Fig. 23.1
Interaction between genetic factors, estrogen, and aging in the development of Th1/TH2 differentiation and subsequent autoimmune diseases in aging women (Reprinted with permission from Expert Rev Obs Gyn 7(6), 557–571 (2012) with permission of Expert Reviews Ltd)
Immunosenescence in both genders impacts cellular, humoral, and innate immunity [11]. Significant consequences of aging include atrophy of the thymus, changes in both the total numbers and subsets of lymphocytes, changes in the function of both B and T cells, changes in the patterns of secretion of cytokines and growth factors, disruption of intracellular signaling, changes in the patterns of antibody production, loss of antibody repertoire, loss of response to antigens and mitogens, and disruption of immunological tolerance (Table 23.1). Gender-specific increases in some aspects of immunosenescence have been observed and will be discussed below.
Table 23.1
Changes in immune function with age (both men and women)
Immune function | Change | Reference |
---|---|---|
B cell | Reduction in early progenitor B cells in the bone marrow | [110] |
Increase in polyclonal response against diverse mitogens | [8] | |
Decrease of specific antigen response | [8] | |
Production of antibodies independent of T-cell activation | [5] | |
Oligoclonal expansion of B cells | [5] | |
Increase in B1 cell fraction of B cells | [49] | |
Maturation of B cells blocked (believed to be inability to rearrange Ig genes because of decrease in expression of RAG-1 and RAG-2 recombinases | [111] | |
Reduced output of naïve B cells | [110] | |
Accumulation of oligoclonally expanded, functionally incompetent memory lymphocytes | [110] | |
Decreased numbers of circulating B cells | [112] | |
CD20+ B cells decrease | [15] | |
Reduced antigen-recognition repertoire of B cells | [113] | |
Class switch alterations in immunoglobulins produced | [114] | |
Stimulated B cells show significant alterations in cellular structures with role in signal transduction | [115] | |
Increase of IgA and several IgG subclasses | [116] | |
Lymphocytes | Reduction in total numbers | [59] |
Molecular | Alterations in cell surface receptors (e.g., loss of co-stimulatory receptor CD28 expression in CD8+ cells) | [117] |
Alterations in cytokine and cytokine receptor alterations in T cells | [118] | |
Alterations in effector molecules | ||
Interleukin 6 levels increase | [121] | |
Alterations in transcriptional regulators | [119] | |
Diminished RAG expression as result of defective bone marrow microenvironment | [5] | |
Reduced activity of transcription factors like E2A and Pax-5 | [5] | |
Systemic | Decline in humoral immunity | [119] |
Increased levels of circulating proinflammatory cytokines IL-6 and TNF-α | [122] | |
Decreased microbicidal activity against C. albicans and E. coli with reduced reactive oxygen species produced during the respiratory burst | [123] | |
Decreased response of activated neutrophils to cytokines that suppress apoptosis | [124] | |
Frequency of monoclonal gammopathies increases with age (doubles from 2 % at age 50 to 4 % at age 70) | [125] | |
NK cells | Total cell numbers increase | [15] |
NK cytotoxic function impaired | [5] | |
T-cell functions | Reduced diversity of T-cell repertoire | [119] |
Decrease of T-helper (Th) cells | [8] | |
Decrease of T-suppressor cells | [8] | |
Decrease in expression of lymphocyte growth factors | [8] | |
Decrease in expression of specific lymphocyte receptors | [8] | |
Disruption of intracellular signaling | [126] | |
Reduced diversity of antigen recognition repertoire (about 108 in young adults to 106 in elderly) | [127] | |
Impaired proliferation in response to antigenic stimulation | [128] | |
Changes in cytokine profiles | [119] | |
Atrophy of the thymus with reduction of thymic production of T cells | [129] | |
Substantial reduction in numbers of naïve lymphocytes | [129] | |
Impaired helper function of naïve CD4+ T cells | [130] | |
Decline in CD3+ and CD5 +populations | [15] | |
Accumulation of CD 28- CD8+ circulating T cells | [119] | |
Loss of CD28 which abrogates normal T-cell elimination | [131] | |
Decrease in total numbers of CD4+ and CD8+ cells | [15] | |
Decrease in suppressor-inducer naïve T cells (CD4+CD45RA+) decrease | [15] | |
Increase in helper-inducer T cells (CD4CD29+) | [15] | |
Increase in CD4+/CD8+ ratio | [15] | |
Clonal expansion of CD8+ T cells | [132] | |
Impaired response of CD44hiCD8+ cells to IL-15 | [133] | |
Impaired maturation of naïve CD4+ cells into Th1 or Th2 cells | [134] | |
Decreased postactivation of Il-2 | [135] | |
Alterations in several components of signaling complex in both memory and naïve T cells in aged mice | [126] | |
Naïve T cells defective, production of lower levels of Il-2, increased levels of Il-4, reduced proliferative capability, reduced capability of differentiation into effecter cells (much driven by Il-2 deficit) | [16] |
Although aging affects many immune cell types, the cumulative effects of aging on T-cell function are the most consistently observed and most extensive [12]. The human thymus decreases in both size and cellularity in a process called thymic involution; thymus tissue is replaced with fat [13]. By age sixty, thymus-derived hormones are absent from the circulation.
