2 The skin is the interface between humans and their environment. It weighs an average of 4 kg and covers an area of 2 m2. It acts as a barrier, protecting the body from harsh external conditions and preventing the loss of important body constituents, especially water. A death from destruction of skin, as in a burn, or in toxic epidermal necrolysis (p. 121), and the misery of unpleasant acne, remind us of its many important functions, which range from the vital to the cosmetic (Table 2.1). Table 2.1 Functions of the skin. The skin has three layers. The outer one is the epidermis, which is firmly attached to, and supported by connective tissue in the underlying dermis. Beneath the dermis is loose connective tissue, the subcutis or hypo-dermis, which usually contains abundant fat (Figure 2.1). Figure 2.1 Three-dimensional diagram of the skin, including a hair follicle. The epidermis consists of many layers of closely packed cells, the most superficial of which are flattened and filled with keratins; it is therefore a stratified squamous epithelium. It adheres to the dermis at the basement membrane where downward projections (epidermal ridges or pegs) interlock with upward projections of the dermis (dermal papillae) (Figure 2.1). The epidermis contains no blood vessels. It varies in thickness from less than 0.1 mm on the eyelids to nearly 1 mm on the palms and soles. As dead surface squames are shed (accounting for some of the dust in our houses), the thickness is kept constant by cells dividing in the deepest (basal) layer. A generated cell moves, to the surface, passing through the prickle and granular cell layers before dying in the horny layer. The journey from the basal layer to the surface (epidermal turnover or transit time) takes about 30 days. During this time the appearance and function of the cell changes in a process known as terminal differentiation. A vertical section through the epidermis summarizes the life history of a single epidermal cell (Figure 2.2). Figure 2.2 Changes during keratinization. The basal layer, the deepest layer, rests on a basement membrane, which attaches it to the dermis. It is a single layer of columnar cells, whose basal surfaces sprout many fine processes and hemidesmosomes, anchoring them to the lamina densa of the basement membrane. In normal skin some 30% of basal cells are preparing for division (growth fraction). Following mitosis, a cell enters the G1 phase, synthesizes RNA and protein, and grows in size (Figure 2.3). Later, when the cell is triggered to divide, DNA is synthesized (S phase) and chromosomal DNA is replicated. A short post-synthetic (G2) phase of further growth occurs before mitosis (M). DNA synthesis continues through the S and G2 phases, but not during mitosis. The G1 phase is then repeated, and one of the daughter cells moves into the suprabasal layer. It then differentiates (Figure 2.2), having lost the capacity to divide, and synthesizes keratins. Some basal cells remain inactive in a so-called G0 phase but may re-enter the cycle and resume proliferation. Stem cells reside amongst the interfollicular basal cells, the base of sebaceous glands, and amongst the cells of the external root sheath at the bulge in the hair follicle at the level of attachment of the arrector pili muscle. These cells divide infrequently, but can generate new proliferative cells in the epidermis and hair follicle in response to damage. Figure 2.3 The cell cycle. The spinous or prickle cell layer (Figure 2.4) is composed of keratinocytes. These differentiating cells, which synthesize keratins, are larger than basal cells. Keratinocytes are firmly attached to each other by small interlocking cytoplasmic processes, by abundant desmosomes, and by other cadherins separated by an intercellular layer of glycoproteins and lipoproteins. Under the light microscope, the desmosomes look like ‘prickles’. They are specialized attachment plaques that physically bind adjacent keratinocytes to one another and connect keratin intermediate filaments within keratinocytes to the cell membrane. Desmosomes are composed of transmembranous desmoglein–desmocollin pairs, which