The authors present an overview of 3D computer-aided design and computer-aided modeling tools available to the facial plastic surgeon. They describe the role of 3D tools in all phases of computer-aided surgery including: data acquisition, planning, surgery, and assessment. Applications of these tools include obtaining 3D measurements, using mirror imaging to reconstruct missing areas of the head and neck, and 3D sizing or segmentation of bone and soft tissue. They review of clinical outcomes obtained from studies reviewing 3D tools. These systems have potential value for education, reducing operating room time, and improving clinical outcomes.
Despite the use of sound surgical technique, some outcomes in facial plastic surgery may be viewed as suboptimal to clinician and patient. Recently, there has been an explosion of 3-D imaging tools that have made their way from the research bench to clinical bedside, in an effort to assist surgeons in achieving optimal functional and esthetic results. The innovation of computer-aided design and computer-aided modeling (CAD/CAM) software, initially implemented in neurosurgery and radiology procedures, and the easy acquisition and transfer of Digital Imaging and Communications in Medicine (DICOM) data has facilitated the development of various proprietary software programs for use in the craniomaxillofacial skeleton. Contemporary software allows surgeons to analyze patients by performing 3-D measurements and to manipulate deformed or missing anatomy by mirror imaging, segmentation, or insertion of unaltered or ideal skeletal constructs. The virtual reconstruction may be transferred to reality (the patient) by using custom stereolithographic models (SLMs), implants, cutting jigs, or occlusal splints that are constructed using a CAD/CAM process, or through image-guided surgery in the form of intraoperative navigation (frameless stereotaxy) performed to the idealized virtual image. Finally, the accuracy of the surgical reconstruction may be confirmed using modern portable intraoperative CT scanners. Third-party service providers have made this technology available and accessible to most surgeons.
Computer-aided surgery can be divided into 4 phases:
- 1.
Data acquisition phase, in which all clinical information, anthropometric measurements, and bite registrations are obtained
- 2.
Planning phase, in which CT data are imported into a proprietary software program for the purposes of virtual planning before surgery
- 3.
Surgical phase, which is performed using CAD/CAM-derived SLMs, guide stents, occlusal splints, and/or intraoperative navigation
- 4.
Assessment phase, in which the accuracy of the treatment plan transfer is evaluated using intraoperative (or postoperative CT imaging).
Recently, basic and patient-oriented research has demonstrated efficacy of this approach in (1) head and neck/skull base surgery; (2) maxillomandibular reconstructive surgery; (3) orbital surgery ( Fig. 1 ), and (4) orthognathic/craniofacial surgery ( Fig. 2 ). The purpose of this article is to describe the authors’ approach to craniomaxillofacial surgery and how 3-D analysis and virtual surgery have affected the workflow process at their institution.
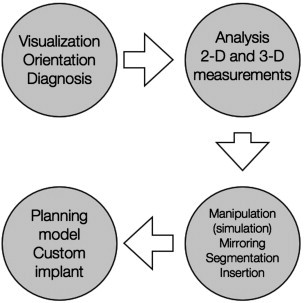
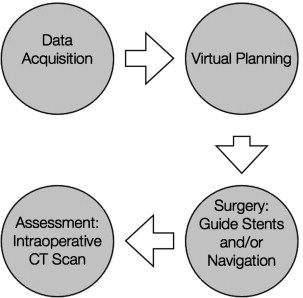
Data acquisition
Data acquisition occurs in the form of
- •
Quantifying the clinical deformity or problem through clinical examination
- •
Obtaining all necessary anatomic bite registrations and/or dental models
- •
Acquiring a CT scan of the patient, with the DICOM data to be imported into the appropriate planning software.
The clinical information necessary for computer planning depends on the clinical application. In head and neck surgery, for example, very specific information regarding the particular pathologic diagnosis (benign vs malignant), injury, hard and soft tissue involvement, and the extent of the planned surgical resection or reconstruction should be documented, but it is generally not necessary to obtain bite registrations. In contrast, computer-aided surgical simulation in orthognathic surgery requires registration of centric relation before CT imaging, registration of natural head position, laser scanning of plaster dental models, and high-quality facial photography, in addition to accurate anthropometric data.
Once the clinical evaluation is completed and presurgical records have been obtained, the CT scan is completed using the standard scanning algorithm, matrix of 512 × 512 at 0.625-mm slice thickness, 25 cm or lesser field of view, 0° gantry tilt, and 1:1 pitch. The CT images are then transferred to the appropriate surgical planning software in DICOM format.