Involution of the thymus in humans occurs in concert with a depletion of naïve T cells and a shift in the T cell population towards memory CD4+ cells [14]. In young adulthood, the CD4+ subset is characterized by roughly equivalent numbers of memory and naïve CD4+ cells but in older adults becomes predominantly memory CD4+ [15], a shift which reduces the potential antigenic repertoire [16]. The shift towards memory T cells with age is largely a consequence of the imbalance in T-cell maturation produced by thymus involution [17] paired with an age-related impairment of T-cell proliferation [18] in concert with clonal expansion of T cells activated by specific antigens [16]. The shift towards memory cells in the T-cell compartment affects cytokine production as well, with less interleukin (IL)-2 produced (primarily a product of naïve T cells) but more IL-4 (primarily a product of memory T cells) [19].
The cumulative loss of T helper (Th) cells with age plays a profound role in immunosenescence, ultimately affecting both cellular and humoral immunity. Disruption of T-cell helper cells and alterations of cytokine levels which control B-cell functions compromise humoral immunity substantially, with decreased production of long-term immunoglobulin (Ig)-producing B cells as well as a reduction of Ig diversity [5]. Although B-cell numbers do not change significantly, there is a significant impairment of B-cell response to primary antigenic stimulation [16]; specific immunoglobulins produced become more random, and those produced have decreased affinity for their specific antigen [16]. With age, therefore, the B-cell repertoire poised to respond to new antigenic challenge is limited, and the predominance of memory T cells seen with thymic involution is mirrored in the B-cell compartment [20]. IL-15, particularly, stimulates proliferation of memory T cells; IL-15 levels are nearly double in healthy adults 95 or older (3.05 pg/mL as compared to both older adults [aged 60–89] 1.94 pg/mL and midlife adults [aged 30–59] 1.73 pg/mL) [21].
Immunosenescence is compounded by the presence in the aged of a chronic low-grade inflammation characterized by increased proinflammatory cytokines such as Il-6 and tumor necrosis factor alpha (TNF-α), compounds that create oxidative stress and decrease cellular antioxidant capacity [11]. These proinflammatory cytokines are positively associated with stress as well as salivary cortisol levels and may play a significant role in creating the degenerative changes associated with aging [18]. Other body processes, most notably innate immunity [22] and interactions of immunity with the neuroendocrine system [23], also contribute to immune system aging.
Antigen-presenting cells (APCs) such as dendritic cells and macrophages serve as a bridge between the innate and the adaptive immune systems. APCs interact with foreign molecules and release pathogen-specific cytokines that drive the activation of naïve CD4 helper cells into either Th1 or T helper 2 (Th2) effector cells [24].
Production of IL-12 and interferon gamma (IFN-γ) drives commitment of naïve T cells to the Th1 lineage. Th1 cells produce cytokines which favor a cell-mediated response (IL-2, lymphotoxin, IFN-γ, tumor necrosis factor beta [TNFβ]), warding off intracellular pathogens, mounting delayed-type hypersensitivity responses to viral and bacterial antigens, and eliminating tumor cells [16].
Production of IL-4 and IL-10 drives commitment to the Th2 subtypes [24]. Th2 cells release cytokines which produce an environment favoring humoral immunity (IL-4, IL-5, IL-6, IL-10, and IL-13) by stimulating Th2-cell proliferation, differentiation, and participation in humoral immunity [16].