bind to the tonofilaments via desmoplakins, plakoglobin and plakophilin-1. There are four types of desmoglein found in the epidermis. Desmoglein (Dsg) 1 is expressed in the upper epidermis while Dsg 3 is mostly expressed in the basal epidermis. Desmoglein 1 is expressed at lower levels in the mucosal epithelium than Dsg 3. Autoantibodies to the desmogleins are found in pemphigus (p. 113), when they are responsible for the detachment of keratinocytes from one another and so for intraepidermal blister formation. Cytoplasmic continuity between keratinocytes occurs at gap junctions, specialized areas on opposing cell walls. Tonofilaments are small fibres running from the cytoplasm to the desmosomes. They are more numerous in cells of the spinous layer than of the basal layer, and are packed into bundles called tonofibrils. Many lamellar granules (otherwise known as membrane-coating granules, Odland bodies or keratinosomes), derived from the Golgi apparatus, appear in the superficial keratinocytes of this layer. They contain polysaccharides, hydrolytic enzymes, and stacks of lipid lamellae composed of phospholipids, cholesterol and glucosylceramides. Their contents are discharged into the intercellular space of the granular cell layer to become precursors of the lipids in the intercellular space of the horny layer (see Barrier function below). Figure 2.4 Layers of the epidermis. (a) Light microscopy and (b) electron micrograph. Cellular differentiation continues in the granular layer, which normally consists of two or three layers of cells that are flatter than those in the spinous layer, and have more tonofibrils. As the name of the layer implies, these cells contain large irregular basophilic granules of keratohyalin, which merge with tonofibrils. These keratohyalin granules contain proteins, including involucrin, loricrin and profilaggrin, which is cleaved into filaggrin by specific phosphatases as the granular cells move into the horny layer. As keratinocytes migrate out through the outermost layers, their keratohyalin granules break up and their contents are dispersed throughout the cytoplasm. Filaggrin peptides aggregate the keratin cytoskeleton, collapsing it, and thus converting the granular cells to flattened squames. These make up the thick and tough peripheral protein coating of the horny envelope. Its structural proteins include loricrin and involucrin, the latter binding to ceramides in the surrounding intercellular space under the influence of transglutaminase. Filaggrin, involucrin and loricrin can all be detected histochemically and are useful as markers of epidermal differentiation. The horny layer (stratum corneum) is made of piled-up layers of flattened dead cells (corneocytes) – the bricks – separated by lipids – the mortar – in the intercellular space. Together these provide an effective barrier to water loss and to invasion by infectious agents and toxic chemicals. The corneocyte cytoplasm is packed with keratin filaments, embedded in a matrix and enclosed by an envelope derived from the keratohyalin granules. This envelope, along with the aggregated keratins that it encloses, gives the corneocyte its toughness, allowing the skin to withstand all sorts of chemical and mechanical insults. Horny cells normally have no nuclei or intracytoplasmic organelles, these having been destroyed by hydrolytic and degrading enzymes found in lamellar granules and the lysosomes of granular cells. All cells have an internal skeleton made up of microfilaments (7 nm diameter; actin), microtubules (20–35 nm diameter; tubulin), and intermediate filaments (10 nm diameter). Keratins (from the Greek keras meaning ‘horn’) are the main intermediate filaments in epithelial cells and are comparable to vimentin in mesenchymal cells, neurofilaments in neurons, and desmin in muscle cells. Keratins are not just a biochemical curiosity, as mutations in their genes cause a number of skin diseases including simple epidermolysis bullosa (p. 123) and bullous ichthyosiform erythroderma (p. 47). The keratins are a family of more than 30 proteins, each produced by different genes. These separate into two gene