Surface imaging, or scanning, is a technique in which a laser or other capturing device is used to replicate the 3-D surface of soft tissue, bones, or even dental cast. These data can then be imported digitally into a computer software program. Advantages compared with 3-D reconstruction of CT data include the lack of exposure to radiation and general anesthesia, particularly in the pediatric patient population. Surface scanning software imports individual points on the face, and then combines them in the X, Y, and Z axes rendering a 3-D representation of the soft-tissue skeleton. Therefore, the scans resolution is limited by the number of points in can imported into its data set, which is increasing with the development of higher-resolution laser acquisition software.
Facial surface acquisition devices are classified as either coordinate measurement machines, range imaging, moire, stereophotogrammetry, and position tracking devices. Recently, surface imaging devices have shown accuracy in the submillimeter range when compared with their real-life representations, becoming especially popular in the field of craniofacial/cleft lip and palate surgery where precision is crucial for flap design and movement. One group in particular has rigorously developed and tested a 3-D laser scanner for acquisition of the surface anatomy in the cleft/lip and palate population. The patient is scanned at a set distance (ie, 1 mm) as controlled by a tape measure fixed to the camera. The patient’s facial surface then reflects the distorted light of the laser, which is then captured by a charged-coupled distributor camera and converted into distance information using triangulation principles. The system then captures geometric points on a scatter plot. Using a software program, the scatter plot is then transferred into surface information via a software program, which can then manipulate the surfaces texture and color rendering an accurate representation of the soft tissue profile of the patient.
Preoperative planning
Surgical stimulation and manipulation of the 3-D facial skeleton using data derived from CT datasets can now be performed on several software systems. The planned surgery derived from preoperative surgical simulation can then be combined with intraoperative navigation to implement the plan, check its accuracy, and modify it if needed. The simplest, yet most studied and effective, way to perform hard tissue reconstruction is by mirroring the images of the opposite, unaffected side of the facial skeleton, to the affected side of the face using a variety of software programs. Virtual software allows segmentation of the area of interest and either virtual manipulation or reconstruction of a patient’s native anatomy, such as in congenital craniofacial defects, or insertion of implants into the virtual field, such as in traumatic or postablative reconstruction.
In the case of maxillomandibular reconstruction, the jaws can be virtually analyzed for horizontal, vertical, and sagittal discrepancies; 3-D measurements are obtained; and osteotomies are made, segmented, and manipulated in the X, Y, and Z axes and a virtual surgery can be undertaken in minutes. Furthermore, the resultant virtual outcome can then be transformed into an SLM to show the clinician and patient the final planned result. Patient-specific implants can be derived or implants can be prebent before surgery based on this idealized model. For complex orbital reconstruction, not only can implants be inserted and adapted into the virtual field but also plates can be constructed using the digital data derived from virtual hardware adaption.
Several CAD/CAM programs are currently available to the craniomaxillofacial surgeon ( Table 1 ). Although all have their advantages and drawbacks, several offer the specific feature of back-converting data from the software’s proprietary language to standard DICOM format. This allows the virtual data and subsequent work done in one software system to be transferred to another. The purpose of this may be to use components of several software systems that are unique to each or to display and teach via presentation at other workstations not loaded with the original software used to do a virtual procedure. In addition, the data set may be transferred into a navigation system, to guide the accurate placement of osteotomies, bone grafts, flaps, or implant placement.
Software Program | Cost (Estimates May Vary) |
---|---|
Amira (Visage Imaging GmbH, Berlin, Germany) | $4800 (base package) |
Analyze (AnalyzeDirect, Inc, Overland Park, Kansas) | $5000 |
iNtellect Cranial Navigation System (Stryker, Freiburg, Germany) | NA |
iPlan (BrainLab, Westchester, Illinois) | $30,000 (planning station and software) |
Maxilim (Medicim, Bruges, Belgium) | NA |
Mimics (Materialise, Leuven, Belgium) | NA |
SurgiCase CMF (Materialise, Leuven, Belgium) | $6500.00 |
SimPlant OMS (Materialise Dental, Leuven, Belgium) | NA |
Voxim (IVS Solutions, Chemnitz, Germany) | $20,000 (Voxim basics) |
3dMD (Atlanta, Georgia) | NA |
Alma3D (Alma IT Systems, Barcelona, Spain) | NA |
ImageJ (National Institutes of Health, Boston, Masssachusetts) | Free ( http://rsbweb.nih.gov/ij/ ) |
Preoperative planning
Surgical stimulation and manipulation of the 3-D facial skeleton using data derived from CT datasets can now be performed on several software systems. The planned surgery derived from preoperative surgical simulation can then be combined with intraoperative navigation to implement the plan, check its accuracy, and modify it if needed. The simplest, yet most studied and effective, way to perform hard tissue reconstruction is by mirroring the images of the opposite, unaffected side of the facial skeleton, to the affected side of the face using a variety of software programs. Virtual software allows segmentation of the area of interest and either virtual manipulation or reconstruction of a patient’s native anatomy, such as in congenital craniofacial defects, or insertion of implants into the virtual field, such as in traumatic or postablative reconstruction.
