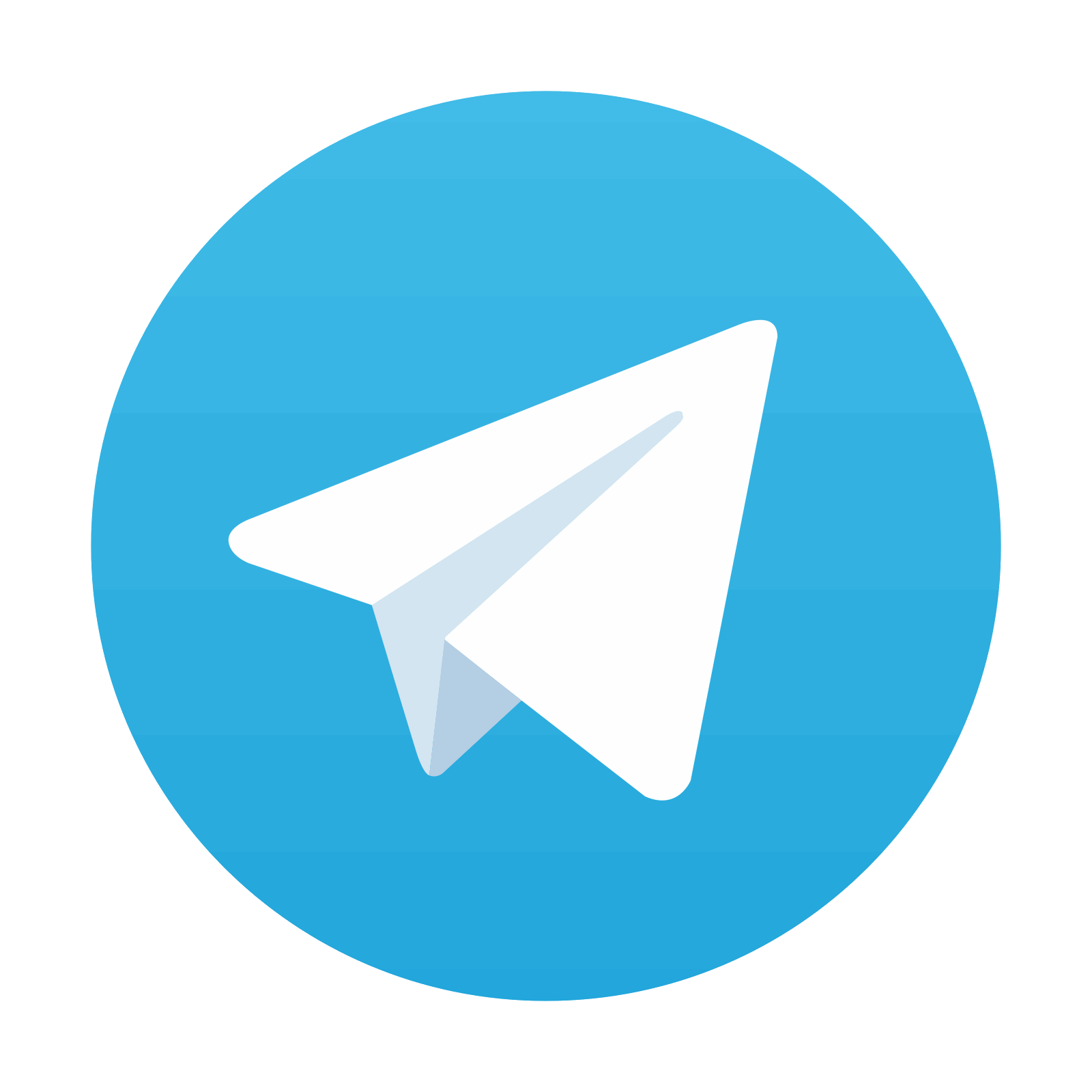
Stay updated, free articles. Join our Telegram channel

Full access? Get Clinical Tree
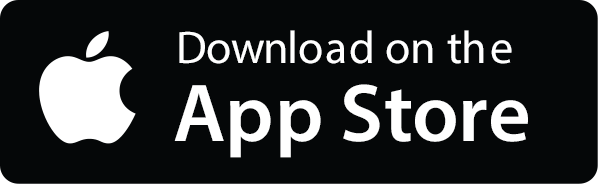
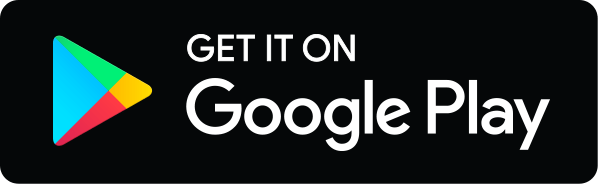