families: one responsible for basic and the other for acidic keratins. The keratin polypeptide has a central helical portion with a non-helical N-terminal head and C-terminal tail. Individual keratins exist in pairs so that their double filament always consists of one acidic and one basic keratin polypeptide. The intertwining of adjacent filaments forms larger fibrils. Different keratins are found at different levels of the epidermis depending on the stage of differentiation and disease; normal basal cells make keratins 5 and 14, but terminally differentiated suprabasal cells make keratins 1 and 10 (Figure 2.2). Keratins 6 and 16 become prominent in hyperproliferative states such as psoriasis. During differentiation, the keratin fibrils in the cells of the horny layer align and aggregate, under the influence of filaggrin. Cysteine, found in keratins of the horny layer, allows cross-linking of fibrils to give the epidermis strength to withstand injury. Firm cohesion in the spinous layer is ensured by ‘stick and grip’ mechanisms. A glycoprotein intercellular substance acts as a cement, sticking the cells together, and the intertwining of the small cytoplasmic processes of the prickle cells, together with their desmosomal attachments, accounts for the grip. The cytoskeleton of tonofibrils also maintains the cell shape rigidly. The typical ‘basket weave’ appearance of the horny layer in routine histological sections is artefactual and deceptive. In fact, cells deep in the horny layer stick tightly together and only those at the surface flake off; this is in part caused by the activity of cholesterol sulfatase. This enzyme is deficient in X-linked recessive ichthyosis (p. 46), in which poor shedding leads to the piling up of corneocytes in the horny layer. Desquamation is normally responsible for the removal of harmful exogenous substances from the skin surface. The cells lost are replaced by newly formed corneocytes; regeneration and turnover of the horny layer is therefore continuous. The horny layer prevents the loss of interstitial fluid from within, and acts as a barrier to the penetration of potentially harmful substances from outside. Solvent extraction of the epidermis leads to an increased permeability to water, and it has been known for years that essential fatty acid deficiency causes poor cutaneous barrier function. These facts implicate ceramides, cholesterol, free fatty acids (from lamellar granules; p. 10) and smaller quantities of other lipids, in cutaneous barrier formation. Natural moisturising factor (NMF), predominantly made up of amino acids and their metabolites, also helps maintain the properties of the stratum corneum. Barrier function is impaired when the horny layer is removed – experimentally, by successive strippings with adhesive tape, or clinically, by injury or skin disease. It is also decreased by excessive hydration or dehydration of the horny layer and by detergents. The relative impermeability of the stratum corneum and ‘moving staircase’ effect of continually shedding the outer corneocytes provides a passive barrier to infective organisms. In addition to this, protection is given by the various antimicrobial peptides (AMPs) found on epithelial surfaces. The two major families of AMPs are the defensins and the cathelicidins which have a broad range of antimicrobial activity and form the first line of immune defence of the body. The speed at which a substance penetrates through the epidermis is directly proportional to its concentration difference across the barrier layer, and inversely proportional to the thickness of the horny layer. A rise in skin temperature aids penetration. A normal horny layer is slightly permeable to water, but relatively impermeable to ions such as sodium and potassium. Some other substances (e.g. glucose and urea) also penetrate poorly, whereas some aliphatic alcohols pass through easily. The penetration of a solute dissolved in an organic liquid depends mainly on the qualities of the solvent. Both the thickness of the normal epidermis, and the number of cells in it, remain constant, as cell loss at the surface is balanced by cell