In the case of maxillomandibular reconstruction, the jaws can be virtually analyzed for horizontal, vertical, and sagittal discrepancies; 3-D measurements are obtained; and osteotomies are made, segmented, and manipulated in the X, Y, and Z axes and a virtual surgery can be undertaken in minutes. Furthermore, the resultant virtual outcome can then be transformed into an SLM to show the clinician and patient the final planned result. Patient-specific implants can be derived or implants can be prebent before surgery based on this idealized model. For complex orbital reconstruction, not only can implants be inserted and adapted into the virtual field but also plates can be constructed using the digital data derived from virtual hardware adaption.
Several CAD/CAM programs are currently available to the craniomaxillofacial surgeon ( Table 1 ). Although all have their advantages and drawbacks, several offer the specific feature of back-converting data from the software’s proprietary language to standard DICOM format. This allows the virtual data and subsequent work done in one software system to be transferred to another. The purpose of this may be to use components of several software systems that are unique to each or to display and teach via presentation at other workstations not loaded with the original software used to do a virtual procedure. In addition, the data set may be transferred into a navigation system, to guide the accurate placement of osteotomies, bone grafts, flaps, or implant placement.
Software Program | Cost (Estimates May Vary) |
---|---|
Amira (Visage Imaging GmbH, Berlin, Germany) | $4800 (base package) |
Analyze (AnalyzeDirect, Inc, Overland Park, Kansas) | $5000 |
iNtellect Cranial Navigation System (Stryker, Freiburg, Germany) | NA |
iPlan (BrainLab, Westchester, Illinois) | $30,000 (planning station and software) |
Maxilim (Medicim, Bruges, Belgium) | NA |
Mimics (Materialise, Leuven, Belgium) | NA |
SurgiCase CMF (Materialise, Leuven, Belgium) | $6500.00 |
SimPlant OMS (Materialise Dental, Leuven, Belgium) | NA |
Voxim (IVS Solutions, Chemnitz, Germany) | $20,000 (Voxim basics) |
3dMD (Atlanta, Georgia) | NA |
Alma3D (Alma IT Systems, Barcelona, Spain) | NA |
ImageJ (National Institutes of Health, Boston, Masssachusetts) | Free ( http://rsbweb.nih.gov/ij/ ) |
Surgery
Virtual surgical planning in and of itself is useful as an educational and teaching instrument by allowing 3-D analysis of the clinical problem and visualization of idealized digital manipulations. Without the ability to transfer the virtual plan into reality, however, it remains just that: an educational tool. The incorporation of CAD/CAM technology into the head and neck work flow process at our institution has allowed replicating the virtual plan in patients and theoretically positively affecting functional and esthetic treatment outcomes, reducing operating times, and improving quality.
The preoperative virtual plan is transferred to the patient in 1 of 3 ways, depending on the clinical problem. Reconstruction of the mandible and the inset of microvascular bone flaps may be facilitated by using virtually reconstructed or idealized SLMs, guide stents, and cutting guides. In the maxilla and midface, the cutting guides are augmented by intraoperative navigation, which is also used to aid in orbital reconstruction and skull base surgery. Orthognathic surgery is facilitated by the use of intermediate and final occlusal splints designed based on the virtual reconstruction.