In the aged, however, naïve cells are less likely to become effectors; in those that do, there is a documented shift towards a Th2 cytokine response [16].
The molecular and cellular changes associated with aging have substantial clinical ramifications. The elderly have impaired ability to achieve immunization but much higher levels of circulating autoantibodies (due to the lack of naïve effectors), impaired response to viral infections, increased risk of bacterial infections, and increased risk of both neoplastic and autoimmune disease [8].
23.3 Gender and Immunity
Immunosenescence does not affect men and women equally [12]. The dysregulation in T-cell function, for example, that is associated with aging, occurs much more dramatically in women than in men [25,26].
Gender-specific differences in immunosenescence are at least partly attributable to sex hormones, evidenced by the fact that men and postmenopausal women have reduced T-cell immunity as compared to premenopausal women [27]. The fact that men live shorter lives, on average, than women is also partially attributed to the thymic involution produced by higher circulating levels of androgens in men [28], and a recent study has documented greater age-associated decline of less differentiated lymphocytes and increase of highly differentiated T cells in females [29].
Much is known about the influence of sex hormones on immunity in general. Androgens, estrogen, and progesterone all influence immune functions; estrogen in the form of 17β estradiol has been particularly associated with profound influences on the immune system (Table 23.2).
Table 23.2
Biological functions of estrogen
Immune function | Immune characteristic | Reference |
---|---|---|
B cell | Induction of antibody production | [38] |
Produces higher overall immunoglobulin levels | [31] | |
Promotes antibody response to foreign antigens | [31] | |
Increases IgG and IgM levels | [35] | |
Produces polyclonal induction of B cells in vitro | [136] | |
Cytokines and effector molecules | Stimulates proinflammatory serum markers | [137] |
Downregulates soluble cell adhesion molecules in women | [138] | |
Inhibits expression of monocyte chemoattractant protein-1 | [139] | |
Stimulates interleukin production (IL-1, IL-4, IL-6, IL-10 in macrophages; IL-4, IL-5,IL-6; and 1-0 in Th2 cells | [38] | |
Stimulates cellular response to cytokines | [31] | |
Stimulates secretion of IL-4, IFN-γ, and IL-1 | [31] | |
Stimulates production of anti-apoptosis protein Bcl-2 | [140] | |
Inhibits IL-1-induced expression of cell adhesion molecules | [139] | |
Inhibits of TNF-α expression | [141] | |
Downregulates soluble TNF-α | [142] | |
T cell | Downregulates number of immature T lymphocytes | [143] |
Systemic | Stimulates resistance to certain infections | [31] |
Downregulates induction of tolerance | [31] | |
Stimulates graft rejection | [31] | |
T cell | Stimulates involution of the thymus | [143] |
Stimulates production of regulatory T cells (Tregs) | [53] | |
Downregulates CD4 T cells | [31] | |
Stimulates an increase CD4/CD8 ratios | [31] |
Females, in general, have superior immune vigilance as compared to males, with both humoral and cell-mediated arms mounting more vigorous responses to immune stimulation [30]. Women maintain higher antibody levels than men, as well as higher levels of circulating IL-1, IL-4, and INF-γ [31]. Females reject grafts faster [32]. A general pattern is observed in which estrogen enhances humoral immunity, while androgens as well as progesterone tend to suppress it [33]. Women respond to antigenic stimulation with a predominantly Th2 response, with increased antibody production [34]. Estrogen stimulates the Th2 response by stimulating Th lymphocytes to secrete type 2 cytokines which promote the synthesis of antibodies [35]. High estrogen levels associated with pregnancy also produce a shift towards Th2 response [36].
Conversely, men respond to antigenic stimulation primarily with a Th1 response [34]. Androgens stimulate Th cells to produce type 1 cytokines, which suppress Th2 activity and stimulate CD8 cells [37], a process that produces inflammation as the predominant immune response [34].
The fact that estrogen favors a stronger overall immune response, particularly with regard to antibody response in women, is a mixed blessing. Although it produces a superior resistance to infection as compared to men, it also increases the risk in women of autoimmune disease [38].
23.4 Aging and the Development of Autoimmunity
There are at least 70 documented autoimmune diseases [38], and the prevalence of autoimmune disease is rapidly rising worldwide, for reasons not completely understood [39]. Although the disorders share a pathogenic immunity against the body’s own tissues that is the product of a progressive disorganization of immune function, the precise etiology is unknown. Autoimmunity appears to be a multifactorial process in which genetic, environmental, and biochemical processes all participate.