production in the basal layer. Locally produced cytokines, transcription factors and integrins stimulate or inhibit epidermal proliferation and differentiation, interacting in complex ways to ensure homeostasis (Table 2.2). Table 2.2 Regulators of epidermopoiesis. The steroid 7-dehydrocholesterol, found in keratinocytes, is converted by sunlight to cholecalciferol. The vitamin becomes active after 25-hydroxylation in the kidney. Kidney disease and lack of sun, particularly in dark-skinned peoples, can both cause vitamin D deficiency and rickets. Low vitamin D levels have been linked to a wide range of diseases including multiple sclerosis, cardiovascular disease, hypertension and solid organ tumours. However, results of vitamin D supplementation trials have been variable. Vitamin D may merely be a marker for sun exposure, with other sun-related factors such as cutaneous nitric oxide leading to health improvements. Alternatively, the direction of association may be the other way, with unhealthy patients unable to spend as much time outside and thus prone to low sun exposure and vitamin D levels. Keratinocytes make up about 85% of cells in the epidermis, but three other types of cell are also found there: melanocytes, Langerhans cells and Merkel cells (Figure 2.5). Figure 2.5 Melanocyte, Langerhans cell and Merkel cell. Melanocytes are the only cells that can synthesize melanin. They migrate from the neural crest into the basal layer of the ectoderm where, in human embryos, they are seen as early as 8 weeks’ gestation. They are also found in hair bulbs, the retina and pia arachnoid. Each dendritic melanocyte associates with a number of keratinocytes, forming an epidermal melanin unit (Figure 2.5). The dendritic processes of melanocytes wind between the epidermal cells and end as discs in contact with them. Their cytoplasm contains discrete organelles, the melanosomes, containing varying amounts of the pigment melanin (Figure 2.6). This is ‘injected’ into surrounding keratinocytes to provide them with pigmentation to help protect the skin against damaging ultraviolet radiation. Figure 2.6 Melanocyte (electron micrograph), with melanosomes (inset). Melanogenesis is described at the beginning of Chapter 19 on disorders of pigmentation. The Langerhans cell is a dendritic cell (Figures 2.5 and 2.7) like the melanocyte. It also lacks desmosomes and tonofibrils, but has a lobulated nucleus. The specific granules within the cell look like a tennis racket when seen in two dimensions in an electron micrograph (Figure 2.8), or like a sycamore seed when reconstructed in three dimensions. They are plate-like, with a rounded bleb protruding from the surface. Figure 2.7 Adenosine triphosphase-positive Langerhans cells in an epidermal sheet. The network provides a reticulo-epithelial trap for contact allergens. Figure 2.8 Langerhans cell (electron micrograph), with characteristic granule (inset). Langerhans cells come from a mobile pool of precursors originating in the bone marrow. There are approximately 800 Langerhans cells per mm2 in human skin and their dendritic processes fan out to form a striking network seen best in epidermal sheets (Figure 2.7). Langerhans cells are alone among epidermal cells in possessing surface receptors for C3b and the Fc portions of IgG and IgE, and in bearing major histocompatibility complex (MHC) Class II antigens (HLA-DR, -DP and -DQ). They are best thought of as highly specialized macrophages. Langerhans cells have a key role in many immune reactions. They take up exogenous antigen, process it and present it to T lymphocytes either in the skin or in the local lymph nodes (p. 26). They probably play a part in immunosurveillance for viral and tumour antigens. Topical or systemic glucocorticoids reduce the density of epidermal Langerhans cells as does ultraviolet radiation. Merkel cells are found in normal epidermis (Figure 2.5) and act as transducers for fine touch. They are non-dendritic cells, lying in or near the basal layer, and are of the same size as keratinocytes. They are concentrated in localized thickenings of the epidermis near hair follicles (hair discs), and