Stereolithographic Modeling
Developed and refined in the later part of the twentieth century, SLMs are 3-D representations of the craniomaxillofacial skeleton. Using a variety of manufacturing techniques and materials, they are a direct representation of a patient’s CT data set. Early on, SLMs were milled from 2-D CT data. These models were useful as an adjunct in preoperative planning and helpful in getting a general view of the craniomaxillofacial skeleton, but by no means were taken for an exact representation of a patient’s hard tissues. With the advent of high-resolution, however, CT the resultant accuracy of SLMs has increased immensely. Current manufacturing protocols fabricate models that are highly accurate and are fabricated with a photocurable monomer hardened by lasers. SLMs may assist surgeons in preoperative planning and intraoperative bony reconstruction. These models offer an unprecedented level of accuracy and variations of these techniques continue to be developed and used today.
The utility of SLMs is in the preoperative staging of facial surgery patients. There has been an abundance of studies demonstrating their efficacy in the preoperative stages of maxillomandibular reconstruction including anteroposterior jaw positioning, guiding plate adaptation, maxillomandibular contouring, and craniofacial and dental implant placement. SLMs are used in orthognathic surgery and distraction osteogenesis and give surgeons a once unobtainable 3-D view of the maxilla and mandible, allowing precise visualization of the pitch, row, and yaw of planned maxillomandibular movement and vector plane in distraction osteogenesis. Craniofacial surgery has also reaped the benefits of SLMs using them in the preoperative planning of craniofacial recontouring and skeletal segment repositioning. Likewise, preoperative planning using SLMs in head and neck tumor reconstruction has been implemented in the reconstruction of oncologic defects by aiding preoperative plate adaptation, planned tumor resection, and custom implant and osteocutaneous flap placement. The reconstruction of complex orbital defects secondary to trauma or tumor ablation has significantly benefited from SLMs. SLMs allow surgeons to visualize deep into the bony orbit and to adapt stock implants or construct custom plates to the intricate anatomy of the orbit. Bell and Markiewicz demonstrated the use of SLMs on a cohort of patients with complex secondary traumatic defects and found SLMs extremely useful in reducing intraoperative plate adaptation and bone graft recontouring.
The drawbacks of SLMs lie in their poor representation of the midface and possibly the orbit where the thin walls of these areas of the facial skeleton may be misrepresented by lower-resolution CT and may not be as accurately represented as other craniomaxillofacial structures. Therefore, it is pivotal that patients receive high-resolution CT when SLMs are implemented. One area of poor representation of the facial skeleton that cannot be ameliorated by high-resolution CT are the occlusal surfaces of the dentition. This becomes critical in the preoperative planning of the orthognathic surgery and distraction osteogenesis patient. Recently, Xia and colleagues circumvented this dilemma by digitally substituting laser scans of the teeth of plaster models for the low-resolution teeth of the CT, thereby allowing the surgeon to making accurate movements of the jaws and to digitally fabricate highly accurate intraoperative and final occlusal stents.
Intraoperative Navigation
Intraoperative navigation software programs import a DICOM CT data set and allow surgeons real-time confirmation of their position within the craniomaxillofacial skeleton. Intraoperative navigation has 3 key components:
- 1.
Localizer, which is the camera or device that tracks the movements of the
- 2.
Surgical probe, which is the actual position of the patient currently on the operating table
- 3.
CT scan data set, which is projected and confirms the position of the probe within the patient to the preoperative 3-D image.
A patient’s position in space is maintained by the tracking system via the placement of fiducial markers on stable and reliable anatomic points on the patient. The fiducial markers may be invasive and fixed, or noninvasive. In the invasive technique, the fiducial markers are secured into the patient’s facial skeleton with screws via small incisions into the scalp or by the use of a custom splint that is adapted precisely to the patient face. When using these types of fiducial markers, the patient’s head must be immobilized by attaching it to a Mayfield headrest. Noninvasive, surface fiducial markers are now commercially available in the form of individual adhesive markers or light-emitting diode (LED) mask. This technique is much quicker and far less invasive than the fixed techniques and offers more mobility of the patient during surgery. Disadvantages of the noninvasive technique include if the mask is in the operating zone, such as when a coronal flap is used. In this instance, the fixed technique must be used. A third, yet more time-consuming method is the markerless technique, where a set of points on the patients face are scanned and correlated with the CT data set. The position of the surgical probe relative to the patient is determined by the computer using the local rigid body concept for which there must be at least 3 registration points (fiducial markers). A registration process is then undertaken in which the navigation software integrates the spatial information from the headset, surgical probe, and fiducial markers and the end result is alignment of the Cartesian coordinates of the actual patient in the X, Y, and Z planes onto the computer monitor in comparison with the preoperative CT data set.