A genetic or familial predisposition to autoimmunity clearly plays a role. Pairwise analyses examined discordant familiar risks for seven common autoimmune diseases using a large national databank that included the records of 172,242 patients. Records examined demonstrated a genetic pattern of inheritance for RA, SLE, type I diabetes, ankylosing spondylitis, Crohn’s disease, celiac disease, and ulcerative colitis. Incidence of each of the seven autoimmune diseases analyzed was associated additionally with at least three of the others [40].
Environmental factors such as pollution or occupational exposures or contact with viral, bacterial, or parasitic pathogens may trigger autoimmunity. Lifestyle differences like nutritional choices, sleep patterns, medications, and stress may also trigger illness [38].
Endogenous factors also play a role; for example, sex hormones are a major influence [31]. The most striking gender-based difference in immune system function is the remarkable female predominance of autoimmune diseases [41]. An estimated 78 % of those affected by autoimmune disease are women [34].
Gender-specific patterns in the development of autoimmune diseases suggest a strong role of sex hormones, as predominance in the female sex changes with age at disease diagnosis, lending strong support [42]. Autoimmunity in males shows less age-dependent variation. Autoimmune diseases prevalent in males typically present before the sixth decade with the appearance of autoantibodies, acute inflammation, and increase in the proinflammatory cytokines characteristic of a Th1 response.
Those that manifest primarily in females are more complicated. Autoimmune diseases that manifest early in life in females generally have a clear antibody-mediated pathology. Those with increased incidence in females that appear after the age of 50 (in menopause) tend to be characterized by a more chronic disease course and fibrotic Th-mediated pathology.
A central role for sex hormones in autoimmune disease in women is also evidenced by the dramatic differences in prevalence during the different reproductive periods of a woman’s life, which are themselves driven by profound modulation of circulating levels of sex steroids, particularly estrogen [31]. Many autoimmune diseases in which women predominate are exacerbated by the higher levels of female sex steroids in pregnancy (a period that is also characterized by a shift towards Th2 response), primarily estrogen, which worsens disease, while androgens produce beneficial effect.
Strict correlation of autoimmunity with estrogen levels, however, is not observed. Other female-predominant autoimmune diseases, such as multiple sclerosis and rheumatoid arthritis, worsen during pregnancy [43] and improve in the postmenopausal period [44] (Table 23.3).
Table 23.3
Preponderance of females afflicted with autoimmune diseases
Autoimmune disease | Target of autoantibodies | Percent female | F/M differential | Peak of prevalence | Pathway | Effect of menopause |
---|---|---|---|---|---|---|
Hashimoto’s thyroiditis | Thyroid gland | 95 %a | 37:1b | 40s in women, 50s in men | Th2 | Associated with early menopause |
Sjögren’s syndrome | Mucous membranes and salivary glands | 94 %a | 14:1b | 30–40s | Th2 | Worsens |
SLE | DNA (primarily double stranded) | 89 %a | 11:1b | Late 20s, early 30s | Th2 | Improves |
Grave’s disease | Thyroid | 88 %a | 6:1b | Late 40s to early 60s | Th2 | Can precipitate premature menopause |
Scleroderma | Connective tissue | 92 %a | 3.5:1b | 40s | Th2 | Little affect |
Diabetes mellitus | Pancreas | 48 %a | 5:1b | 20s | Th1 | Worsens |
Rheumatoid arthritis | Synovial joints | 75 %a | 2.5:1b | 60s | Th1 | Worsens |
Multiple sclerosis | Central nervous system | 64 %a | Unknown | Late 20s | Th1 | Worsens |
Myasthenia gravis | Muscle tissue | 73 %a | 2.5:1b | Early 30s | Unknown | Unknown |
The influence on autoimmune disease of the decreasing levels of estrogen associated with aging in women is essentially unascertained. The declining efficacy of the immune system with age, in both genders, is accompanied by a characteristic increase in both the variety and level of circulating autoantibodies [8]. Antinuclear antibody (ANA) levels remain constant until about age 60 and then rise. About 5 % of healthy individuals of that age have high ANA titers (1:160), compared to 37 % of those over 70 years of age [45].