contain membrane-bound spherical granules, 80–100 nm in diameter, which have a core of varying density, separated from the membrane by a clear halo. Sparse desmosomes connect these cells to neighbouring keratinocytes. Fine unmyelinated nerve endings are often associated with Merkel cells, which express immunoreactivity for various neuropeptides. The skin appendages are derived from epithelial germs during embryogenesis and, except for the nails, lie in the dermis. They include hair, nails, and sweat and sebaceous glands. They are described, along with the diseases that affect them, in Chapters 12 and 13, respectively. The basement membrane lies at the interface between the epidermis and dermis. With light microscopy it can be highlighted using a periodic acid–Schiff (PAS) stain, because of its abundance of neutral mucopolysaccharides. Electron microscopy (Figure 2.9) shows that the lamina densa (rich in type IV collagen) is separated from the basal cells by an electron-lucent area, the lamina lucida. Hemidesmosomes span the basal plasma membrane of basal keratinocytes and structurally bind the epidermis to the dermis. They are complex structures made up of bullous pemphigoid antigens 1 (a 230-kDa plakin) and 2 (180 kDa collagen XVII), plectin and α6 β4 integrin. Antibodies to BP antigens 1 and 2 are found in pemphigoid (p. 117), a subcutaneous blistering condition. The intracellular component of the hemidesmosome binds to the intermediate filament network within keratinocytes. Figure 2.9 Structure and molecular composition of the dermo-epidermal junction. Fine anchoring filaments, largely composed of laminin-332, cross the lamina lucida and connect the lamina densa to the plasma membrane of the basal cells by interacting with the α6 β4 integrin component of the hemidesmosome. Anchoring fibrils (of type VII collagen), dermal microfibril bundles and single small collagen fibres (types I and III), extend from the papillary dermis to the deep part of the lamina densa. Laminins, large non-collagen glycoproteins produced by keratinocytes, aided by nidogen, promote adhesion between the basal cells above the lamina lucida and type IV collagen, the main constituent of the lamina densa, below it. Laminin 332 is the most important member of this family, which also includes laminins 311 and 511. Laminins bind to cells by interactions with cellular integrins. The structures within the dermo-epidermal junction provide mechanical support, encouraging the adhesion, growth, differentiation and migration of the overlying basal cells, and also act as a semipermeable filter that regulates the transfer of nutrients and cells from dermis to epidermis.
The Function and Structure of the Skin
Function
Structure/cell involved
Protection against:
chemicals, particles
Horny layer
ultraviolet radiation
Melanocytes
antigens, haptens
Langerhans cells
microbes
Langerhans cells
Preservation of a balanced internal environment
Horny layer
Prevents loss of water, electrolytes and macromolecules
Horny layer
Shock absorber
Dermis and subcutaneous fat
Strong, yet elastic and compliant
Temperature regulation
Blood vessels
Eccrine sweat glands
Insulation
Subcutaneous fat
Sensation
Specialized nerve endings
Lubrication
Sebaceous glands
Protection and prising
Nails
Calorie reserve
Subcutaneous fat
Vitamin D synthesis
Keratinocytes
Body odour/pheromones
Apocrine sweat glands
Psychosocial, display
Skin, lips, hair and nails
Epidermis
Keratinocytes
Keratinization
Cell cohesion and desquamation
The epidermal barrier
Epidermopoiesis and its regulation
Designation
Function
P63
Probable stem cell marker. Encourages stem cell proliferation
β1 integrin
TGF-α
C-myc
Drives stem cell proliferation
TGF-β
Inhibits stem cell proliferation
Notch signalling
PPARα
Controls epidermal differentiation
Vitamin D synthesis
Other cells in the epidermis
Melanocytes
Langerhans cells
Merkel cells
Epidermal appendages
Dermo-epidermal junction
Dermis
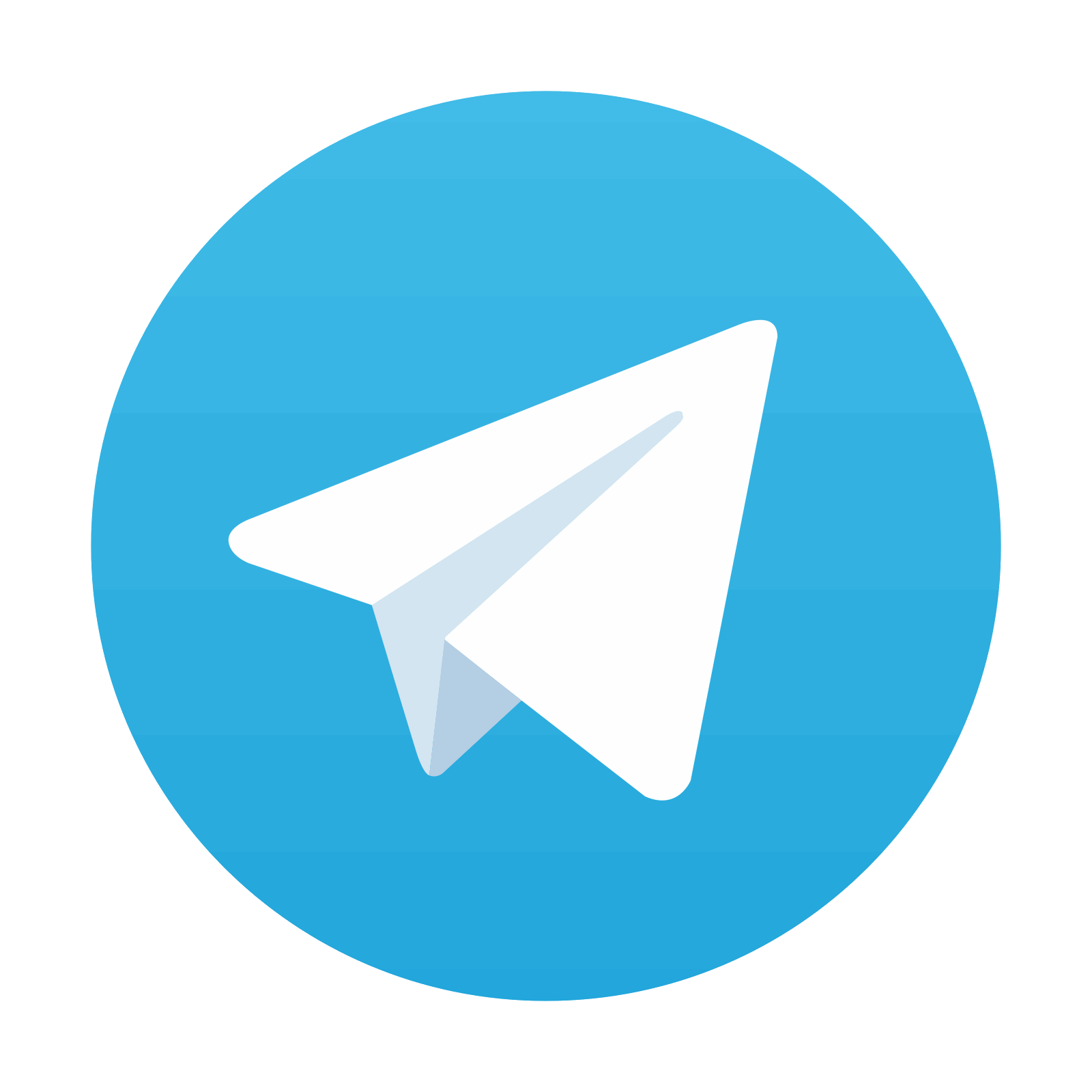
Stay updated, free articles. Join our Telegram channel

Full access? Get Clinical Tree
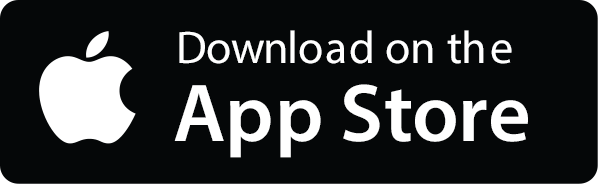
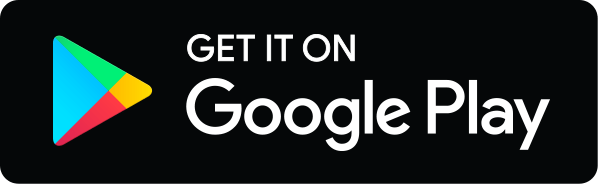