Tracking systems have changed dramatically in recent years. Older navigation systems use electromagnetic fields that are superimposed over the operative site tracking the surgical probe, which may be by itself, or attached to an instrument of the surgeon’s choice. These systems, however, carried the disadvantage of interference from the metallic surgical instruments used during an operation. More recent systems use an optical tracking system design, similar to the current motion activated gaming systems available today for the public. They rely on LEDs or reflective spheres that are tracked by the optical systems. Optical tacking systems can be divided into 2 categories, active and passive trackers. Active trackers are equipped with battery-powered LEDs attached to the surgical probe. These probes can be placed anywhere in the body with a high degree of accuracy. Passive optical tacking systems have reflectors on the surgical probe instead of LEDs and have the advantage of not needing electrical wires or batteries attached to the probe making for a lighter probe with more flexible maneuverability. The major disadvantage of passive optical tacking systems, however, is that artificial light sources, such as operating room lights, may interfere with tracking.
Initially developed for neurosurgical and endoscopic sinus surgical procedures, intraoperative navigation is used for a variety of facial surgery procedures, including complex orbital repair, head and neck oncologic surgery, and craniofacial surgery. Intraoperative navigation offers the advantage of virtual visualization of a patient’s anatomy and access to areas of the facial skeleton that once required larger incisions and increased hard tissue exposure. There are several computer-based surgical navigation systems available for use in facial surgery ( Box 1 ). Using frameless stereotaxy, which literally means, touching a point in space , these programs allow precise location of anatomic landmarks or implants to with a margin of error of 1 mm to 2 mm when compared with the projected CT in the operating room. There are several evidence-based indications for intraoperative navigation in the facial surgery patient, which are elaborated in further detail in Box 2 .
Instatrak (General Electric Health Care, Buckinghamshire, UK)
Stealth Station (Medtronic-Xomed, Jacksonville, Florida)
Stryker Navigation System (Stryker-Leibinger, Kalamazoo, Michigan)
Vector Vision (BrainLab, Westchester, Illinois)
VoNaviX (IVS Solutions, Chemnitz, Germany)
Complex septoplasty/rhinoplasty
Facial implant placement
Head and neck tumor resection
Skull base surgery
Complex craniofacial/orthognathic surgery
Temporomandibular joint surgery
Foreign body removal
Complex orbital reconstruction
Maxillomandibular reconstruction
Cranial reconstruction
Evaluation
Once the virtual plan has been transferred to the patient by performing osteotomies or insetting grafts, flaps, or implants, the accuracy of the surgery is assessed (when appropriate) using a portable CT scanner. Available at many tertiary care facilities with an active neurosurgical presence, intraoperative CT assessment allows for any revision to occur before the patient leaving the operating room. This is particularly useful in orbital reconstruction, where the surgical access is limited and the relationship of the orbital implant relative to the orbital apex and critical medial and inferior bulges is unclear.
Clinical outcomes
Head and Neck/Skull Base Surgery
3-D imaging in the form of intraoperative CT ( Fig. 3 ) scanning has several benefits in head and neck oncologic surgery, originally reported in skull base surgery. Batra and colleagues found that the use of intraoperative CT in the resection of sinonasal and skullbase tumors offered advantages, such as evaluating the extent of tumor resection and margin confirmation and subsequent additional tumor resection, dissection of the ethmoid partitions, frontal stent placement, and frontal bone drilling. Preoperative 3-D CT imaging and intraoperative navigation has been shown an effective way to outline the margins of tumor resection in other areas of the craniomaxillofacial skeleton. Intraoperative navigation allows full visualization of the tumor using minimal exposure and morbidity to uninvolved tissues. Surgical resection of tumors of the pterygomaxillary fossa, skull base, infratemporal fossa, paranasal sinuses, and temporomandibular joint complex may be performed with increased maneuverability yet with the ability to virtually visualize and defer from involvement of adjacent vital structures without necessarily exposing the overlying tissues. Preoperative 3-D planning also affords surgeons the ability to perform osteotomies, either digitally or on SLMs manufactured from the CT data.