Although autoantibodies, found in the blood of all healthy humans, serve a useful function in a healthy adult, acting to clear away cellular debris produced by routine injury and inflammation [46], the number of different circulating autoantibodies has proven to be a good predictor of autoimmune disease. The risk of developing childhood diabetes within 5 years, for example, is only 10 % with the presence of one autoantibody specificity, but increases to as much as 80 % if there are three [34]. Autoantibody levels are normally kept in check by immune tolerance processes, but can (through age or overt disease) reach clinically significant levels, at which point the binding of self-antigens activates the complement cascade and results in cytotoxicity or other immune pathology [34]. Rising levels of antibodies, apart from the number of different specificities, are also associated with a generally increased risk of autoimmunity in old age [8].
What specifically causes the documented increase in both autoantibodies and autoimmunity in older adults is a matter of debate. With age, the normally tight orchestration of interdependent immune functions begins to decay, with progressive perturbation of immune function that can eventually lead to autoimmune disease [8]. Increased autoimmunity appears to be primarily the result of the combined effects of the reduction in naïve T cells (produced by thymus involution) in concert with an activation of self-reactive memory B cells.
B-cell activation may result from a variety of antigenic stimuli [47]. Exposure to an infectious agent with molecular mimicry of a self-antigen may prompt a memory response [16]. Cumulative exposure to a variety of antibody specificities, in the presence of chronic infections, for example, may also produce hyperstimulation of B cells. In a study of the elderly in Cameroon (where multiple chronic infections are not uncommon), the pattern of autoantibodies observed in the elderly subjects was markedly different from that in industrialized countries, suggesting a role for long-term multiple antigen exposures [48]. Normal aging can also reduce the efficiency of normal physical immune barriers, resulting in increased pathogen intrusion [49]. Innate immunity also deteriorates with age, which can contribute to chronic immune stimulation. The persistence of high antigen levels can also make co-stimulatory T cells less susceptible to downregulation [49].
Other sources of B-cell memory activation are neoantigens which are revealed by a progressive loss of tissue integrity and increased inflammation as individuals age [50]. Inappropriate activation of lymphocytes can also result from defective clearance of cellular debris, resulting in prolonged exposure to autoantigens [49]. Self-antigens may also acquire alterations that increase immunogenicity. For example, posttranslational modification of proteins increases in immunosenescence; particular modifications, such as isoaspartyl formation, can trigger an autoimmune response [49].
Improper self-antigen recognition by dendritic cells (DC) and T cells initiates, through release of specific cytokines (as described above), destructive Th1 or Th2 responses. Rheumatoid arthritis, for example, is characterized by an exaggerated Th1 self-reactive immunity, SLE by an excessive Th2 [24]. Estrogens are known to drive physiological selection of Th1 or Th2 pathways.
A perturbation in immune receptor signaling may underlie the increase in autoimmune phenomena in the elderly, particularly those that contribute to the regulation of immune tolerance. Optimal immune function necessitates a tight balance of the signaling pathways in both T- and B-cell compartments; these pathways are altered in both compartments in SLE and other autoimmune diseases [51].
Other aspects of aging can increase immune dysfunction as well: stress, with associated increased cortisol levels; sleep dysregulation and associated effects on immunity; decrease in physical activity and negative effects on immunocompetence; and nutritional deficiencies common in old age with a negative impact on immunocompetence [4].
23.5 Specific Effects of Estrogen on Autoimmunity
In women, the cumulative physiological degeneration associated with normal aging is augmented by a dramatic and systemic estrogen deprivation. Estrogens generally favor immune processes involving CD4+ Th2 cells and B cells [52], thereby promoting B-cell-mediated autoimmune diseases. Physiological levels of estrogen, however, also stimulate the expansion of CD4+ CD25+regulatory T cells (Treg cells), which help maintain tolerance to self-antigens and therefore ameliorate autoimmune disease, as well as the expression of the Foxp3 gene, a marker for Treg cell function [53]. Follicular helper T cells (Tfh cells), in contrast, appear to drive autoantibody production in the germinal center and are associated with the development of systemic autoimmunity [54].
Androgens, on the other hand, encourage processes involving CD4+Th1 cells and CD8+ cells, acting to suppress or ameliorate B-cell-mediated autoimmune diseases [31]. The influence of these hormones on the immune system, therefore, produces more autoimmune pathology in women in autoimmune disease mediated by Th2-dominant processes and more in men when Th1 processes are involved (see Table 23.3).