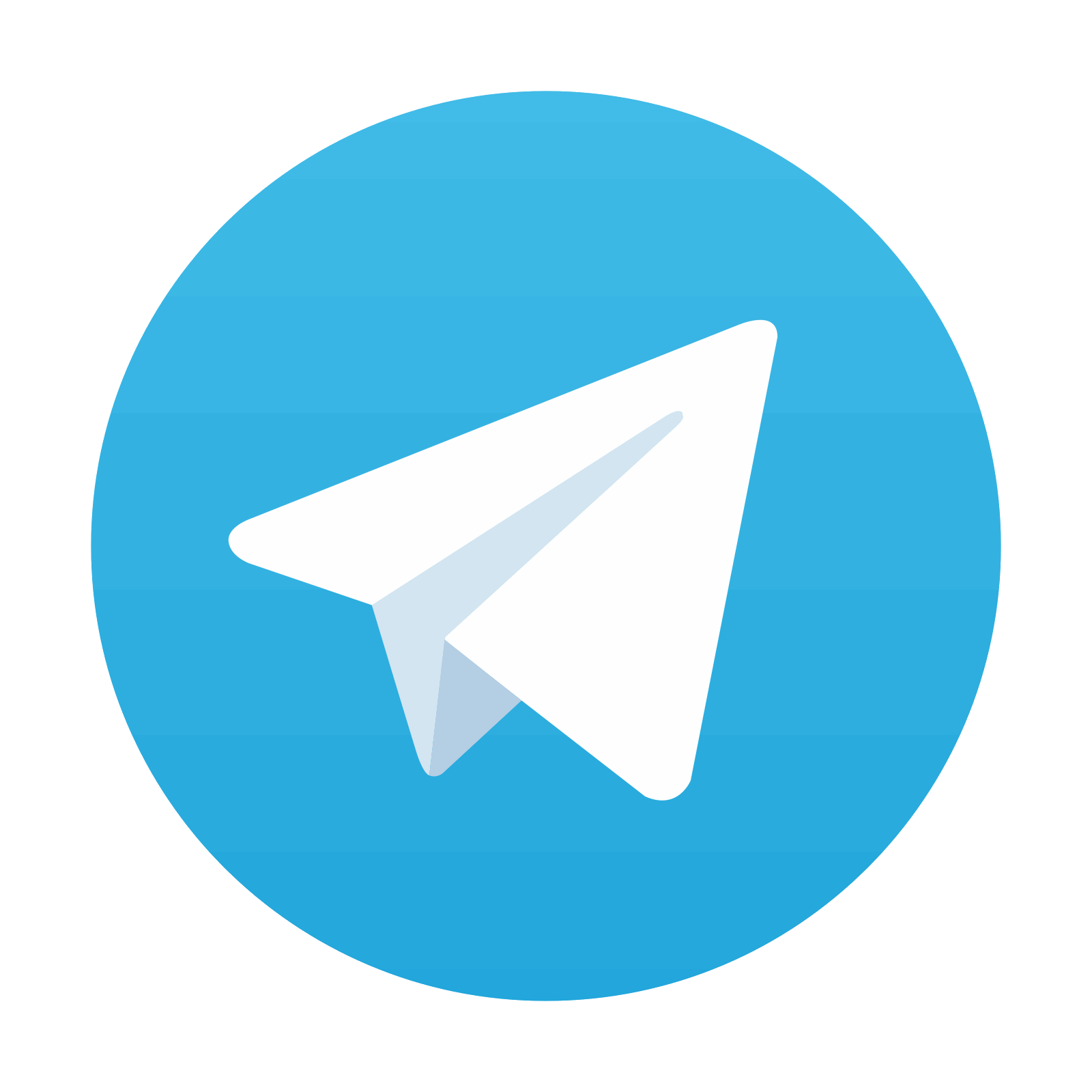
Stay updated, free articles. Join our Telegram channel

Full access? Get Clinical Tree
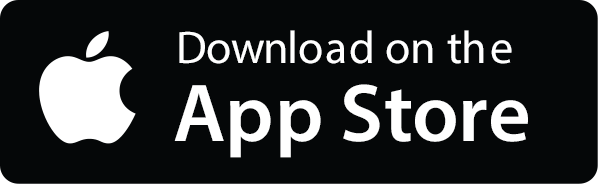
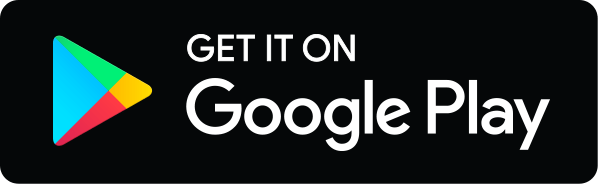