Hormonal manipulations can alter immune reactivity and modulate disease expression [41]. Estrogens provoke involution of the thymus [38]; treatment of castrated animals with exogenous sex hormones causes massive atrophy of the thymus [55]. Thymus regeneration occurs following ovariectomy [5], and thymus involution occurs during pregnancy, which reverts after lactation ceases [56].
Estrogen-induced involution of the thymus is associated with a reduction in numbers of immature T lymphocytes [38]. Estrogen affects T-cell subset composition, as well as T-cell function and activation [12]. Oral estrogen replacement therapy has been shown to restore T-cell function [57].
Estrogen drastically reduces not only the size of the thymus but also of the bone marrow cavity as well, the sites where most deletion of autoreactive cells occurs. B cells developing at alternative sites (liver and spleen), where less stringent selection occurs, may escape normal controls [31].
Estrogens, in fact, stimulate lymphopoiesis outside of the bone marrow [58]. Mice treated with exogenous estrogen develop impressive hemopoietic centers in the liver and spleens filled with antibody-producing cells [31].
Estrogen depletion in women, characteristic of postmenopausal women, has been specifically associated with reductions in B cells and T cells [59] and Th-derived cytokines [60] as well as an impaired immune response to viral infections [61]. In vitro, estrogen stimulates Th1 cytokine production by T cells [47].
Estrogens diminish the number of monocytes, through apoptosis, as well as through a modulation of the cell cycle which delays mitosis [62]. Autoreactive B-cell apoptosis, particularly, is inhibited [63]. Estrogen however simultaneously induces a rapid maturation of B lymphocytes [38] and stimulates Th lymphocytes to secrete type 2 cytokines that promote antibody production [35,37].
23.6 What Is Known About the Estrogen Deficiency of Menopause and Specific Autoimmune Diseases
It is known that many natural, pathological, and therapeutic conditions can change serum estrogen levels, including the menstrual cycle, pregnancy, menopause, use of oral contraceptives, use of hormonal replacement treatment, and disease. The estrogen withdrawal of menopause, however, is a significant hormonal transition and one with numerous physiological ramifications. The interrelationship of menopause and autoimmune diseases is complex and not yet fully understood. Recent literature has noted that autoimmune disease and/or autoimmune disease treatments can play a role in causing premature ovarian failure (POF) resulting in early menopause [64,65]. In addition, some symptoms and effects of autoimmune disease (e.g., increased cardiovascular risk, increased fracture risk, cognitive decline, urinary incontinence/irritable bladder, etc.) can be similar to those of normal aging, which increases the challenge of distinguishing the expected effects of menopause and aging from those of autoimmune disease [64,66]. These aspects of the relationship between menopause and autoimmune disease will not be discussed in this chapter, which shall rather concentrate on effects of menopause on selected autoimmune diseases.
The normal immunosenescence evidenced in both sexes may be modulated in some degree by changing levels and forms of endogenous estrogen. The production of cytokines Il-6, Il-1, and TNF-α increases after menopause, as does the physiological response to those cytokines [11]. There is a postmenopausal decrease in CD4 T and B lymphocytes, as well as decrease in the cytotoxic activity of natural killer (NK) cells [33].
In vitro analysis of three different T-cell signaling proteins (Janus kinase 2 [JAK2], Janus kinase 3 [JAK3], and CD3-zeta) found that postmenopausal levels were substantially reduced as compared to premenopausal levels [12]. In addition, Jurkat T cells exposed to premenopausal levels of E2 also formed significantly more IL-2 producing colonies as compared to those exposed to postmenopausal levels (75.3 ± 2.2 vs 55.7 ± 2.1 [P < 0.0001]) [12]. Studies suggest a normalization of cellular response after hormone replacement therapy (HRT) [11].
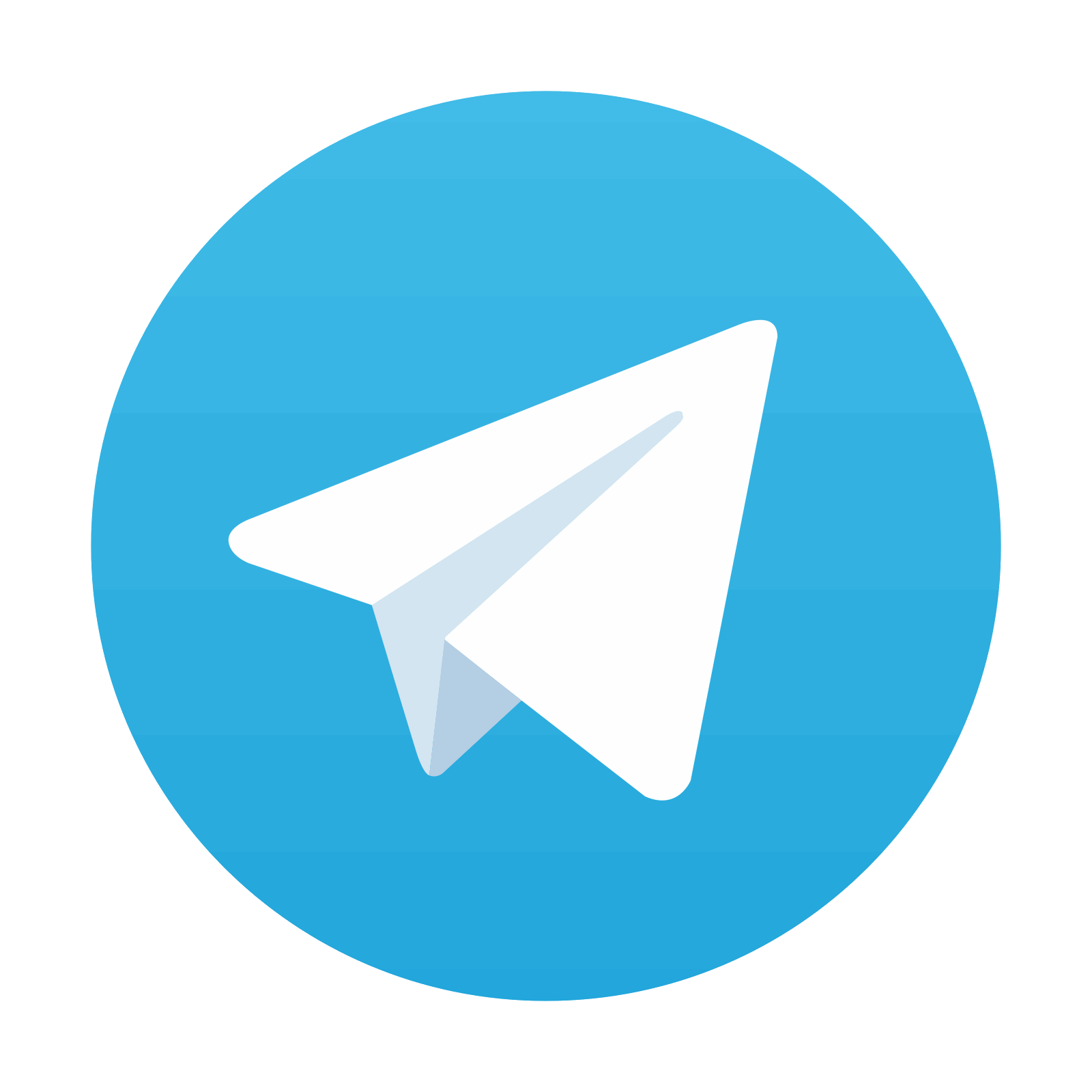
Stay updated, free articles. Join our Telegram channel

Full access? Get Clinical Tree
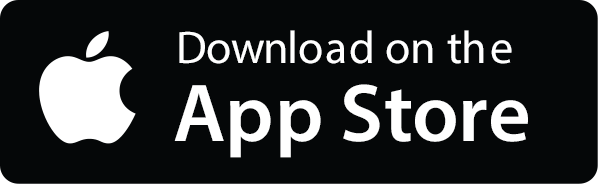
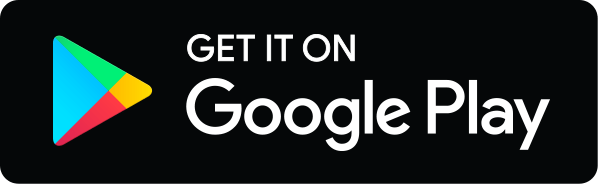